3D Printing of Diatomite Incorporated Composite Scaffolds for Skin Repair of Deep Burn Wounds
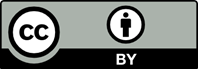
Deep burn injury always causes severe damage of vascular network and collagen matrix followed by delayed wound healing process. In this study, natural diatomite (DE) microparticles with porous nanostructure were separated based on the particles size through a dry sieving method and combined with gelatin methacryloyl (GelMA) hydrogel to form a bioactive composite ink. The DE-containing inorganic/organic composite scaffolds, which were successfully prepared through threedimensional (3D) printing technology, were used as functional burn wound dressings. The scaffolds incorporated with DE are of great benefit to several cellular activities, including cell spreading, proliferation, and angiogenesis-related gene expression in vitro, which can mainly be attributed to the positive effect of bioactive silicon (Si) ions released from the embedded DE. Moreover, due to establishment of bioactive ionic environment, the deep burn wounds treated with 3D-printed DE incorporated scaffolds exhibited rapid wound healing rate, enhanced collagen deposition, and dense blood vessel formation in vivo. Therefore, the present study demonstrates that the cost-effective DE can be used as biocompatible Si source to significantly promote the bioactivities of wound dressings for effective tissue regeneration.
1. Gurtner GC, Werner S, Barrandon Y, et al., 2008, Wound Repair and Regeneration. Nature, 453:314–21. https://doi.org/1038/nature07039
2. Tobin DJ, 2006, Biochemistry of Human Skin Our Brain on the Outside. Chem Soc Rev, 35:52–67. https://doi.org/1039/B505793K
3. Jahromi MA, Zangabad PS, Basri SM, et al., 2018, Nanomedicine and Advanced Technologies for Burns: Preventing Infection and Facilitating Wound Healing. Adv Drug Deliv Rev, 123:33–64. https://doi.org/1016/j.addr.2017.08.001
4. Rowan MP, Cancio LC, Elster EA, et al., 2015, Burn Wound Healing and Treatment: Review and Advancements. Crit Care, 19:243. https://doi.org/1186/s13054-015-0961-2
5. Ma PX, 2008, Biomimetic Materials for Tissue Engineering. Adv Drug Deliv Rev, 60:184–98. https://doi.org/1016/j.addr.2007.08.041
6. Chung JJ, Im HJ, Kim SH, et al., 2020, Toward Biomimetic Scaffolds for Tissue Engineering: 3D Printing Techniques in Regenerative Medicine. Front Bioeng Biotechnol, 8:586406. https://doi.org/3389/fbioe.2020.586406
7. Do AV, Khorsand B, Geary SM, et al., 2015, 3D Printing of Scaffolds for Tissue Regeneration Applications. Adv Healthc Mater, 4:1742–62. https://doi.org/1002/adhm.201500168
8. Rouwkema J, Rivron NC, van Blitterswijk CA, 2008, Vascularization in Tissue Engineering. Trends Biotechnol, 26:434–41. https://doi.org/1016/j.tibtech.2008.04.009
9. O’Brien FJ, 2011, Biomaterials and Scaffolds for Tissue Engineering. Mater Today, 14:88–95. https://doi.org/1016/S1369-7021(11)70058-X
10. Chouhan D, Dey N, Bhardwaj N, et al., 2019, Emerging and Innovative Approaches for Wound Healing and Skin Regeneration: Current Status and Advances. Biomaterials, 216:119267. https://doi.org/1016/j.biomaterials.2019.119267
11. Kankala RK, Han YH, Xia HY, et al., 2022, Nanoarchitectured Prototypes of Mesoporous Silica Nanoparticles for Innovative Biomedical Applications. J Nanobiotechnol, 20:126. https://doi.org/1186/s12951-022-01315-x
