3D Composite Bioprinting for Fabrication of Artificial Biological Tissues
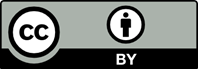
Three-dimensional (3D) bioprinting is an important technology for fabricating artificial tissue. To effectively reconstruct the multiscale structure and multi-material gradient of natural tissues and organs, 3D bioprinting has been increasingly developed into multi-process composite mode. The current 3D composite bioprinting is a combination of two or more printing processes, and oftentimes, physical field regulation that can regulate filaments or cells during or after printing may be involved. Correspondingly, both path planning strategy and process control all become more complex. Hence, the computer-aided design and computer-aided manufacturing (CAD/CAM) system that is traditionally used in 3D printing system is now facing challenges. Thus, the scale information that cannot be modeled in the CAD process should be considered in the design of CAM by adding a process management module in the traditional CAD/CAM system and add more information reflecting component gradient in the path planning strategy.
1. Groll J, Burdick JA, Cho DW, et al., 2018, A Definition of Bioinks and their Distinction from Biomaterial Inks [J]. Biofabrication, 11(1):013001. https://doi.org/10.1088/1758-5090/aaec52
2. Gungor-Ozkerim PS, Inci I, Zhang YS, et al., 2018, Bioinks for 3D Bioprinting: An Overview [J]. Biomater Sci, 6(5):915–46. https://doi.org/10.1039/c7bm00765e
3. Ng WL, Chua CK, Shen YF, 2019, Print Me An Organ! Why We Are Not There Yet [J]. Prog Polym Sci, 97:101145. https://doi.org/10.1016/j.progpolymsci.2019.101145
4. Ng WL, Chan A, Ong YS, et al., 2020, Deep Learning for Fabrication and Maturation of 3D Bioprinted Tissues and Organs [J]. Virtual Phys Prototyp 15(3):340–58
5. Ozbolat IT, Hospodiuk M, 2016, Current Advances and Future Perspectives in Extrusion-based Bioprinting [J]. Biomaterials, 76:321–43. https://doi.org/10.1016/j.biomaterials.2015.10.076
6. Emmermacher J, Spura D, Cziommer J, et al., 2020, Engineering Considerations on Extrusion-based Bioprinting: Interactions of Material Behavior, Mechanical Forces and Cells in the Printing Needle [J]. Biofabrication, 12(2):025022. https://doi.org/10.1088/1758-5090/ab7553
7. Gao G, Kim BS, Jang J, et al., 2019, Recent Strategies in Extrusion-Based Three-Dimensional Cell Printing toward Organ Biofabrication [J]. ACS Biomater Sci Eng, 5(3):1150–69. https://doi.org/10.1021/acsbiomaterials.8b00691
8. Gudapati H, Dey M, Ozbolat I, 2016, A Comprehensive Review on Droplet-based Bioprinting: Past, Present and Future [J]. Biomaterials, 102:20–42. https://doi.org/10.1016/j.biomaterials.2016.06.012
9. Ng WL, Lee JM, Yeong WY, et al., 2017, Microvalve-based Bioprinting Process, Bio-inks and Applications [J]. Biomater Sci, 5(4):632–47. https://doi.org/10.1039/c6bm00861e
10. Koch L, Brandt O, Deiwick A, et al. 2017, Laser-assisted Bioprinting at Different Wavelengths and Pulse Durations with a Metal Dynamic Release Layer: A Parametric Study [J]. Int J Bioprint, 3(1):42–53. https://doi.org/10.18063/ijb.2017.01.001
11. Onses MS, Sutanto E, Ferreira PM, et al., 2015, Mechanisms, Capabilities, and Applications of High-Resolution Electrohydrodynamic Jet Printing [J]. Small, 11(34):4237–66. https://doi.org/10.1002/smll.201500593
12. Ng WL, Lee JM, Zhou M, et al., 2020, Vat Polymerization based Bioprinting Process, Materials, Applications and Regulatory Challenges [J]. Biofabrication, 12(2):022001. https://doi.org/10.1088/1758-5090/ab6034
13. Li W, Mille LS, Robledo JA, et al., 2020, Recent Advances in Formulating and Processing Biomaterial Inks for Vat Polymerization-Based 3D Printing [J]. Adv Healthc Mater, 9(15):2000156. https://doi.org/10.1002/adhm.202000156
14. Quan H, Zhang T, Xu H, et al., 2020, Photo-curing 3D Printing Technique and its Challenges [J]. Bioact Mater, 5(1):110–5.
