Bioprinting of a Hepatic Tissue Model Using HumanInduced Pluripotent Stem Cell-derived Hepatocytes for Drug-Induced Hepatotoxicity Evaluation
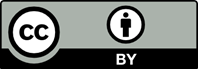
Three-dimensional (3D) bioprinting technology is an effective method for exploring the biological functions of hepatocytes by building biomimetic 3D microenvironments. Various hepatic tissue models have been developed for disease modeling, drug screening, and tissue regeneration using 3D bioprinting technology. Human-induced pluripotent stem cells (hiPSCs) are a promising cell source for the generation of functional hepatocytes for bioprinting. In this study, we introduced hiPSC-derived hepatocytes (hiPSC-Heps) as mature hepatocytes for the bioprinting of a 3D hepatic tissue model. The 3D-printed (3DP) model facilitated the formation of hiPSC-Hep spheroids with higher viability and proliferation than the commonly used non-printed sandwich-cultured model. hiPSC-Heps in the 3DP model exhibited higher mRNA expression of liver-specific functions than those in the two-dimensional-cultured model. Moreover, enhanced secretion of liver functionrelated proteins, including α-1-antitrypsin, albumin, and blood urea nitrogen, was observed in the 3DP model. For the evaluation of acetaminophen-induced hepatotoxicity, the 3DP model exhibited a favorable drug response with upregulation of the drug metabolism-related gene cytochrome P450-1A2 (CYP1A2). Overall, the bioprinted hepatic tissue model showed great biofunctional and drug-responsive performance, which could be potentially applied in in vitro toxicological studies.
1. Agarwal T, Banerjee D, Konwarh R, et al., 2021, Recent Advances in Bioprinting Technologies for Engineering Hepatic Tissue. Mater Sci Eng C Mater Biol Appl, 123:112005. https://doi.org/10.1016/j.msec.2021.112013
2. Hwang DG, Choi YM, Jang J, 2021, 3D Bioprinting-Based Vascularized Tissue Models Mimicking Tissue-Specific Architecture and Pathophysiology for In Vitro Studies. Front Bioeng Biotechnol, 9:685507. https://doi.org/10.3389/fbioe.2021.685507
3. Ma L, Wu YT, Li YT, et al., 2020, Current Advances on 3D-Bioprinted Liver Tissue Models. Adv HealthcMater, 9:2001517. https://doi.org/10.1002/adhm.202001517
4. Gough A, Soto-Gutierrez A, Vernetti L, et al., 2021, Human Biomimetic Liver Microphysiology Systems in Drug Development and Precision Medicine. Nat Rev Gastroenterol Hepatol, 18:252–68. https://doi.org/10.1038/s41575-020-00386-1
5. Hiller T, Berg J, Elomaa L, et al., 2018, Generation of a 3D Liver Model Comprising Human Extracellular Matrix in an Alginate/Gelatin-Based Bioink by Extrusion Bioprinting for Infection and Transduction Studies. Int J Mol Sci, 19:3129. https://doi.org/10.3390/ijms19103129
6. Huang DT, Gibeley SB, Xu C, et al., 2020, Engineering Liver Microtissues for Disease Modeling and Regenerative Medicine. Adv Funct Mater, 30:9553. https://doi.org/10.1002/adfm.201909553
7. Nguyen DG, Funk J, Robbins JB, et al., 2016, Bioprinted 3D Primary Liver Tissues Allow Assessment of Organ-Level Response to Clinical Drug Induced Toxicity In Vitro. PLoS One, 11:5867. https://doi.org/10.1371/journal.pone.0158674
8. Gori M, Giannitelli SM, Torre M, et al., 2020, Biofabrication of hepatic constructs by 3D bioprinting of a cell-laden thermogel: An effective tool to assess drug-induced hepatotoxic response. Adv Healthc Mater, 9:1163. https://doi.org/10.1002/adhm.202001163
