Selective Laser Melted Rare Earth Magnesium Alloy with High Corrosion Resistance
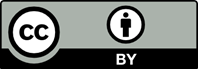
Magnesium (Mg) degrades too fast in human body, which limits its orthopedic application. Single-phase Mgbased supersaturated solid solution is expected to possess high corrosion resistance. In this work, rare earth scandium (Sc) was used as alloying element to prepare Mg(Sc) solid solution powder by mechanical alloying (MA) and then shaped into implant using selective laser melting (SLM). MA utilizes powerful mechanical force to introduce numerous lattice defects, which promotes the dissolution of Sc in Mg matrix and forms supersaturated solid solution particles. Subsequently, SLM with fast heating and cooling rate maintains the original supersaturated solid solution structure. Immersion tests revealed that high Sc content significantly enhanced the corrosion resistance of Mg matrix because of the formation of protective corrosion product film, which was also proved by the electrochemical impedance spectroscopy measurements. Thereby, Mg(Sc) alloy showed a relatively low degradation rate of 0.61 mm/year. In addition, cell tests showed that the Mg(Sc) exhibited favorable biocompatibility and was suitable for medical application.
1. Wang JL, Xu JK, Hopkins C, et al., 2020, Biodegradable Magnesium‐agnesiImplants in Orthopedics a General Review and Perspectives. Adv Sci, 7:1902443. https://doi.org/10.1002/advs.201902443
2. Zhou H, Liang B, Jiang H, et al., 2021, Magnesium-based Biomaterials as Emerging Agents for Bone Repair and Regeneration: From Mechanism to Application. J Magnes Alloys, 9:779–804. https://doi.org/10.1016/j.jma.2021.03.004
3. Atrens A, Johnston S, Shi Z, et al., 2018, Understanding Mg Corrosion in the Body for Biodegradable Medical Implants. Script Mater, 154:92–100. https://doi.org/10.1016/j.scriptamat.2018.05.021
4. Wang S, Zhang X, Li J, et al., 2020, Investigation of Mg-Zn-Y-Nd Alloy for Potential Application of Biodegradable Esophageal Stent Material. Bioact Mater, 5:1–8. https://doi.org/10.1016/j.bioactmat.2020.01.002
5. Wang J, Xu J, Wang X, et al., 2021, Magnesium-pretreated Periosteum for Promoting Bone-tendon Healing after Anterior Cruciate Ligament Reconstruction. Biomaterials, 268:120576. https://doi.org/10.1016/j.biomaterials.2020.120576
6. Dong Q, Zhang M, Zhou X, et al., 2021, 3D-printed Mg-Incorporated PCL-based Scaffolds: A Promising Approach for Bone Healing. Mater Sci Eng C, 129:112372. https://doi.org/10.1016/j.msec.2021.112372
7. Han HS, Jun I, Seok HK, et al., 2020, Biodegradable Magnesium Alloys Promote Angioncial interests that could have influeAdv Sci, 7:2000800. https://doi.org/10.1002/advs.202000800
8. Bommala VK, Krishna MG, Rao CT, 2019, Magnesium Matrix Composites for Biomedical Applications: A Review. J Magnes Alloys, 7:72–9. https://doi.org/10.1016/j.jma.2018.11.001
9. Lai Y, Li Y, Cao H, et al., 2019, Osteogenic Magnesium Incorporated into PLGA/TCP Porous Scaffold by 3D Printing for Repairing Challenging Bone Defect. Biomaterials, 197:207–19. https://doi.org/10.1016/j.biomaterials.2019.01.013
10. Yang Y, He C, Dianyu E, et al., 2020, Mg Bone Implant: Features, Developments And Perspectives. Mater Des, 185:108259. https://doi.org/10.1016/j.matdes.2019.108259
11. Chen H, Yuan B, Zhao R, et al., 2021, Evaluation on the Corrosion Resistance, Antibacterial Property and Osteogenic Activity of Biodegradable Mg-Ca and Mg-Ca-Zn-Ag Alloys. J Magnes Alloys, In Press https://doi.org/10.1016/j.jma.2021.05.013
12. Jin S, Zhang D, Lu X, et al., 2020, Mechanical Properties, Biodegradability and Cytocompatibility of Biodegradable Mg-Zn-Zr-Nd/Y Alloys. J Mater Sci Technol, 47:190–201. https://doi.org/10.1016/j.jmst.2020.02.017