12. Zhu H, Zheng K, Boccaccini AR, 2021, Multi-functional Silica-based Mesoporous Materials for Simultaneous Delivery of Biologically Active Ions and Therapeutic Biomolecules. Acta Biomater, 129:1–17. https://doi.org/1016/j.actbio.2021.05.007
13. Han YH, Liu CG, Chen BQ, et al., 2022, Orchestrated Tumor Apoptosis (Cu2+) and Bone Tissue Calcification (Ca2+) by Hierarchical Copper/Calcium-ensembled Bioactive Silica for Osteosarcoma Therapy. Chem Eng J, 435:134820. https://doi.org/1016/j.cej.2022.134820
14. Lv F, Wang J, Xu P, et al., 2017, A Conducive Bioceramic/Polymer Composite Biomaterial for Diabetic Wound Healing. Acta Biomater, 60:128–43. https://doi.org/1016/j.actbio.2017.07.020
15. Xu H, Lv F, Zhang Y, et al., 2015, Hierarchically Micropatterned Nanofibrous Scaffolds with a Nanosized Bioglass Surface for Accelerating Wound Healing. Nanoscale, 7:18446–452. https://doi.org/1039/C5NR04802H
16. Valtchev V, Tosheva L, 2013, Porous Nanosized Particles: Preparation, Properties, and Applications. Chem Rev, 113:6734–60. https://doi.org/1021/cr300439k
17. Yang P, Gai S, Lin J, 2012, Functionalized Mesoporous Silica Materials for Controlled Drug Delivery. Chem Soc Rev, 41:3679–98. https://doi.org/1039/C2CS15308D
18. Erdem E, Çölgeçen G, Donat R, 2005, The Removal of Textile Dyes by Diatomite Earth. J Colloid Interface Sci, 282:314–9. https://doi.org/1016/j.jcis.2004.08.166
19. Khraisheh MA, Al-degs YS, McMinn WA, 2004, Remediation of Wastewater Containing Heavy Metals Using Raw and Modified Diatomite. Chem Eng J, 99:177–84. https://doi.org/1016/j.cej.2003.11.029
20. Tamburaci S, Tihminlioglu F, 2018, Biosilica Incorporated 3D Porous Scaffolds for Bone Tissue Engineering Applications. Mater Sci Eng C Mater Biol Appl, 91:274–91. https://doi.org/1016/j.msec.2018.05.040
21. Tamburaci S, Tihminlioglu F, 2017, Diatomite Reinforced Chitosan Composite Membrane as Potential Scaffold for Guided Bone Regeneration. Mater Sci Eng: C, 80:222–31. https://doi.org/1016/j.msec.2017.05.069
22. Le TD, Liaudanskaya V, Bonani W, et al., 2018, Enhancing Bioactive Properties of Silk Fibroin with Diatom Particles for Bone Tissue Engineering Applications. J Tissue Eng Regen Med, 12:89–97. https://doi.org/1002/term.2373
23. Maher S, Alsawat M, Kumeria T, et al., 2015, Luminescent Silicon Diatom Replicas: Self-Reporting and Degradable Drug Carriers with Biologically Derived Shape for Sustained Delivery of Therapeutics. Adv Funct Mater, 25:5107–16. https://doi.org/1002/adfm.201501249
24. Lv J, Sun B, Jin J, et al., 2019, Mechanical and Slow-released Property of Poly (Acrylamide) Hydrogel Reinforced by Diatomite. Mater Sci Eng C, 99:315–21. https://doi.org/1016/j.msec.2019.01.109
25. Feng C, Li J, Wu GS, et al., 2016, Chitosan-Coated Diatom Silica as Hemostatic Agent for Hemorrhage Control. ACS Appl Mater Interfaces, 8:34234–43. https://doi.org/1021/acsami.6b12317
26. Le TD, Bonani W, Speranza G, et al., 2016, Processing and Characterization of Diatom Nanoparticles and Microparticles as Potential Source of Silicon for Bone Tissue Engineering. Mater Sci Eng C, 59:471–9. https://doi.org/1016/j.msec.2015. https://doi.org/040
27. Zhang YX, Huang M, Li F, et al., 2014, One-pot Synthesis of Hierarchical MnO2-modified Diatomites for Electrochemical Capacitor Electrodes. J Power Sources, 246:449–56. https://doi.org/1016/j.jpowsour.2013.07.115
28. Gencel O, del Coz Diaz JJ, Sutcu M, et al., 2016, A Novel Lightweight Gypsum Composite with Diatomite and Polypropylene Fibers. Constr Build Mater, 113:732–40. https://doi.org/1016/j.conbuildmat.2016.03.125
