Extrusion-Based Bioprinting through Glucose-Mediated Enzymatic Hydrogelation
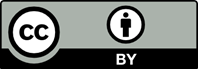
We report an extrusion-based bioprinting approach, in which stabilization of extruded bioink is achieved through horseradish peroxidase (HRP)-catalyzed cross-linking consuming hydrogen peroxide (H2 O2 ) supplied from HRP and glucose. The bioinks containing living cells, HRP, glucose, alginate possessing phenolic hydroxyl (Ph) groups, and cellulose nanofiber were extruded to fabricate 3D hydrogel constructs. Lattice- and human nose-shaped 3D constructs were successfully printed and showed good stability in cell culture medium for over a week. Mouse 10T1/2 fibroblasts enclosed in the printed constructs remained viable after 7 days of culture. It was also able to switch a non-cell-adhesive surface of the printed construct to celladhesive surface for culturing cells on it through a subsequent cross-linking of gelatin possessing Ph moieties. These results demonstrate the possibility of utilizing the presented cross-linking method for 3D bioprinting.
1. An J, Teoh JEM, Suntornnond R, et al., 2015, Design and 3D Printing of Scaffolds and Tissues. Engineering, 1(2):261–8. DOI 10.15302/J-ENG-2015061.
2. Liu F, Mishbak H, Bartolo P, et al., 2019, Hybrid Polycaprolactone/Hydrogel Scaffold Fabrication and Inprocess Plasma Treatment Using PABS. Int J Bioprint, 5(1):174. DOI: 10.18063/ijb.v5i1.174.
3. Yang Y, Wang G, Liang H, et al., 2019, Additive Manufacturing of Bone Scaffolds. Int J Bioprint, 5(1):148. DOI: 10.18063/ijb.v5i1.148.
4. Zhuang P, Sun AX, An J, et al., 2018, 3D Neural Tissue Models: From Spheroids to Bioprinting. Biomaterials, 154:113–33. DOI: 10.1016/j.biomaterials.2017.10.002.
5. Mironov V, Trusk T, Kasyanov V, et al., 2009, Biofabrication: A 21st Century Manufacturing Paradigm. Biofabrication, 1(2):022001. DOI: 10.1088/1758-5082/1/2/022001.
6. Mir TA, Nakamura M, Iwanaga S, et al., 2019, Biofabrication Offers Future Hope for Tackling Various Obstacles and Challenges in Tissue Engineering and Regenerative Medicine: A Perspective, Int J Bioprint, 5(1):153. DOI: 10.18063/ijb.v5i1.153.
7. Ng WL, Chua CK, Shen YF, et al., 2019, Print Me an Organ! Why We are not There Yet. Prog Polym Sci, 97:101145. DOI:10.1016/j.prog-polymsci.2019.101145.
8. Lee JM, Sing SL, Zhou M, et al., 2018, 3D Bioprinting Processes: A Perspective on Classification and Terminology. Int J Bioprint, 4(2):151. DOI: 10.18063/ijb.v4i2.151.
9. Murphy SV, Atala A, 2014, 3D Bioprinting of Tissues and Organs. Nat Biotechnol, 32(8):773–85. DOI: 10.1038/nbt.2958.
10. Du X, 2018, 3D Bio-Printing Review. Mater Sci Eng, 301(1):012023. DOI: 10.1088/1757-899X/301/1/012023.
11. Heinrich MA, Liu W, Jimenez A, et al., 2019, 3D Bioprinting: From Benches to Translational Applications. Small, 15(23):1805510. DOI: 10.1002/smll.201805510.
12. Lee JY, An J, Chua CK, et al., 2017, Fundamentals and Applications of 3D Printing for Novel Materials. Appl Mater Today, 7:120–33. DOI: 10.1016/j.apmt.2017.02.004.
13. Nakamura K, Nishiyama Y, Henmi C, et al., 2008, Ink Jet Three-dimensional Digital Fabrication for Biological Tissue Manufacturing: Analysis of Alginate Microgel Beads Produced by Ink Jet Droplets for Three Dimensional Tissue Fabrication. J Imaging Sci Technol, 52(6):060201. DOI: 10.2352/J.ImagingSci.Technol.(2008)52:6(060201).
14. Arai K, Iwanaga S, Toda H, et al., 2011, Three- Dimensional Inkjet Biofabrication Based on Designed Images. Biofabrication, 3(3):034113. DOI: 10.1088/1758- 5082/3/3/034113.