13. Yan C, Xin Y, Chen XB, et al., 2021, Evading Strength corrosion Tradeoff in Mg Alloys Via Dense Ultrafine Twins. Nat Commun, 12:1–9. https://doi.org/10.1038/s41467-021-24939-3
14. Feng J, Li H, Deng K, et al., 2018, Unique Corrosion Resistance of Ultrahigh Pressure Mg-25Al Binary Alloys. Corrosion Sci, 143:229–39. https://doi.org/10.1016/j.corsci.2018.08.013
15. Han C, Fang Q, Shi Y, et al., 2020, Recent Advances on Highighnces Alloys for 3D Printing. Adv Mater, 32:1903855. https://doi.org/10.1002/adma.201903855
16. Goncharov I, Razumov N, Silin A, et al., 2019, Synthesis of Nb-based Powder alloy by Mechanical Alloying and Plasma Spheroidization Processes for Additive Manufacturing. Mater Lett, 245:188–91. https://doi.org/10.1016/j.matlet.2019.03.014
17. Xiao B, Xu L, Cayron C, et al., 2020, Solute-dislocation Interactions and Creep-enhanced Cu Precipitation in a Novel Ferritic-martensitic Steel. Acta Mater, 195:199–208. https://doi.org/10.1016/j.actamat.2020.05.054
18. Geng Y, Mo Y, Zheng H, et al., 2021, Effect of Laser Shock Peening on the hot Corrosion Behavior of Ni-based Single crystal Superalloy at 750° C. Corrosion Sci, 185:109419. https://doi.org/10.1016/j.corsci.2021.109419
19. Li L, Shi J, Ma K, et al., 2021, Robotic In Situ 3D Bioprinting Technology for Repairing Large Segmental Bone Defects. J Adv Res, 30:75–84. https://doi.org/10.1016/j.jare.2020.11.011
20. Sing SL, 2022, Perspectives on Additive Manufacturing Enabled Beta-Titanium Alloys for Biomedical Applications. Int J Bioprint, 8:478. https://doi.org/10.18063/ijb.v8i1.478
21. Zhang H, Gu D, Dai D, 2022, Laser Printing Path and its Influence on Molten Pool Configuration, Microstructure and Mechanical Properties of Laser Powder Bed Fusion Processed Rare Earth Element Modified Al-Mg Alloy. Virtual Phys Prototyp, 17:308–28. https://doi.org/10.1080/17452759.2022.2036530
22. Sing S, Kuo C, Shih C, et al., 2021, Perspectives of Using Machine Learning in Laser Powder Bed Fusion for Metal Additive Manufacturing. Virtual Phys Prototyp, 16:372–86. https://doi.org/10.1080/17452759.2021.1944229
23. Wang D, Liu L, Deng G, et al., 2022, Recent Progress on Additive Manufacturing of Multi-material Structures with Laser Powder Bed Fusion. Virtual Phys Prototyp, 17:329–65. https://doi.org/10.1080/17452759.2022.2028343
24. Shuai C, He C, Qian G, et al., 2021, Mechanically Driving Supersaturated Fe-Mg Solid Solution for Bone Implant: Preparation, Solubility and Degradation. Compos Part B Eng, 207:108564. https://doi.org/10.1016/j.compositesb.2020.108564
25. Li B, Han C, Lim CW, et al., 2022, Interface Formation and Deformation Behaviors of an Additively Manufactured Nickel-aluminum-bronze/15-5 PH Multimaterial Via Laserpowder Directed Energy Deposition. Mater Sci Eng A, 829:142101. https://doi.org/10.1016/j.msea.2021.142101
26. Zhang T, Liu CT, 2021, Design of Titanium Alloys by Additive Manufacturing: A Critical Review. Adv Powder Mater, 1:100014. https://doi.org/10.1016/j.apmate.2021.11.001
27. Esmaily M, Zeng Z, Mortazavi A, et al., 2020, A Detailed Microstructural and Corrosion Analysis of Magnesium Alloy WE43 Manufactured by Selective Laser Melting. Addit Manuf, 35:101321. https://doi.org/10.1016/j.addma.2020.101321
28. Ogawa Y, Ando D, Sutou Y, et al., 2016, A Lightweight Shape-memory Magnesium Alloy. Science, 353:368–70. https://doi.org/10.1126/science.aaf6524
29. Cacace S, Semeraro Q, 2021, Fast Optimisation Procedure for the Selection of L-PBF Parameters Based on Utility Function. Virtual Phys Prototyp, 1-13. https://doi.org/10.1080/17452759.2021.1998871
30. Yu W, Xiao Z, Zhang X, et al., 2022, Processing and Characterization of Crack-free 7075 Aluminum Alloys with Elemental Zr Modification by Laser Powder Bed Fusion. Int J Bioprint, 1:4. http://dx.doi.org/10.18063/msam.v1i1.4