9. Schmidt K, Berg J, Roehrs V, et al., 2020, 3D-bioprinted HepaRG Cultures as a Model for Testing Long Term Aflatoxin B1 Toxicity In Vitro. Toxicol Rep, 7:1578–87. https://doi.org/10.1016/j.toxrep.2020.11.003
10. Lu SM, Zhang JW, Lin S, et al., 2021, Recent Advances in the Development of In Vitro Liver Models for Hepatotoxicity Testing. Bio Des Manuf, 4:717–34. https://doi.org/10.1007/s42242-021-00142-7
11. Kryou C, Leva V, Chatzipetrou M, et al., 2019, Bioprinting for Liver Transplantation. Bioeng Basel, 6:95. https://doi.org/10.3390/bioengineering6040095
12. Yang HY, Sun LJ, Pang Y, et al., 2021, Three-dimensional Bioprinted Hepatorganoids Prolong Survival of Mice with Liver Failure. Gut, 70:567–74. https://doi.org/10.1136/gutjnl-2019-319960
13. Xie FH, Sun LJ, Pang Y, et al., 2021, Three-dimensional Bioprinting of Primary Human Hepatocellular Carcinoma for Personalized Medicine. Biomaterials, 265:120416. https://doi.org/10.1016/j.biomaterials.2020.120416
14. Faulkner-Jones A, Fyfe C, Cornelissen DJ, et al., 2015, Bioprinting of Human Pluripotent Stem Cells and their Directed Differentiation into Hepatocyte-like Cells for the Generation of Mini-livers in 3D. Biofabrication, 7:044102. https://doi.org/10.1088/1758-5090/7/4/044102
15. Ma X Y, Qu X, Zhu W, et al., 2016, Deterministically Patterned Biomimetic Human iPSC-derived Hepatic Model Via Rapid 3D Bioprinting. Proc Natl Acad Sci U S A, 113:2206–11. https://doi.org/10.1073/pnas.1524510113
16. Goulart E, de Caires LC, Telles-Silva KA, et al., 2020, 3D Bioprinting of Liver Spheroids Derived from Human Induced Pluripotent Stem Cells Sustain Liver Function and Viability In Vitro. Biofabrication, 12:30. https://doi.org/10.1088/1758-5090/ab4a30
17. Jeon H, Kang K, Park SA, et al., 2017, Generation of Multilayered 3D Structures of HepG2 Cells Using a Bioprinting Technique. Gut Liver, 11:121–8. https://doi.org/10.5009/gnl160https://doi.org/10
18. Sun LJ, Yang HY, Wang YN, et al., 2020, Application of a 3D Bioprinted Hepatocellular Carcinoma Cell Model in Antitumor Drug Research. Front Oncol, 10:878. https://doi.org/10.3389/fonc.2020.00878
19. Kang HK, Sarsenova M, Kim DH, et al., 2021, Establishing a 3D In Vitro Hepatic Model Mimicking Physiologically Relevant to In Vivo State. Cells, 10:1268. https://doi.org/10.3390/cells10051268
20. Lewis PL, Green RM, Shah RN, 2018, 3D-printed Gelatin Scaffolds of Differing Pore Geometry Modulate Hepatocyte Function and Gene Expression. Acta Biomater, 69:63–70. https://doi.org/10.1016/j.actbio.2017.12.042
21. Hannoun Z, Steichen C, Dianat N, et al., 2016, The Potential of Induced Pluripotent Stem Cell Derived Hepatocytes. J Hepatol, 65:182–99. https://doi.org/10.1016/j.jhep.2016.02.025
22. Chen YF, Tseng CY, Wang HW, et al., 2012, Rapid Generation of Mature Hepatocyte-like Cells from Human Induced Pluripotent Stem Cells by an Efficient Three-step Protocol. Hepatology, 55:1193–203. https://doi.org/10.1002/hep.24790
23. Yi F, Liu GH, Belmonte JC, 2012, Human Induced Pluripotent Stem Cells Derived Hepatocytes: Rising Promise for Disease Modeling, Drug Development and Cell Therapy. Protein Cell, 3:246–50. https://doi.org/10.1007/s13238-012-2918-4.
