Three-dimensional-printing for microfluidics or the other way around?
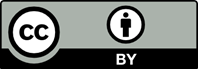
As microfluidic devices are designed to tackle more intricate tasks, the architecture of microfluidic devices becomes more complex, and more sophisticated fabrication techniques are in demand. Therefore, it is sensible to fabricate microfluidic devices by three-dimensional (3D)-printing, which is well-recognized for its unique ability to monolithically fabricate complex structures using a near-net-shape additive manufacturing process. Many 3D-printed microfluidic platforms have been demonstrated but can 3D-printed microfluidics meet the demanding requirements in today’s context, and has microfluidics truly benefited from 3D-printing? In contrast to 3D-printed microfluidics, some go the other way around and exploit microfluidics for 3D-printing. Many innovative printing strategies have been made possible with microfluidicsenabled 3D-printing, although the limitations are also largely evident. In this perspective article, we take a look at the current development in 3D-printed microfluidics and microfluidics-enabled 3D printing with a strong focus on the limitations of the two technologies. More importantly, we attempt to identify the innovations required to overcome these limitations and to develop new high-value applications that would make a scientific and social impact in the future.
1. Whitesides GM, 2006, The Origins and the Future of Microfluidics. Nature, 442(7101):368.
2. Mitchell P, 2001, Microfluidics-downsizing Large-scale Biology. Nat Biotechnol, 19(8):717.
3. Yin H, Marshall D, 2012, Microfluidics for Single Cell Analysis. Curr Opin Biotechnol, 23(1):110-9.
4. Weibel DB, Whitesides GM, 2006, Applications of Microfluidics in Chemical Biology. Curr Opin Chem Biol, 10(6):584-91.
5. Grayson ACR, Shawgo RS, Johnson AM, et al., 2004, A bioMEMS Review: MEMS Technology for Physiologically Integrated Devices. Proc IEEE, 92(1):6-21. DOI 10.1109/ jproc.2003.820534.
6. Ziaie B, Baldi A, Lei M, et al., 2004, Hard and Soft Micromachining for BioMEMS: Review of Techniques and Examples of Applications in Microfluidics and Drug Delivery. Adv Drug Deliv Rev, 56(2):145-72. DOI 10.1016/j. addr.2003.09.001.
7. Shawgo RS, Grayson ACR, Li Y, et al., 2002, BioMEMS for Drug Delivery. Curr Opin Solid State Mater Sci, 6(4):329-34.
8. Tay FE, 2002, Microfluidics and BioMEMS Applications. Berlin, Germany: Springer.
9. Bashir R, 2004, BioMEMS: State-of-the-art in Detection, Opportunities and Prospects. Adv Drug Deliv Rev, 56(11):1565-86. DOI 10.1016/j.addr.2004.03.002.
10. Xia Y, Whitesides GM, 1998, Soft Lithography. Angew Chem Int Ed, 37(5):550-75.
11. Unger MA, Chou HP, Thorsen T, et al., 2000, Monolithic Microfabricated Valves and Pumps by Multilayer Soft Lithography. Science, 288(5463):113-6. DOI 10.1126/ science.288.5463.113.
12. Lecault V, White AK, Singhal A, et al., 2012, Microfluidic Single Cell Analysis: From Promise to Practice. Curr Opin Chem Biol, 16(3-4):381-90.
13. Kim P, Kwon KW, Park MC, et al., 2008, Soft Lithography for Microfluidics: A Review. Biochip J, 2:1-11.
14. Beebe DJ, Mensing GA, Walker GM, 2002, Physics and Applications of Microfluidics in Biology. Ann Rev Biomed Eng, 4(1):261-86.
15. Minteer SD, 2006, Microfluidic Techniques: Reviews and Protocols. Vol. 321. New York: Springer Science and Business Media.
16. Au AK, Huynh W, Horowitz LF, et al., 2016, 3D-printed Microfluidics. Angew Chem Int Ed, 55(12):3862-81. DOI 10.1002/anie.201504382.
17. Yazdi AA, Popma A, Wong W, et al., 2016, 3D Printing: An Emerging Tool for Novel Microfluidics and Lab-ona- chip Applications. Microfluid Nanofluidics, 20(3):50. DOI 10.1007/s10404-016-1715-4.
18. Waheed S, Cabot JM, Macdonald NP, et al., 2016, 3D Printed Microfluidic Devices: Enablers and Barriers. Lab Chip, 16(11):1993-2013. DOI 10.1039/c6lc00284f.