31. Huang S, Narayan RL, Tan JH, et al., 2021, Resolving the Porosity-unmelted Inclusion Dilemma during In-Situ Alloying of Ti34Nb via Laser Powder Bed Fusion. Acta Mater, 204:116522. https://doi.org/10.1016/j.actamat.2020.116522
32. Munk J, Breitbarth E, Siemer T, et al., 2022, Geometry Effect on Microstructure and Mechanical Properties in Laser Powder Bed Fusion of Ti-6Al-4V. Metals, 12:482. https://doi.org/10.3390/met12030482
33. Lapointe S, Guss G, Reese Z, et al., 2022, Photodiode-based Machine Learning for Optimization of Laser Powder Bed Fusion Parameters in Complex Geometries. Addit Manuf, 53:102687. https://doi.org/10.1016/j.addma.2022.102687
34. Gong X, Zeng D, Groeneveld-Meijer W, 2022, Additive Manufacturing: A Machine Learning Model of Process-Structure-property Linkages for Machining Behavior of Ti-6Al-4V. Mater Sci Add Manuf, 1:16. https://doi.org/10.18063/msam.v1i1.6
35. Cao F, Shi Z, Hofstetter J, et al., 2013, Corrosion of Ultrahigh-purity Mg in 3.5% NaCl Solution Saturated with Mg(OH) 2. Corrosion Sci, 75:78–99. https://doi.org/10.1016/j.corsci.2013.05.018
36. Peng Q, Meng J, Li Y, et al., 2011, Effect of Yttrium Addition on Lattice Parameter, Young’s Modulus and Vacancy of Magnesium. Mater Sci Eng A, 528:2106–9. https://doi.org/10.1016/j.msea.2010.11.042
37. Shang C, Axinte E, Sun J, et al., 2017, CoCrFeNi (W1-xMox) High-entropy Alloy Coatings with Excellent Mechanical Properties and Corrosion Resistance Prepared by Mechanical Alloying and Hot Pressing Sintering. Mater Des, 117:193–202. https://doi.org/10.1016/j.matdes.2016.12.076
38. Ma C, Gu D, Dai D, et al., 2015, Aluminum-based Nanocomposites with Hybrid Reinforcements Prepared by Mechanical Alloying and Selective Laser Melting Consolidation. J Mater Res, 30:2816–28. https://doi.org/10.1557/jmr.2015.267
39. Sankhla A, Patil A, Kamila H, et al., 2018, Mechanical Alloying of Optimized Mg2 (Si, Sn) Solid Solutions: Understanding Phase Evolution and Tuning Synthesis Parameters for Thermoelectric Applications. ACS Appl Energy Mater, 1:531–42. https://doi.org/10.1021/acsaem.7b00128
40. Wang C, Chen J, Li B, et al., 2021, Cathodic Behavior of Scandium (III) on Reactive Copper Electrode in LiF-CaF2 Eutectic Molten Salt∗. J Rare Earths. https://doi.org/10.1016/j.jre.2021.08.011
41. Cai X, Ding S, Li Z, et al., 2021, Simultaneous Sintering of Low-melting-point Mg with High-melting-point Ti Via a Novel One-step High-pressure Solid-phase Sintering Strategy. J Alloys Compounds, 858:158344. https://doi.org/10.1016/j.jallcom.2020.158344
42. Li B, Zheng H, Han C, et al., 2021, Nanotwins-containing Microstructure and Superior Mechanical Strength of a Cu-9Al-5Fe-5Ni Alloy Additively Manufactured by Laser Metal Deposition. Addit Manuf, 39:101825. https://doi.org/10.1016/j.addma.2020.101825
43. Wang C, Shuai Y, Yang Y, et al., 2022, Amorphous Magnesium Alloy with High Corrosion Resistance Fabricated by Laser Powder Bed Fusion. J Alloys Compounds, 897:163247. https://doi.org/10.1016/j.jallcom.2021.163247
44. Bahl S, Mishra S, Yazar K, et al., 2019, Non-equilibrium Microstructure, Crystallographic Texture and Morphological Texture Synergistically Result in Unusual Mechanical Properties of 3D Printed 316L Stainless Steel. Addit Manuf, 28:65–77. https://doi.org/10.1016/j.addma.2019.04.016
45. Yang M, Shuai Y, Yang Y, et al., 2022, In Situ Grown Rare Earth Lanthanum on Carbon Nanofibre for Interfacial Reinforcement in Zn Implants. Virtual Phys Prototyp, 17:700–17. https://doi.org/10.1080/17452759.2022.2053929
46. Montiel H, Xu B, Li J, 2019, Selective Laser Melting of Mechanically Alloyed Metastable Al5Fe2 Powders. J Manuf Sci Eng, 141:071008. https://doi.org/10.1115/1.4043730
