Research Dynamics of Tissue Spheroids as Building Blocks: A Scientometric Analysis
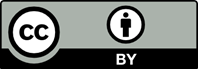
Tissue spheroids represent an innovative solution for tissue engineering and regenerative medicine. They constitute an in vitro three-dimensional cell culture model capable of mimicking the complex composition of a native tissue on a microscale; this model can function as a building block and be assembled into larger tissue constructs. Due to the potential tissue spheroids have for the evolution of the health industry, there is a need to assess the research dynamics of this field. Thus far, there have been no studies on their use as building blocks. To fill this gap, a study was performed to characterize the evolution of research where tissue spheroids were used as building blocks to generate tissue constructs. A scientometric analysis of the literature regarding tissue spheroid technologies was developed by quantification of bibliometric performance indicators. For this purpose, articles published during the period January 1, 2015 – December 31, 2021, from the Scopus database were organized and analyzed. The main subject areas, countries, cities, journals, institutions, and top-cited articles as well as the types of techniques, cells, culture time, and principal applications were identified. This research supports the definition and growth of research and development strategies for new technologies such as tissue spheroids.
1. Costa EC, de Melo‐Diogo D, Moreira AF, et al., 2018, Spheroids Formation on Non‐adhesive Surfaces by Liquid Overlay Technique: Considerations and Practical Approaches. Biotechnol J, 13:1700417. https://doi.org/10.1002/biot.201700417
2. Beachley V, Kasyanov V, Nagy-Mehesz A, et al., 2014. The Fusion of Tissue Spheroids Attached to Pre-stretched Electrospun Polyurethane Scaffolds. J Tissue Eng, 5:1-11. https://doi.org/10.1177/2041731414556561
3. Kelm JM, Lorber V, Snedeker JG, et al., 2010, A Novel Concept for Scaffold-free Vessel Tissue Engineering: Selfassembly of Microtissue Building Blocks. J Biotechnol, 148:46–55. https://doi.org/10.1016/j.jbiotec.2010.03.002
4. Ong CS, Nam L, Ong K, et al., 2018, 3D and 4D Bioprinting of the Myocardium: Current Approaches, Challenges, and Future Prospects. Biomed Res Int, 2018:6497242. https://doi.org/10.1155/2018/6497242
5. Hospodiuk M, Dey M, Sosnoski D, et al., 2017, The Bioink: A Comprehensive Review on Bioprintable Materials. Biotechnol Adv, 35:217–39. https://doi.org/10.1016/j.biotechadv.2016.12.006
6. Donderwinkel I, Hest JC, Cameron NR, 2017, Bio-inks for 3D Bioprinting: Recent Advances and Future Prospects. Polym Chem, 8:4451–71. https://doi.org/10.1039/c7py00826k
7. Groll J, Boland T, Blunk T, et al., 2016, Biofabrication: Reappraising the Definition of an Evolving Field. Biofabrication, 8:013001. https://doi.org/10.1088/1758-5090/8/1/013001
8. Guillemot F, Mironov V, Nakamura M, 2010, Bioprinting is Coming of Age: Report from the International Conference on Bioprinting and Biofabrication in Bordeaux (3B’09). Biofabrication, 2:010201. https://doi.org/10.1088/1758-5082/2/1/010201
9. Liaw CY, Guvendiren M, 2017, Current and Emerging Applications of 3D Printing in Medicine. Biofabrication, 9:024102. https://doi.org/10.1088/1758-5090/aa7279
10. Blakely AM, Manning KL, Tripathi A, et al., 2015, Bio-pick, Place, and Perfuse: A New Instrument for Three-dimensional Tissue Engineering. Tissue Eng Part C Methods, 21:737–46. https://doi.org/10.1089/ten.tec.2014.0439
11. Cui FR, Ip BC, Morgan JR, et al., 2018, Hydrodynamics of the Bio-Gripper: A Fluid-driven “Claw Machine” for Soft Microtissue Translocation. SLAS Technol, 23:540–9. https://doi.org/10.1177/2472630318775079
12. Ghosh S, Kumar S, Puri I, et al., 2016, Magnetic Assembly of 3D Cell Clusters: Visualizing the Formation of an Engineered Tissue. Cell Proliferation, 49:134–44. https://doi.org/10.1111/cpr.12234
13. Lin RZ, Chu WC, Chiang CC, et al., 2008, Magnetic Reconstruction of Three-dimensional Tissues from Multicellular Spheroids. Tissue Eng Part C Methods, 14:197–205. https://doi.org/10.1089/ten.tec.2008.0061
14. Christensen K, Xu C, Chai W, et al., 2015, Freeform Inkjet Printing of Cellular Structures with Bifurcations. Biotechnol Bioeng, 112:1047–55. https://doi.org/10.1002/bit.25501
15. Gutzweiler L, Kartmann S, Troendle K, et al., 2017. Large Scale Production and Controlled Deposition of Single HUVEC Spheroids for Bioprinting Applications. Biofabrication, 9:025027. https://doi.org/10.1088/1758-5090/aa7218
16. Rodríguez-Salvador M, Ruiz-Cantú L, 2019, Revealing Emerging Science and Technology Research for Dentistry Applications of 3D Bioprinting. Int J Bioprint, 5:170. https://doi.org/10.18063/ijb.v5i1.170
17. Zhou Y, Lin H, Liu Y, et al., 2019. A Novel Method to Identify Emerging Technologies using a Semi-supervised Topic Clustering Model: A Case of 3D Printing Industry. Scientometrics, 120:167–85. https://doi.org/10.1007/s11192-019-03126-8