24. Tabar V, Studer L, 2014, Pluripotent Stem Cells in Regenerative Medicine: Challenges and Recent Progress. Nat Rev Genet, 15:82–92. https://doi.org/10.1038/nrg3563
25. Luce E, Messina A, Duclos-Vallee JC, et al., 2021, Advanced Techniques and Awaited Clinical Applications for Human Pluripotent Stem Cell Differentiation into Hepatocytes. Hepatology, 74:1101–16. https://doi.org/10.1002/hep.31705
26. Chen ST, Wang JL, Ren HZ, et al., 2020, Hepatic Spheroids Derived from Human Induced Pluripotent Stem Cells in Bioartificial Liver Rescue Porcine Acute Liver Failure. Cell Res, 30:1-3. https://doi.org/10.1038/s41422-019-0261-5
27. Wang J, Ren H, Liu Y, et al., 2021, Bioinspired Artificial Liver System with hiPSC-Derived Hepatocytes for Acute Liver Failure Treatment. Adv Healthc Mater, 10:e2101580. https://doi.org/10.1002/adhm.202101580
28. Lin RZ, Chang HY, 2008, Recent Advances in Three dimensional Multicellular Spheroid Culture for Biomedical Research. Biotechnol J, 3:1172–84. https://doi.org/10.1002/biot.200700228
29. Decarli MC, Amaral R, Dos Santos DP, et al., 2021, Cell Spheroids as a Versatile Research Platform: Formation Mechanisms, High Throughput Production, Characterization and Applications. Biofabrication, 13:37. https://doi.org/10.1088/1758-5090/abe6f2
30. Mandon M, Huet S, Dubreil E, et al., 2019, Three dimensional HepaRG Spheroids as a Liver Model to Study Human Genotoxicity In Vitro with the Single Cell Gel Electrophoresis Assay. Sci Rep, 9:1058. https://doi.org/10.1038/s41598-019-47114-7
31. Hurrell T, Ellero AA, Masso ZF, et al., 2018, Characterization and Reproducibility of HepG2 Hanging Drop Spheroids Toxicology In Vitro. Toxicol In Vitro, 50:86–94. https://doi.org/10.1016/j.tiv.2018.02.013
32. Lee G, Lee J, Oh H, et al., 2016, Reproducible Construction of Surface Tension-Mediated Honeycomb Concave Microwell Arrays for Engineering of 3D Microtissues with Minimal Cell Loss. PLoS One, 11:1026. https://doi.org/10.1371/journal.pone.0161026
33. Zhao YS, Xu YX, Zhang BF, et al., 2010, In Vivo Generation of Thick, Vascularized Hepatic Tissue from Collagen Hydrogel-Based Hepatic Units. Tissue Eng Part C Methods, 16:653–9. https://doi.org/10.1089/ten.tec.2009.0053
34. Zuchowska A, Kwapiszewska K, Chudy M, et al., 2017, Studies of Anticancer Drug Cytotoxicity Based on Long term HepG2 Spheroid Culture in a Microfluidic System. Electrophoresis, 38:1206–16. https://doi.org/10.1002/elps.201600417
35. Ehrlich A, Duche D, Ouedraogo G, et al., 2019, Challenges and Opportunities in the Design of Liver-on-Chip Microdevices. Ann Rev Biomed Eng, 21:219–39. https://doi.org/10.1146/annurev-bioeng-060418-052305
36. Ma XY, Liu J, Zhu W, et al., 2018, 3D Bioprinting of Functional Tissue Models for Personalized Drug Screening and In Vitro Disease Modeling. Adv Drug Deliv Rev, 132:235–51. https://doi.org/10.1016/j.addr.2018.06.011
37. Li XD, Wang X, Wang XZ, et al., 2018, 3D Bioprinted rat Schwann Cell-laden Structures with Shape Flexibility and Enhanced Nerve Growth Factor Expression. 3 Biotech, 8:342. https://doi.org/10.1007/s13205-018-1341-9
38. Liu PC, Shen HZ, Zhi Y, et al., 2019, 3D Bioprinting and In Vitro Study of Bilayered Membranous Construct with Human Cells-laden Alginate/Gelatin Composite Hydrogels. Colloids Surfaces B Biointerfaces, 181:1026–34. https://doi.org/10.1016/j.colsurfb.2019.06.069
39. Yu HY, Zhang XY, Song WJ, et al., 2019, Effects of 3-dimensional Bioprinting Alginate/Gelatin Hydrogel Scaffold Extract on Proliferation and Differentiation of Human Dental Pulp Stem Cells. J Endod, 45:706–15. https://doi.org/10.1016/j.joen.2019.03.004