19. Ho CMB, Ng SH, Li KHH, et al., 2015, 3D Printed Microfluidics for Biological Applications. Lab Chip,
15(18):3627-37. DOI 10.1039/c5lc00685f.
20. Au AK, Bhattacharjee N, Horowitz LF, et al., 2015, 3D-printed Microfluidic Automation. Lab Chip, 15(8):1934-41. DOI 10.1039/c5lc00126a.
21. Bhattacharjee N, Urrios A, Kang S, et al., 2016, The Upcoming 3D-printing Revolution in Microfluidics. Lab Chip, 16(10):1720-42. DOI 10.1039/c6lc00163g.
22. Donvito L, Galluccio L, Lombardo A, et al., 2015, Experimental Validation of a Simple, Low-cost, T-junction Droplet Generator Fabricated Through 3D Printing. J Micromech Microeng, 25(3):035013. DOI 10.1088/0960- 1317/25/3/035013.
23. Chen C, Wang Y, Lockwood SY, et al., 2014, 3D-printed Fluidic Devices Enable Quantitative Evaluation of Blood Components in Modified Storage Solutions for Use in Transfusion Medicine. Analyst, 139(13):3219-26. DOI 10.1039/c3an02357e.
24. Kitson PJ, Rosnes MH, Sans V, et al., 2012, Configurable 3D-Printed Millifluidic and Microfluidic ‘Lab on a Chip’reactionware Devices. Lab Chip, 12(18):3267-71. DOI 10.1039/c2lc40761b.
25. Bishop GW, Satterwhite JE, Bhakta S, et al., 2015, 3D-printed Fluidic Devices for Nanoparticle Preparation and Flow injection Amperometry Using Integrated Prussian Blue Nanoparticle-modified Electrodes. Anal Chem, 87(10):5437-43. DOI 10.1021/acs.analchem.5b00903.
26. Takenaga S, Schneider B, Erbay E, et al., 2015, Fabrication of Biocompatible Lab-on-chip Devices for Biomedical Applications by Means of a 3D-printing Process. Physica Status Solidi A, 212(6):1347-52. DOI 10.1002/ pssa.201532053.
27. Lee W, Kwon D, Choi W, et al., 2015, 3D-printed Microfluidic Device for the Detection of Pathogenic Bacteria using Sizebased Separation in Helical Channel with Trapezoid Crosssection. Sci Rep, 5:7717. DOI 10.1038/srep09701.
28. Shallan AI, Smejkal P, Corban M, et al., 2014, Costeffective Three-dimensional Printing of Visibly Transparent Microchips Within Minutes. Anal Chem, 86(6):3124-30. DOI 10.1021/ac4041857.
29. Monaghan T, Harding MJ, Harris RA, et al., 2016, Customisable 3D Printed Microfluidics for Integrated Analysis and Optimisation. Lab Chip, 16(17):3362-73. DOI 10.1039/c6lc00562d.
30. Cabot JM, Fuguet E, Rosés M, et al., 2015, Novel Instrument for Automated p K a Determination by Internal Standard Capillary Electrophoresis. Anal Chem, 87(12):6165-72. DOI 10.1021/acs.analchem.5b00845.
31. Gelber MK, Bhargava R, 2015, Monolithic Multilayer Microfluidics via Sacrificial Molding of 3D-printed Isomalt. Lab Chip, 15(7):1736-41. DOI 10.1039/c4lc01392a.
32. Anderson KB, Lockwood SY, Martin RS, et al., 2013, A 3D Printed Fluidic Device that Enables Integrated Features. Anal Chem, 85(12):5622-6. DOI 10.1021/ac4009594.
33. Au AK, Lee W, Folch A, 2014, Mail-order Microfluidics: Evaluation of Stereolithography for the Production of Microfluidic Devices. Lab Chip, 14(7):1294-301. DOI 10.1039/c3lc51360b.
34. Gong H, Woolley AT, Nordin GP, 2016, High Density 3D Printed Microfluidic Valves, Pumps, and Multiplexers. Lab Chip, 16(13):2450-8. DOI 10.1039/c6lc00565a.
35. Rogers CI, Qaderi K, Woolley AT, et al., 2015, 3D Printed Microfluidic Devices with Integrated Valves. Biomicrofluidics, 9(1):016501. DOI 10.1063/1.4905840.
36. Keating SJ, Gariboldi MI, Patrick WG, et al., 2016, 3D Printed Multimaterial Microfluidic Valve. PLoS One, 11(8):e0160624. DOI 10.1371/journal.pone.0160624.