47. Liu X, Shan D, Song Y, et al., 2011, Influences of the Quantity of Mg2Sn Phase on the Corrosion Behavior of Mg-7Sn Magnesium Alloy. Electrochim Acta, 56:2582–90. https://doi.org/10.1016/j.electacta.2010.12.030
48. Song Y, Han EH, Dong K, et al., 2014, Study of the Corrosion Product Films Formed on the Surface of Mg-xZn Alloys in NaCl Solution. Corrosion Sci, 88:215–25. https://doi.org/10.1016/j.corsci.2014.07.034
49. Yang Y, Lu C, Shen L, et al., 2021, In-Situ Deposition of Apatite Layer to Protect Mg-based Composite Fabricated via Laser Additive Manufacturing. J Magnes Alloys, Available online. https://doi.org/10.1016/j.jma.2021.04.009
50. Song Y, Shan D, Han EH, 2017, Pitting Corrosion of a Rare Earth Mg Alloy GW93. J Mater Sci Technol, 33:954–60. https://doi.org/10.1016/j.jmst.2017.01.014
51. Chen X, Ning S, Wang A, et al., 2020, Microstructure, Mechanical Properties and Corrosion Behavior of Quasicrystal-reinforced Mg-Zn-Y Alloy Subjected to Dual frequency Ultrasonic Field. Corrosion Sci, 163:108289. https://doi.org/10.1016/j.corsci.2019.108289
52. Jiang W, Wang J, Zhao W, et al., 2019, Effect of Sn Addition on the Mechanical Properties and Bio-corrosion Behavior of Cytocompatible Mg-4Zn Based Alloys. J Magnes Alloys, 7:15–26. https://doi.org/10.1016/j.jma.2019.02.002
53. Orazem ME, Frateur I, Tribollet B, et al., 2013, Dielectric Properties of Materials Showing Constant-phase-element (CPE) Impedance Response. J Electrochem Soc, 160:C215. https://doi.org/10.1149/2.033306jes
54. Hirschorn B, Orazem ME, Tribollet B, et al., 2010, Determination of Effective Capacitance and Film Thickness from Constant-phase-element Parameters. Electrochim Acta, 55:6218–27. https://doi.org/10.1016/j.electacta.2009.10.065
55. Wang X, Chen Z, Ren J, et al., 2020, Corrosion Behavior of as-cast Mg-5Sn Based Alloys with in Additions in 3.5 wt% NaCl Solution. Corrosion Sci, 164:108318. https://doi.org/10.1016/j.corsci.2019.108318
56. Narayanan TS, Lee MH, 2016, A Simple Strategy to Modify the Porous Structure of Plasma Electrolytic Oxidation Coatings on Magnesium. Rsc Adv, 6:16100–14. https://doi.org/10.1039/C5RA20647B
57. Zhang C, Wu L, Liu H, et al., 2020, Microstructure and Corrosion Behavior of Mg-Sc Binary Alloys in 3.5 wt.% NaCl Solution. Corrosion Sci, 174:108831. https://doi.org/10.1016/j.corsci.2020.108831
58. Ma K, Zhao T, Yang L, et al., 2020, Application of Robotic assisted In Situ 3D Printing in Cartilage Regeneration with HAMA hydrogel: An In Vivo Study. J Adv Res, 23:123–32. https://doi.org/10.1016/j.jare.2020.01.010
59. Qi F, Liao R, Shuai Y, et al., 2022, A Conductive Network Enhances Nerve Cell Response. Addit Manuf, 52:102694. https://doi.org/10.1016/j.addma.2022.102694
60. Qi F, Gao X, Shuai Y, et al., 2022, Magnetic-driven Wireless Electrical Stimulation in a Scaffold. Compos Part B Eng, 237:109864. https://doi.org/10.1016/j.compositesb.2022.109864
61. Munir K, Lin J, Wen C, et al., 2020, Mechanical, Corrosion, and Biocompatibility Properties of Mg-Zr-Sr-Sc Alloys for Biodegradable Implant Applications. Acta Biomater, 102:493–507. https://doi.org/10.1016/j.actbio.2019.12.001
62. Zheng L, Zhuang Z, Li Y, et al., 2021, Bone Targeting Antioxidative Nano-iron Oxide for Treating Postmenopausal Osteoporosis. Bioact Mater, 14:250–61. https://doi.org/10.1016/j.bioactmat.2021.11.012
63. Qi F, Wang Z, Shuai Y, et al., 2022, Sr2+ Sustained Release System Augments Bioactivity of Polymer Scaffold. ACS Appl Polym Mater, 4:2691–702. https://doi.org/10.1021/acsapm.2c00024
64. Jahnen-Dechent W, Ketteler M, 2012, Magnesium Basics. Clin Kidney J, 5 Suppl 1:i3–14.
65. Liu J, Lin Y, Bian D, et al., 2019, In Vitro and In Vivo Studies of Mg-30Sc Alloys with Different Phase Structure for Potential Usage Within Bone. Acta Biomater, 98:50–66. https://doi.org/10.1016/j.actbio.2019.03.009