15. Jun I, Han HS, Edwards JR, et al., 2018, Electrospun Fibrous Scaffolds for Tissue Engineering: Viewpoints on Architecture and Fabrication [J]. Int J Mol Sci, 19(3):745. https://doi.org/10.3390/ijms19030745
16. Liashenko I, Rosell-Llompart J, Cabot A, 2020, Ultrafast 3D Printing with Submicrometer Features Using Electrostatic Jet Deflection [J]. Nat Commun 11(1):753. https://doi.org/10.1038/s41467-020-14557-w
17. Wang C, Meng G, Zhang L, et al., 2012, Physical Properties and Biocompatibility of a Core-sheath Structure Composite Scaffold for Bone Tissue Engineering In Vitro [J]. J Biomed Biotechnol, 2012:579141
18. Kolesky DB, Homan KA, Skylar-Scott MA, et al., 2016, Three-dimensional Bioprinting of thick Vascularized Tissues [J]. Proc Natl Acad Sci U S A, 113(12):3179–84. https://doi.org/10.1073/pnas.1521342113
19. Skylar-Scott MA, Uzel SG, Nam LL, et al., 2019, Biomanufacturing of Organ-specific Tissues with High Cellular Density and Embedded Vascular Channels [J]. Sci Adv, 5(9): eaaw2459. https://doi.org/10.1126/sciadv.aaw2459
20. Wang W, Junior JR, Nalesso PR, et al., 2019, Engineered 3D Printed Poly(ɛ caprolactone)/Graphene Scaffolds for Bone Tissue Engineering [J]. Mater Sci Eng C, 100:759–70. https://doi.org/10.1016/j.msec.2019.03.047
21. Chen YW, Shen YF, Ho CC, et al., 2018, Osteogenic and Angiogenic Potentials of the Cell-laden Hydrogel/Mussel inspired Calcium Silicate Complex Hierarchical Porous Scaffold Fabricated by 3D Bioprinting [J]. Mater Sci Eng C, 91:679–87. https://doi.org/10.1016/j.msec.2018.06.005
22. Mekhileri NV, Lim KS, Brown GC, et al., 2018, Automated 3D Bioassembly of Micro-tissues for Biofabrication of Hybrid Tissue Engineered Constructs [J]. Biofabrication, 10(2):024103. https://doi.org/10.1088/1758-5090/aa9ef1
23. Gao Q, He Y, Fu JZ, et al., 2015, Coaxial Nozzle-assisted 3D Bioprinting with Built-in Microchannels for Nutrients Delivery [J]. Biomaterials, 61:203–15. https://doi.org/10.1016/j.biomaterials.2015.05.031
24. Andrique L, Recher G, Alessandri K, et al., 2019, A Model of Guided Cell Self-organization for Rapid and Spontaneous Formation of Functional Vessels [J]. Sci Adv, 5(6): eaau6562. https://doi.org/10.1126/sciadv.aau6562
25. Jia WT, Gungor-Ozkerim PS, Zhang YS, et al., 2016, Direct 3D Bioprinting of Perfusable Vascular Constructs Using a Blend Bioink [J]. Biomaterials, 106:58–68. https://doi.org/10.1016/j.biomaterials.2016.07.038
26. Millik SC, Dostie AM, Karis DG, et al., 2019, 3D Printed Coaxial Nozzles for the Extrusion of Hydrogel Tubes Toward Modeling Vascular Endothelium [J]. Biofabrication, 11(4):045009. https://doi.org/10.1088/1758-5090/ab2b4d
27. Narayanan LK, Huebner P, Fisher MB, et al., 2016, 3D-Bioprinting of Polylactic Acid (PLA) Nanofiber-Alginate Hydrogel Bioink Containing Human Adipose-Derived Stem Cells [J]. ACS Biomater Sci Eng, 2(10):1732–42. https://doi.org/10.1021/acsbiomaterials.6b00196
28. Tabriz AG, Hermida MA, Leslie NR, et al., 2015, Three dimensional Bioprinting of Complex Cell Laden Alginate Hydrogel Structures [J]. Biofabrication, 7(4):045012. https://doi.org/10.1088/1758-5090/7/4/045012
29. Lozano R, Stevens L, Thompson BC, et al., 2015, 3D Printing of Layered Brain-like Structures Using Peptide Modified Gellan Gum Substrates [J]. Biomaterials, 67:264–73. https://doi.org/10.1016/j.biomaterials.2015.07.022
30. Wang X, Li X, Dai X, et al., 2018, Coaxial Extrusion Bioprinted Shell-core Hydrogel Microfibers Mimic Glioma Microenvironment and Enhance the Drug Resistance of Cancer Cells [J]. Colloids Surf B Biointerfaces, 171:291–9. https://doi.org/10.1016/j.colsurfb.2018.07.042
31. Abel SB, Ballarin FM, Abraham GA, 2020, Combination of Electrospinning with other Techniques for the Fabrication of 3D Polymeric and Composite Nanofibrous Scaffolds with Improved Cellular Interactions [J]. Nanotechnology, 31(17):172002. https://doi.org/10.1088/1361-6528/ab6ab4