40. Khoshnood N, Zamanian A, Abbasi M, 2021, The Potential Impact of Polyethylenimine on Biological Behavior of 3D-printed Alginate Scaffolds. Int J Biol Macromol, 178:19–28. https://doi.org/10.1016/j.ijbiomac.2021.02.152
41. Cho CH, Park J, Nagrath D, et al., 2007, Oxygen Uptake Rates and Liver-specific Functions of Hepatocyte and 3T3 Fibroblast Co-cultures. Biotechnol Bioeng, 97:188–99. https://doi.org/10.1002/bit.21225
42. Demol J, Lambrechts D, Geris L, et al., 2011, Towards a Quantitative Understanding of Oxygen Tension and Cell Density Evolution in Fibrin Hydrogels. Biomaterials, 32:107–18. https://doi.org/10.1016/j.biomaterials.2010.08.093
43. Pang Y, Mao SS, Yao R, et al., 2018, TGF-beta Induced Epithelial-mesenchymal Transition in an Advanced Cervical Tumor Model by 3D Printing. Biofabrication, 10:044102. https://doi.org/10.1088/1758-5090/aadbde
44. Mao SS, He JY, Zhao Y, et al., 2020, Bioprinting of Patient derived In Vitro Intrahepatic Cholangiocarcinoma Tumor Model: Establishment, Evaluation and Anti-cancer Drug Testing. Biofabrication, 12:045014. https://doi.org/10.1088/1758-5090/aba0c3
45. Brophy CM, Luebke-Wheeler JL, Amiot BP, et al., 2009, Rat Hepatocyte Spheroids Formed by Rocked Technique Maintain Differentiated Hepatocyte Gene Expression and Function. Hepatology, 49:578–86. https://doi.org/10.1002/hep.22674
46. Ni HM, Bockus A, Boggess N, et al., 2012, Activation of Autophagy Protects against Acetaminophen-induced Hepatotoxicity. Hepatology, 55:222–31. https://doi.org/10.1002/hep.24690
47. Rodrigues RM, Heymans A, De Boe V, et al., 2016, Toxicogenomics-based Prediction of Acetaminophen induced Liver Injury Using Human Hepatic Cell Systems. Toxicol Lett, 240:50–9. https://doi.org/10.1016/j.toxlet.2015.10.014
48. Gaskell H, Sharma P, Colley HE, et al., 2016, Characterization of a Functional C3A Liver Spheroid Model. Toxicol Res, 5:1053–65. https://doi.org/10.1039/c6tx00101g
49. Takayama K, Kawabata K, Nagamoto Y, et al., 2013, 3D Spheroid Culture of hESC/hiPSC-derived Hepatocyte-like Cells for Drug Toxicity Testing. Biomaterials, 34:1781–9. https://doi.org/10.1016/j.biomaterials.2012.11.029
50. Tasnim F, Toh YC, Qu YH, et al., 2016, Functionally Enhanced Human Stem Cell Derived Hepatocytes in Galactosylated Cellulosic Sponges for Hepatotoxicity Testing. Mol Pharm, 13:1947–57. https://doi.org/10.1021/acs.molpharmaceut.6b00119
51. Gu JY, Shi XL, Zhang Y, et al., 2009, Study on the Effects and Mechanisms of Bone Marrow Mesenchymal Stem Cells on Porcine Primary Hepatocyte Culture In Vitro. Zhonghua Gan Zang Bing Za Zhi, 17:867–71.
52. He JY, Pang Y, Yang HY, et al., 2020, Modular Assembly based Approach of Loosely Packing Co-cultured Hepatic Tissue Elements with Endothelialization for Liver Tissue Engineering. Ann Transl Med, 8:100. https://doi.org/10.21037/atm-20-1598
53. Salerno S, Campana C, Morelli S, et al., 2011, Human Hepatocytes and Endothelial Cells in Organotypic Membrane Systems. Biomaterials, 32:8848–59. https://doi.org/10.1016/j.biomaterials.2011.08.004
54. Thomas RJ, Bhandari R, Barrett DA, et al., 2005, The Effect of Three-dimensional Co-culture of Hepatocytes and Hepatic Stellate Cells on Key Hepatocyte Functions In Vitro. Cells Tissues Organs, 181:67–79. https://doi.org/10.1159/000091096
55. Zinchenko YS, Schrum LW, Clemens M, et al., 2006, Hepatocyte and Kupffer Cells Co-cultured on Micropatterned Surfaces to Optimize Hepatocyte Function. Tissue Eng, 12:751–61. https://doi.org/10.1089/ten.2006.12.751