18. Hess DJ, 1997, Science Studies. An Advanced Introduction. New York: University Press.
19. Elsevier, 2019, Scopus. Available from: http://www.elsevier. com/solutions/scopus [Last accessed on 2021 Nov 21].
20. Rodriguez-Salvador M, Perez-Benitez BE, Padilla-Aguirre KM, 2021, Discovering the Latest Scientific Pathways on Tissue Spheroids: Opportunities to Innovate. Int J Bioprint, 7:331. https://doi.org/10.18063/ijb.v7i1.331
21. Rodríguez-Salvador M, Rio-Belver RM, Garechana-Anacabe G, 2017, Scientometric and Patentometric Analyses to Determine the Knowledge Landscape in Innovative Technologies: The Case of 3D Bioprinting. PLoS One, 12:0180375. https://doi.org/10.1371/journal.pone.0180375
22. Lin BL, Chen JF, Qiu WH, et al., 2017, Allogeneic Bone Marrow-derived Mesenchymal Stromal Cells for Hepatitis B Virus-related Acute-on-chronic Liver Failure: A Randomized Controlled Trial. Hepatology (Baltimore, Md.), 66:209–19. https://doi.org/10.1002/hep.29189
23. Xu Y, Shi T, Xu A, et al., 2016, 3D Spheroid Culture Enhances Survival and Therapeutic Capacities of MSCs Injected into Ischemic Kidney. J Cell Mol Med, 20:1203–13. https://doi.org/10.1111/jcmm.12651
24. Avolio E, Meloni M, Spencer HL, et al., 2015, Combined Intramyocardial Delivery of Human Pericytes and Cardiac Stem Cells Additively Improves the Healing of Mouse Infarcted Hearts Through Stimulation of Vascular and Muscular Repair. Circulation Res, 116:e81–94. https://doi.org/10.1161/circresaha.115.306146
25. Reid JA, Mollica PA, Johnson GD, et al., 2016, Accessible Bioprinting: Adaptation of a Low-cost 3D-printer for Precise Cell Placement and Stem Cell Differentiation. Biofabrication, 8:025017. https://doi.org/10.1088/1758-5090/8/2/025017
26. Knowlton S, Yenilmez B, Tasoglu S, 2016, Towards Single-Step Biofabrication of Organs on a Chip via 3D Printing. Trends Biotechnol, 34:685–8. https://doi.org/10.1016/j.tibtech.2016.06.005
27. Zhao H, Chen Y, Shao L, et al., 2018, Airflow-assisted 3D Bioprinting of Human Heterogeneous Microspheroidal Organoids with Microfluidic Nozzle. Small, 14:1802630. https://doi.org/10.1002/smll.201802630
28. Harley WS, Li CC, Toombs J, et al., 2021, Advances in Biofabrication Techniques Towards Functional Bioprinted Heterogeneous Engineered Tissues: A Comprehensive Review. Bioprinting, 23:e00147. https://doi.org/10.1016/j.bprint.2021.e00147.
29. UNESCO, 2019a, How much Does Your Country Invest in R&D? Available from: http://www.uis.unesco.org/apps/visualisations/research-and-development-spending [Last accessed on 2022 Jan 28].