32. Lee SJ, Heo DN, Park JS, et al., 2015, Characterization and Preparation of Bio-tubular Scaffolds for Fabricating Artificial Vascular Grafts by Combining Electrospinning and a 3D Printing System [J]. Phys Chem Chem Phys, 17(5):2996–9 https://doi.org/10.1039/c4cp04801f
33. Akentjew TL, Terraza C, Suazo C, et al., 2019, Rapid Fabrication of Reinforced and Cell-laden Vascular Grafts Structurally Inspired by Human Coronary Arteries [J]. Nat Commun, 10(1):3098. https://doi.org/10.1038/s41467-019-11446-9
34. Jungst T, Pennings I, Schmitz M, et al., 2019, Heterotypic Scaffold Design Orchestrates Primary Cell Organization and Phenotypes in Cocultured Small Diameter Vascular Grafts [J]. Adv Funct Mater, 29(43):1905987. https://doi.org/10.1002/adfm.201905987
35. Wang K, Zheng W, Pan Y, et al., 2016, Three-Layered PCL Grafts Promoted Vascular Regeneration in a Rabbit Carotid Artery Model [J]. Macromol Biosci, 16(4):608–18. https://doi.org/10.1002/mabi.201500355
36. Liu Y, Xiang K, Chen H, et al., 2015, Composite Vascular Repair Grafts via Micro-imprinting and Electrospinning [J]. AIP Adv, 5(4):041318. https://doi.org/10.1063/1.4906571
37. Wu P, Wang L, Li W, et al., 2020, Construction of Vascular Graft with Circumferentially Oriented Microchannels for Improving Artery Regeneration [J]. Biomaterials, 242:119922. https://doi.org/10.1016/j.biomaterials.2020.119922
38. Lee SJ, Kim ME, Nah H, et al., 2019, Vascular Endothelial Growth Factor Immobilized on Mussel-inspired Three dimensional Bilayered Scaffold for Artificial Vascular Graft Application: In Vitro and In Vivo Evaluations [J]. J Colloid Interface Sci, 537:333–44. https://doi.org/10.1016/j.jcis.2018.11.039
39. Chen X, Ergun A, Gevgilili H, et al., 2013, Shell-core bilayered Scaffolds for Engineering of Vascularized Osteonlike Structures [J]. Biomaterials, 34:8203–12. https://doi.org/10.1016/j.biomaterials.2013.07.035
40. Costa PF, Vaquette C, Zhang Q, et al., 2014, Advanced Tissue Engineering Scaffold Design for Regeneration of the Complex Hierarchical Periodontal Structure [J]. J Clin Periodontol, 41(3):283–94. https://doi.org/10.1111/jcpe.12214
41. Vaquette C, Fan W, Xiao Y, et al., 2012, A Biphasic Scaffold Design Combined with Cell Sheet Technology for Simultaneous Regeneration of Alveolar Bone/Periodontal Ligament Complex [J]. Biomaterials, 33(22):5560–73. https://doi.org/10.1016/j.biomaterials.2012.04.038
42. Kumar PT, Hashimi S, Saifzadeh S, et al., 2018, Additively Manufactured Biphasic Construct Loaded with BMP-2 for Vertical Bone Regeneration: A Pilot Study in Rabbit [J]. Mater Sci Eng C, 92:554–64. https://doi.org/10.1016/j.msec.2018.06.071
43. De Ruijter M, Ribeiro A, Dokter I, et al., 2019, Simultaneous Micropatterning of Fibrous Meshes and Bioinks for the Fabrication of Living Tissue Constructs [J]. Adv Healthc Mater, 8(7):1800418. https://doi.org/10.1002/adhm.201800418
44. Rajzer I, Kurowska A, Jabłoński A, et al., 2018, Layered Gelatin/PLLA Scaffolds Fabricated by Electrospinning and 3D Printing for Nasal Cartilages and Subchondral Bone Reconstruction [J]. Mater Des, 155:297–306. https://doi.org/10.1016/j.matdes.2018.06.012
45. Diloksumpan P, De Ruijter M, Castilho M, et al., 2020, Combining Multi-scale 3D Printing Technologies to Engineer Reinforced Hydrogel-ceramic Interfaces [J]. Biofabrication, 12(2):025014. https://doi.org/10.1088/1758-5090/ab69d9
46. Park JA, Lee HR, Park SY, et al., 2020, Self-Organization of Fibroblast-Laden 3D Collagen Microstructures from Inkjet-Printed Cell Patterns [J]. Adv Biosyst, 4(5):1900280. https://doi.org/10.1002/adbi.201900280
47. Kérourédan O, Bourget JM, Rémy M, et al., 2019, Micropatterning of Endothelial Cells to Create a Capillary like Network with Defined Architecture by Laser-assisted Bioprinting [J]. J Mater Sci Mater Med, 30(2):28. https://doi.org/10.1007/s10856-019-6230-1
48. Kérourédan O, Hakobyan D, Rémy M, et al., 2019, In Situ Prevascularization Designed by Laser-assisted Bioprinting: Effect on Bone Regeneration [J]. Biofabrication, 11(4):045002. https://doi.org/10.1088/1758-5090/ab2620