29. Ying GL, Jiang N, Maharjan S, et al., 2018, Aqueous Two-Phase Emulsion Bioink-Enabled 3D Bioprinting of Porous Hydrogels. Adv Mater, 30:1805460. https://doi.org/1002/adma.201805460
30. Schuurman W, Levett PA, Pot MW, et al., 2013, Gelatin-Methacrylamide Hydrogels as Potential Biomaterials for Fabrication of Tissue-Engineered Cartilage Constructs. Macromol Biosci, 13:551–61. https://doi.org/1002/mabi.201200471
31. Cross LM, Shah K, Palani S, et al., 2018, Gradient Nanocomposite Hydrogels for Interface Tissue Engineering. Nanomed Nanotechnol Biol Med, 14:2465–74. https://doi.org/1016/j.nano.2017.02.022
32. Wang N, Ma M, Luo Y, et al., 2018, Mesoporous Silica Nanoparticles-Reinforced Hydrogel Scaffold together with Pinacidil Loading to Improve Stem Cell Adhesion. ChemNanoMat, 4:631–41. https://doi.org/1002/cnma.201800026
33. Spencer AR, Primbetova A, Koppes AN, et al., 2018, Electroconductive Gelatin Methacryloyl-PEDOT: PSS Composite Hydrogels: Design, Synthesis, and Properties. ACS Biomater Sci Eng, 4:1558–67. https://doi.org/1021/acsbiomaterials.8b00135
34. Kaemmerer E, Melchels FP, Holzapfel BM, et al., 2014, Gelatine Methacrylamide-based Hydrogels: An Alternative Three-dimensional Cancer Cell Culture System. Acta Biomater, 10:2551–62. https://doi.org/1016/j.actbio.2014.02.035
35. Zhang Y, Sun M, Liu T, et al., 2021, Effect of Different Additives on the Mechanical Properties of Gelatin Methacryloyl Hydrogel: A Meta-analysis. ACS Omega, 6:9112–28. https://doi.org/1021/acsomega.1c00244
36. Di Carmine M, Toto P, Feliciani C, et al., 2003, Spreading of Epithelial Cells on Machined and Sandblasted Titanium Surfaces: An In Vitro Study. J Periodontol, 74:289–95. https://doi.org/1902/jop.2003.74.3.289
37. Bacakova L, Filova E, Parizek M, et al., 2011, Modulation of Cell Adhesion, Proliferation and Differentiation on Materials Designed for Body Implants. Biotechnol Adv, 29:739–67. https://doi.org/1016/j.biotechadv.2011.06.004
38. Yao X, Peng R, Ding J, 2013, Cell-Material Interactions Revealed Via Material Techniques of Surface Patterning. Adv Mater, 25:5257–86. https://doi.org/1002/adma.201301762
39. Martin P, 1997, Wound Healing--Aiming for Perfect Skin Regeneration. Science, 276:75. https://doi.org/1126/science.276.5309.75
40. Saghiri MA, Asatourian A, Orangi J, et al., 2015, Functional Role of Inorganic Trace Elements in Angiogenesis Part II: Cr, Si, Zn, Cu, and S. Crit Rev Oncol Hematol, 96:143–55. https://doi.org/1016/j.critrevonc.2015.05.011
41. Dashnyam K, Jin GZ, Kim JH, et al., 2017, Promoting Angiogenesis with Mesoporous Microcarriers through a Synergistic Action of Delivered Silicon Ion and VEGF. Biomaterials, 116:145–57. https://doi.org/1016/j.biomaterials.2016.11.053
42. Ma J, Qin C, Wu J, et al., 2021, 3D Printing of Strontium Silicate Microcylinder-Containing Multicellular Biomaterial Inks for Vascularized Skin Regeneration. Adv Healthc Mater, 10:2100523. https://doi.org/1002/adhm.202100523
43. Zhai W, Lu H, Chen L, et al., 2012, Silicate Bioceramics Induce Angiogenesis During Bone Regeneration. Acta Biomater, 8:341–9. https://doi.org/1016/j.actbio.2011.09.008
44. Ahluwalia A, Tarnawski AS, 2012, Critical Role of Hypoxia Sensor HIF-1 alpha in VEGF Gene Activation. Implications for Angiogenesis and Tissue Injury Healing. Curr Med Chem, 19:90–7.