15. Sorkio A, Koch L, Koivusalo L, et al., 2018, Human Stem Cell Based Corneal Tissue Mimicking Structures Using Laser- Assisted 3D Bioprinting and Functional Bioinks. Biomaterials, 171:57–71. DOI: 10.1016/j.biomaterials.2018.04.034.
16. Kérourédan O, Hakobyan D, Rémy M, et al., 2019, In Situ Prevascularization Designed by Laser-assisted Bioprinting: Effect on Bone Regeneration. Biofabrication, 11(4):045002. DOI: 10.1088/1758-5090/ab2620.
17. Sakai S, Kamei H, Mori T, et al., 2018, Visible Light-Induced Hydrogelaton of an Alginate Derivative and Application to Stereolithographic Bioprinting Using a Visible Light Projector and Acic Red. Biomacromolecules, 19(2):672–9. DOI: 10.1021/acs.biomac.7b01827.
18. Lam T, Dehne T, Krüger JP, et al., 2019, Photopolymerizable Gelatin and Hyaluronic Acid for Stereolithographic 3D Bioprinting of Tissue-Engineered Cartilage. J Biomed Mater Res B, 107(8):2649–57. DOI: 10.1002/jbm.b.34354.
19. Zhuang P, Ng WL, An J, et al., 2019, Layer-By-Layer Ultraviolet Assisted Extrusion-Based (UAE) Bioprinting of Hydrogel Constructs with High Aspect Ratio for Soft Tissue Engineering Applications. Plos One, 14(6):e0216776. DOI: 10.1371/journal.pone.0216776.
20. Placone JK, Engler AJ, 2018, Recent Advances in Extrusion-Based 3D Printing for Biomedical Applications. Adv Healthc Mater, 7(8):e1701161. DOI: 10.1002/adhm.201701161.
21. Tai C, Bouissil S, Gantumur E, et al., 2019, Use of Anionic Polysaccharides in the Development of 3D Bioprinting Technology. Appl Sci, 9(13):2596. DOI: 10.3390/app9132596.
22. Hölzl K, Lin S, Tytgat L, et al., 2016, Bioink Properties Before, During and After 3D Bioprinting. Biofabrication, 8(3):032002. DOI: 10.1088/1758-5090/8/3/032002.
23. Ji S, Guvendiren M, et al., 2017, Recent Advances in Bioink Design for 3D Bioprinting of Tissues and Organs. Front Bioeng Biotechnol, 5:23. DOI: 10.3389/fbioe.2017.00023.
24. Das S, Pati F, Choi YJ, et al., 2015, Bioprintable, Cell-Laden Silk Fibroin-Gelatin Hydrogel Supporting Multilineage Differentiation of Stem Cells for Fabrication of Three- Dimensional Tissue Constructs. Acta Biomater, 11:233–46. DOI: 10.1016/j.actbio.2014.09.023.
25. Sakai S, Yamamoto Y, Enkhtuul G, et al., 2017, Inkjetting Plus Peroxidase-Mediated Hydrogelation Produces Cellladen, Cell-Sized Particles with Suitable Characters for Individual Applications. Macromol Biosci, 17(5):1600416. DOI: 10.1002/mabi.201600416.
26. Arai K, Tsukamoto Y, Yoshida H, et al., 2016, The Development for Cell-Adhesive Hydrogel for 3D Printing. Int J Bioprinting, 2(2):44–53. DOI: 10.18063/IJB.2016.02.002.
27. Sakai S, Ueda K, Gantumur E, et al., 2018, Drop-on-Drop Multimaterial 3D Bioprinting Realized by Peroxidase- Mediated Cross-Linking. Macromol Rapid Commun, 39(3):1700534. DOI: 10.1002/marc.201700534.
28. Sakai S, Mochizuki K, Qu Y, et al., 2018, Peroxidase- Catalyzed Microextrusion Bioprinting of Cell-Laden Hydrogel Constructs in Vaporized Ppm-Level Hydrogen Peroxide. Biofabrication, 10(4):045007. DOI: 10.1088/1758-5090/aadc9e.
29. Gantumur E, Kimura M, Taya M, et al., 2020, Inkjet Micropatterning Through Horseradish Peroxidase-mediated Hydrogelation for Controlled Cell Immobilization and Microtissue Fabrication. Biofabrication, 12(1):011001. DOI: 10.1088/1758-5090/ab3b3c.