49. Chen H, Liu Y, Hu Q, 2015, A Novel Bioactive Membrane by Cell Electrospinning [J]. Exp Cell Res, 338(2):261–6.
50. Redd MA, Zeinstra N, Qin W, et al., 2019, Patterned Human Microvascular Grafts Enable Rapid Vascularization and Increase Perfusion in Infarcted Rat Hearts [J]. Nat Commun, 10(1):584. https://doi.org/10.1038/s41467-019-08388-7
51. Clyne AM, Swaminathan S, Lantada AD, 2019, Biofabrication Strategies for Creating Microvascular Complexity [J]. Biofabrication, 11(3):032001. https://doi.org/10.1088/1758-5090/ab0621
52. Chandra P, Atala A, 2019, Engineering Blood Vessels and Vascularized Tissues: Technology Trends and Potential Clinical Applications [J]. Clin Sci, 133(9):1115–35. https://doi.org/10.1042/cs20180155
53. Rouwkema J, Khademhosseini A, 2016, Vascularization and Angiogenesis in Tissue Engineering: Beyond Creating Static Networks [J]. Trends Biotechnol, 34(9):733–45. https://doi.org/10.1016/j.tibtech.2016.03.002
54. Kinstlinger IS, Miller JS, 2016, 3D-printed Fluidic Networks as Vasculature for Engineered Tissue [J]. Lab Chip, 16(11):2025–43. https://doi.org/10.1039/c6lc00193a
55. Miri AK, Khalilpour A, Cecen B, et al., 2019, Multiscale Bioprinting of Vascularized Models [J]. Biomaterials, 198:204–16. https://doi.org/10.1016/j.biomaterials.2018.08.006
56. Sharma D, Ross D, Wang G, et al., 2019, Upgrading Prevascularization in Tissue Engineering: A Review of Strategies for Promoting Highly Organized Microvascular Network Formation [J]. Acta Biomater, 95:112–30. https://doi.org/10.1016/j.actbio.2019.03.016
57. Daly AC, Pitacco P, Nulty J, et al., 2018, 3D Printed Microchannel Networks to Direct Vascularisation during Endochondral Bone Repair [J]. Biomaterials, 162:34–46. https://doi.org/10.1016/j.biomaterials.2018.01.057
58. Pimentel CR, Ko SK, Caviglia C, et al., 2018, Three dimensional Fabrication of Thick and Densely Populated Soft Constructs with Complex and Actively Perfused Channel Network [J]. Acta Biomater, 65:174–84. https://doi.org/10.1016/j.actbio.2017.10.047
59. Negrini NC, Bonnetier M, Giatsidis G, et al., 2019, Tissue mimicking Gelatin Scaffolds by Alginate Sacrificial Templates for Adipose Tissue Engineering [J]. Acta Biomater, 87:61–75. https://doi.org/10.1016/j.actbio.2019.01.018
60. Ji S, Almeida E, Guvendiren M, 2019, 3D Bioprinting of Complex Channels within Cell-laden Hydrogels [J]. Acta Biomater, 95:214–24. https://doi.org/10.1016/j.actbio.2019.02.038
61. Ouyang L, Armstrong JP, Chen Q, et al., 2020, Void-Free 3D Bioprinting for In Situ Endothelialization and Microfluidic Perfusion [J]. Adv Funct Mater, 30(1):1908349. https://doi.org/10.1002/adfm.201908349
62. Kim BS, Gao G, Kim JY, et al., 2019, 3D Cell Printing of
Perfusable Vascularized Human Skin Equivalent Composed
of Epidermis, Dermis, and Hypodermis for Better Structural
Recapitulation of Native Skin [J] Adv. Healthcare Mater,
8(7):1801019. https://doi.org/10.1002/adhm.201801019
63. Kang HW, Lee SJ, Ko IK, et al., 2016, A 3D Bioprinting System to Produce Human-scale Tissue Constructs with Structural Integrity [J]. Nat Biotechnol, 34(3):312–9. https://doi.org/10.1038/nbt.3413
64. Noor N, Shapira A, Edri R, et al., 2019, 3D Printing of Personalized Thick and Perfusable Cardiac Patches and Hearts [J]. Adv Sci, 6(11):1900344. https://doi.org/10.1002/advs.201900344