45. Pugh CW, Ratcliffe PJ, 2003, Regulation of Angiogenesis by Hypoxia: Role of the HIF System. Nat Med, 9:677–84. https://doi.org/1038/nm0603-677
46. Kogata N, Arai Y, Hashimoto K, et al., 2004, Vascular Endothelial Cadherin-Expressing Cells Involved in Both Developmental and Adult Neovascularization. Blood, 104:2610–26. https://doi.org/1182/blood.V104.11.26
47. Zachary I, 1998, Vascular Endothelial Growth Factor. Int J Biochem Cell Biol, 30:1169–74. https://doi.org/1016/S1357-2725(98)00082-X
48. Karkkainen MJ, Petrova TV, 2000, Vascular Endothelial Growth Factor Receptors in the Regulation of Angiogenesis and Lymphangiogenesis. Oncogene, 19:5598–605. https://doi.org/1038/sj.onc.1203855
49. Loucaide S, Cappelle PV, Behrends T, 2008, Dissolution of biogenic silica from land to ocean: Role of salinity and pH. Limnol Oceanogr, 53:1614–21. https://doi.org/4319/lo.2008.53.4.1614
50. Dove PM, Crerar DA, 1990, Kinetics of Quartz Dissolution in Electrolyte-solutions Using a Hydrothermal Mixed Flow Reactor. Geochim Cosmochim Acta, 54:955–69. https://doi.org/1016/0016-7037(90)90431-j
51. Sirova M, Vlierberghe SV, Matyasova V, et al., 2014, Immunocompatibility Evaluation of Hydrogel-coated Polyimide Implants for Applications in Regenerative Medicine. J Biomed Mater Res Part A, 102:1982–90. https://doi.org/1002/jbm.a.34873
52. Klotz BJ, Gawlitta D, Rosenberg AJ, et al., 2016, Gelatin-Methacryloyl Hydrogels: Towards Biofabrication-Based Tissue Repair. Trends Biotechnol, 34:394–407. https://doi.org/1016/j.tibtech.2016.01.002
53. Huang X, Guo X, Qu L, et al., 2021, Gradient Regulation of Osteo-immune Microenvironment by Chito oligosaccharide containing Ion-doped Mesoporous Silica Nanoparticles to Accelerate Osteogenesis. Appl Mater Today, 23:101067. https://doi.org/1016/j.apmt.2021.101067
54. Chen Y, Zhang X, Liu Z, et al., 2021, Obstruction of the Formation of Granulation Tissue Leads to Delayed Wound Healing after Scald Burn Injury in Mice. Burns Trauma, 9:tkab004. https://doi.org/1093/burnst/tkab004
55. Tuan TL, Nichter LS, 1998, The Molecular Basis of Keloid and Hypertrophic Scar Formation. Mol Med Today, 4:19–24. https://doi.org/1016/S1357-4310(97)80541-2
56. Albelda SM, Muller WA, Buck CA, et al., 1991, Molecular and cellular properties of pecam-1 (ENDOCAM/CD31) a novel vascular cell cell-adhesion molecule. J Cell Biol, 114:1059–68. https://doi.org/1083/jcb.114.5.1059
57. Arcangeli G, Cupelli V, Giuliano G, 2001, Effects of Silica on Human Lung Fibroblast in Culture. Sci Total Environ, 270:135–9. https://doi.org/1016/s0048-9697(00)00781-6
58. Park JU, Jung HD, Song EH, et al., 2017, The Accelerating Effect of Chitosan-silica Hybrid Dressing Materials on the Early Phase of Wound Healing. J Biomed Mater Res B Appl Biomater, 105:1828–39. https://doi.org/1002/jbm.b.33711
59. Enciso N, Avedillo L, Fermín ML, et al., 2020, Cutaneous Wound Healing: Canine Allogeneic ASC Therapy. Stem Cell Res Ther, 11:261. https://doi.org/1186/s13287-020-01778-5
60. Brokesh AM, Gaharwar AK, 2020, Inorganic Biomaterials for Regenerative Medicine. ACS Appl Mater Interfaces, 12:5319–44. https://doi.org/1021/acsami.9b17801
61. Reffitt DM, Ogston N, Jugdaohsingh R, et al., 2003, Orthosilicic Acid Stimulates Collagen Type 1 Synthesis and Osteoblastic Differentiation in Human Osteoblast-like Cells In Vitro. Bone, 32:127–35. https://doi.org/1016/S8756-3282(02)00950-X
62. Carmeliet P, Jain RK, 2011, Molecular Mechanisms and Clinical Applications of Angiogenesis. Nature, 473:298–307. https://doi.org/1038/nature10144
63. Kargozar S, Baino F, Hamzehlou S, et al., 2018, Bioactive Glasses: Sprouting Angiogenesis in Tissue Engineering. Trends Biotechnol, 36:430–44. https://doi.org/1016/j.tibtech.2017.12.003
64. Eming SA, Martin P, Tomic-Canic M, 2014, Wound Repair and Regeneration: Mechanisms, Signaling, and Translation. Sci Transl Med, 6:265sr6. https://doi.org/1126/scitranslmed.3009337
65. Zhang X, Yang H, Li S, et al., 2018, Natural Diatomite Particles: Size-, Dose- and Shape- Dependent Cytotoxicity and Reinforcing Effect on Injectable Bone Cement. J Mater Sci Technol, 34:1044–53. https://doi.org/1016/j.jmst.2017.04.020
66. Korunic Z, 1998, Review Diatomaceous Earths, a Group of Natural Insecticides. J Stored Prod Res, 34:87–97. https://doi.org/1016/S0022-474X(97)00039-8.