30. Sakai S, Nakahata M, et al., 2017, Horseradish Peroxidase Catalyzed Hydrogelation for Biomedical, Biopharmaceutical, and Biofabrication Applications. Chem Asian J, 12(24):3098– 109. DOI: 10.1002/asia.201701364.
31. Moriyama K, Minamihata K, Wakabayashi R, et al., 2014, Enzymatic Preparation of a Redox-Responsive Hydrogel for Encapsulating and Releasing Living Cells. Chem Commun, 50(44):5895–8. DOI: 10.1039/c3cc49766f.
32. Gantumur E, Sakai S, Nakahata M, et al., 2017, Cytocompatible Enzymatic Hydrogelation Mediated by Glucose and Cysteine Residues. ACS Macro Lett, 6(5):485–8. DOI: 10.1021/acsmacrolett.7b00122.
33. Gantumur E, Sakai S, Nakahata M, et al., 2019, Horseradish Peroxidase-Catalyzed Hydrogelation Consuming Enzyme- Produced Hydrogen Peroxide in the Presence of Reducing Sugars. Soft Matter, 15(10):2163–9. DOI: 10.1039/ c8sm01839a.
34. Athukoralalage SS, Balu R, Dutta NK, et al., 2019, 3D Bioprinted Nanocellulose-Based Hydrogels for Tissue Engineering Applications: A Brief Review. Polymers, 11(5):898. DOI: 10.3390/polym11050898.
35. Markstedt K, Mantas A, Tournier I, et al., 2015, 3D Bioprinting Human Chondrocytes with Nanocellulose-Alginate Bioink for Cartilage Tissue Engineering Application. Biomacromol, 16(5):1489–96. DOI: 10.1021/acs.biomac.5b00188.
36. Leppiniemi J, Lahtinen P, Paajanen A, et al., 2017, 3D-Printable Bioactivated Nanocellulose-Alginate Hydrogels. ACS Appl Mater Interfaces, 9(26):21959–70. DOI: 10.1021/acs.ami.7b02756.
37. Ojansivu M, Rashad A, Ahlinder A, et al., 2019, Wood-Based Nanocellulose and Bioactive Glass Modified Gelatin-Alginate Bioinks for 3D Bioprinting of Bone Cells. Biofabrication, 11(3):035010. DOI: 10.1088/1758-5090/ab0692.
38. Sakai S, Kawakami K, et al., 2007, Synthesis and Characterization of Both Ionically and Enzymatically Crosslinkable Alginate. Acta Biomater, 3(4):495–501. DOI: 10.1016/j.actbio.2006.12.002.
39. Sakai S, Hirose K, Taguchi K, et al., 2009, An Injectable, In Situ Enzymatically Gellable, Gelatin Derivative for Drug Delivery and Tissue Engineering. Biomaterials, 30(20):3371‑7. DOI: 10.1016/j.biomaterials.2009.03.030.
40. Nguyen D, Hägg DA, Forsman A, et al., 2017, Cartilage Tissue Engineering by the 3D Bioprinting of iPS Cells in a Nanocellulose/Alginate Bioink. Sci Reports, 7(1):658.DOI: 10.1038/s41598-017-00690-y.
41. Wang L, Xu M, Luo L, et al., 2018, Iterative Feedback Bioprinting-derived Cell-laden Hydrogel Scaffolds with Optimal Geometrical Fidelity and Cellular Controllability. Sci Rep, 8(1):2802. DOI: 10.1038/s41598-018-21274-4.
42. Piras CC, Fernandez-Prieto S, De Borggraeve WM, et al., 2017, Nanocellulosic Materials as Bioinks for 3D Bioprinting. Biomater Sci, 5(10):1988–92. DOI: 10.1039/c7bm00510e.
43. Xu W, Zhang X, Yang P, et al., 2019, Surface Engineered Biomimetic Inks Based on UV Cross-Linkable Wood Biopolymers for 3D Printing. Appl Mater Interfaces, 11(13):12389–400. DOI: 10.1021/acsami.9b03442.
44. Ajdary R, Huan S, Ezazi NZ, et al., 2019, Acetylated Nanocellulose for Single-Component Bioinks and Cell Proliferation on 3D-Printed Scaffolds. Biomacromol, 20(7):2770–8. DOI: 10.1021/acs.biomac.9b00527.
45. Sarker B, Singh R, Silva R, et al., 2014, Evaluation of Fibroblasts Adhesion and Proliferation on Alginate-Gelatin Crosslinked Hydrogel. Plos One, 9(9):e107952. DOI: 10.1371/journal.pone.0107952.