65. Yang Y, Chen Z, Song X, et al., 2017, Biomimetic Anisotropic Reinforcement Architectures by Electrically Assisted Nanocomposite 3D Printing [J]. Adv Mater, 29(11):1605750. https://doi.org/10.1002/adma.201605750
66. Chansoria P, Shirwaiker R, 2019, Characterizing the Process Physics of Ultrasound-Assisted Bioprinting [J]. Sci Rep, 9(1):13889. https://doi.org/10.1038/s41598-019-50449-w
67. Kirillova A, Maxson R, Stoychev G, et al., 2017, 4D Biofabrication Using Shape-Morphing Hydrogels [J]. Adv Mater, 29(46):1703443. https://doi.org/10.1002/adma.201703443
68. Kokkinis D, Schaffner M, Studart AR, 2015, Multimaterial Magnetically Assisted 3D Printing of Composite Materials [J]. Nat Commun, 6(1):8643. https://doi.org/10.1038/ncomms9643
69. Kim Y, Yuk H, Zhao R, et al., 2018, Printing Ferromagnetic Domains for Untethered Fast-transforming Soft Materials [J]. Nature, 558(7709):274–9. https://doi.org/10.1038/s41586-018-0185-0
70. Shim JH, Lee JS, Kim JY, et al., 2012, Bioprinting of a Mechanically Enhanced Three-dimensional Dual Cell-laden Construct for Osteochondral Tissue Engineering Using a Multi-head Tissue/organ Building System [J]. J Micromech Microeng, 22(8):085014. https://doi.org/10.1088/0960-1317/22/8/085014
71. Marga F, Jakab K, Khatiwala C, et al., 2012, Toward Engineering Functional Organ Modules by Additive Manufacturing [J]. Biofabrication, 4(2):022001. https://doi.org/10.1088/1758-5082/4/2/022001
72. Whatley BR, Kuo J, Shuai C, et al., 2011, Fabrication of a Biomimetic Elastic Intervertebral Disk Scaffold Using Additive Manufacturing [J]. Biofabrication, 3(1):015004. https://doi.org/10.1088/1758-5082/3/1/015004
73. Chen Z, Li D, Lu B, et al., 2004, Fabrication of Artificial Bioactive Bone Using Rapid Prototyping [J]. Rapid Prototyp J, 10(5):327–33. https://doi.org/10.1108/13552540410562368
74. Liu L, Xiong Z, Yan Y, et al., 2007, Porous Morphology, Porosity, Mechanical Properties of Poly (α-hydroxy acid)-Tricalcium Phosphate Composite Scaffolds Fabricated by Low-temperature Deposition [J]. J Biomed Mater Res Part A, 82A(3):618–29. https://doi.org/10.1002/jbm.a.31177
75. Liu TK, Pang Y, Zhou ZZ, et al., 2019, An Integrated Cell Printing System for the Construction of Heterogeneous Tissue Models [J]. Acta Biomater, 95:245–57. https://doi.org/10.1016/j.actbio.2019.05.052
76. Xie C, Gao Q, Wang P, et al., 2019, Structure-induced Cell Growth by 3D Printing of Heterogeneous Scaffolds with Ultrafine Fibers [J]. Mater Des, 181:108092. https://doi.org/10.1016/j.matdes.2019.108092
77. Liu D, 2012, Multiphysics Coupling Analysis and Experiment of Low-temperature Deposition Manufacturing and Electrospinning for Multi-scale Tissue Engineering Scaffold [J]. J Mech Eng, 48(15):137–43. https://doi.org/10.3901/jme.2012.15.137
78. Dali L, Jun G, Shuhui F, et al., 2012, On-line Monitor System
for Nanoscale Fiber Manufacturing Based on Multi-featured
Pattern Recognition [J]. Opt Precis Eng, 20(2):360–8.
https://doi.org/10.37h88/OPE.20122002.0360
79. Liu Y. 2014, CAD/CAM System and Experimental Study of Biological 3D Printing Composite Process. J Mech Eng, 50(15):147. https://doi.org/10.3901/jme.2014.15.147