30. UNESCO, 2019b, Purchasing Power Parity (PPP). Available form: http://www.uis.unesco.org/en/glossary-term/purchasingpower-parity-ppp [Last accessed on 2022 Jan 28]. https://doi.org/10.1007/springerreference_2272
31. Nagamoto Y, Takayama K, Ohashi K, et al., 2016, Transplantation of a Human iPSC-derived Hepatocyte Sheet Increases Survival in Mice with Acute Liver Failure. J Hepatol, 64:1068–75. https://doi.org/10.1016/j.jhep.2016.01.004
32. Jha AK, Tharp KM, Ye J, et al., 2015, Enhanced Survival and Engraftment of Transplanted Stem Cells using Growth Factor Sequestering Hydrogels. Biomaterials, 47:1–12. https://doi.org/10.1016/j.biomaterials.2014.12.043
33. Pendergraft SS, Sadri-Ardekani H, Atala A, et al., 2017, Three-dimensional Testicular Organoid: a Novel Tool for the Study of Human Spermatogenesis and Gonadotoxicity in vitro. Biol Reprod, 96:720–32. https://doi.org/10.1095/biolreprod.116.143446
34. Zhang L, Chen K, Zhang H, et al., 2018, Microfluidic Templated Multicompartment Microgels for 3D Encapsulation and Pairing of Single Cells. Small (Weinheim an der Bergstrasse, Germany), 14:2955. https://doi.org/10.1002/smll.201702955
35. Saheli, M, Sepantafar, M, Pournasr, B, et al., 2018, Three dimensional Liver‐derived Extracellular Matrix Hydrogel Promotes Liver Organoids Function. J Cell Biochem, 119:4320–33. https://doi.org/10.1002/jcb.26622
36. Siltanen C, Diakatou M, Lowen J, et al., 2017, One Step Fabrication of Hydrogel Microcapsules with Hollow Core for Assembly and Cultivation of Hepatocyte Spheroids. Acta Biomater, 50:428–36. https://doi.org/10.1016/j.actbio.2017.01.010
37. Zuellig RA, Cavallari G, Gerber P, et al., 2017, Improved Physiological Properties of Gravity-enforced Reassembled Rat and Human Pancreatic Pseudo-islets. J Tissue Eng Regen Med, 11:109–20. https://doi.org/10.1002/term.1891
38. Alliance for Regenerative Medicine, 2021, ARM Press Release: Global Cell and Gene Therapy Sector on Pace for Annual Records in Product Approvals and Financings, Says Alliance for Regenerative Medicine. Alliance for Regenerative Medicine. Available from: https://www.alliancerm.org/pressrelease/global-cell-gene-therapy-sector-on-pace-for-annualrecords-in-product-approvals-and-financings-says-alliancefor-regenerative-medicine [Last accessed on 2022 Feb 04]. https://doi.org/10.2217/rme-2020-1512s
39. Moldovan NI, Hibino N, Nakayama K, 2017, Principles of the Kenzan Method for Robotic Cell Spheroid-based Three dimensional Bioprinting. Tissue Eng Part B Rev, 23:237–44. https://doi.org/10.1089/ten.teb.2016.0322
40. 3D Bioprinting Solutions, 2014, Creating Future Possibilities. Available from: https://www.bioprinting.ru/en/productsservices/fabion [Last accessed on 2021 Nov 25].
41. Ng SS, Saeb-Parsy K, Blackford S, et al., 2018, Human iPS Derived Progenitors Bioengineered into Liver Organoids using an Inverted Colloidal Crystal Poly (Ethylene Glycol) Scaffold. Biomaterials, 182:299–311. https://doi.org/10.1016/j.biomaterials.2018.07.043
42. Akkouch A, Yu Y, Ozbolat IT, 2015, Microfabrication of Scaffold-free Tissue Strands for Three-dimensional Tissue Engineering. Biofabrication, 7:031002. https://doi.org/10.1088/1758-5090/7/3/031002
43. Phamduy TB, Sweat RS, Azimi MS, et al., 2015, Printing Cancer Cells into Intact Microvascular Networks: A Model for Investigating Cancer Cell Dynamics during Angiogenesis. Integrat Biol, 7:1068–78. https://doi.org/10.1039/c5ib00151j
44. Yamashita T, Takayama K, Sakurai F, et al., 2018, Billion scale Production of Hepatocyte-like Cells from Human Induced Pluripotent Stem Cells. Biochem Biophys Res Commun, 496:1269–75. https://doi.org/10.1016/j.bbrc.2018.01.186
45. Ye W, Li H, Yu K, et al., 2020, 3D Printing of Gelatin Methacrylate-based Nerve Guidance Conduits with Multiple Channels. Mater Design, 192:108757. https://doi.org/10.1016/j.matdes.2020.108757
46. Wuchter P, Saffrich R, Giselbrecht S, et al., 2016, Microcavity Arrays as an in vitro Model System of the Bone Marrow Niche for Hematopoietic Stem Cells. Cell Tissue Res, 364:573–84. https://doi.org/10.1007/s00441-015-2348-8
47. Herzog N, Hansen M, Miethbauer S, et al., 2016, Primary like Human Hepatocytes Genetically Engineered to Obtain Proliferation Competence Display Hepatic Differentiation Characteristics in Monolayer and Organo typical Spheroid Cultures. Cell Biol Int, 40:341–53. https://doi.org/10.1002/cbin.10574
48. Makkar RR, Smith RR, Cheng K, et al., 2012, Intracoronary Cardiosphere-derived Cells for Heart Regeneration after Myocardial Infarction (CADUCEUS): A Prospective, Randomised Phase 1 Trial. Lancet, 379:895–904. https://doi.org/10.1016/s0140-6736(12)60195-0
49. Labusca L, Herea DD, Minuti AE, et al., 2021, Magnetic Nanoparticle Loaded Human Adipose Derived Mesenchymal Cells Spheroids in Levitated Culture. J Biomed Mater Res Part B Appl Biomater, 109:630–42. https://doi.org/10.1002/jbm.b.34727