37. Sochol R, Sweet E, Glick C, et al., 2016, 3D Printed Microfluidic Circuitry via Multijet-based Additive Manufacturing. Lab Chip, 16(4):668-78. DOI 10.1039/ c5lc01389e.
38. Chen Y, Chan HN, Michael SA, et al., 2017, A Microfluidic Circulatory System Integrated with Capillary-assisted Pressure Sensors. Lab Chip, 17(4):653-62. DOI 10.1039/ c6lc01427e.
39. Bhargava KC, Thompson B, Malmstadt N, 2014, Discrete Elements for 3D Microfluidics. Proc Natl Acad Sci, 111(42):15013-8. DOI 10.1073/pnas.1414764111.
40. Lee KG, Park KJ, Seok S, et al., 2014, 3D Printed Modules for Integrated Microfluidic Devices. RSC Adv, 4(62):32876-80. DOI 10.1039/c4ra05072j.
41. Vittayarukskul K, Lee AP, 2017, A Truly Lego®-like Modular Microfluidics Platform. J Micromech Microeng, 27(3):035004. DOI 10.1088/1361-6439/aa53ed.
42. Kirk CG, 1961, Toy Building Brick. Google Patents.
43. Yuen PK, 2016, A Reconfigurable Stick-n-play Modular Microfluidic System using Magnetic Interconnects. Lab Chip, 16(19):3700-7. DOI 10.1039/c6lc00741d.
44. Tumbleston JR, Shirvanyants D, Ermoshkin N, et al., 2015, Continuous Liquid Interface Production of 3D Objects. Science, 347(6228):1349-52. DOI 10.1126/science.aaa2397.
45. Beauchamp MJ, Nordin GP, Woolley AT, 2017, Moving from Millifluidic to Truly Microfluidic sub-100-μm Cross-section 3D Printed Devices. Anal Bioanal Chem, 409(18):4311-9. DOI 10.1007/s00216-017-0398-3.
46. Mazutis L, Gilbert J, Ung WL, et al., 2013, Single-cell Analysis and Sorting using Droplet-based Microfluidics. Nat Protoc, 8(5):870. DOI 10.1038/nprot.2013.046.
47. ASIGA. Available from: https://www.asiga.com/products/ printers/pico. [Last retrieved on 2019 Jun 10].
48. Lee JM, Zhang M, Yeong WY, 2016, Characterization and Evaluation of 3D Printed Microfluidic Chip for Cell Processing. Microfluid Nanofluidics, 20(1):5. DOI 10.1007/ s10404-015-1688-8.
49. Li F, Macdonald NP, Guijt RM, et al., 2019, Increasing the Functionalities of 3D Printed Microchemical Devices by Single Material, Multimaterial, and Print-pause-print 3D Printing. Lab Chip, 19(1):35-49. DOI 10.1039/c8lc00826d.
50. Colosi C, Shin SR, Manoharan V, et al., 2016, Microfluidic Bioprinting of Heterogeneous 3D Tissue Constructs using Low-viscosity Bioink. Adv Mater, 28(4):677-84. DOI 10.1002/adma.201503310.
51. Serex L, Bertsch A, Renaud P, 2018, Microfluidics: A New Layer of Control for Extrusion-based 3D Printing. Micromachines, 9(2):86. DOI 10.3390/mi9020086.
52. Hansen CJ, Saksena R, Kolesky DB, et al., 2013, High throughput Printing via Microvascular Multinozzle Arrays. Adv Mater, 25(1):96-102. DOI 10.1002/adma.201370002.
53. Ozawa F, Okitsu T, Takeuchi S, 2017, Improvement in the Mechanical Properties of Cell-laden Hydrogel Microfibers using Interpenetrating Polymer Networks. ACS Biomater Sci Eng, 3(3):392-8. DOI 10.1021/acsbiomaterials.6b00619.
54. Gao Q, He Y, Fu JZ, et al., 2015, Coaxial Nozzle-assisted 3D Bioprinting with Built-in Microchannels for Nutrients Delivery. Biomaterials, 61:203-15. DOI 10.1016/j. biomaterials.2015.05.031.
55. Colosi C, Costantini M, Latini R, et al., 2014, Rapid Prototyping of Chitosan-coated Alginate Scaffolds through the use of a 3D Fiber Deposition Technique. J Mater Chem B, 2(39):6779-91. DOI 10.1039/c4tb00732h.
56. Gao Q, Liu Z, Lin Z, et al., 2017, 3D Bioprinting of Vessel-like Structures with Multilevel Fluidic Channels. ACS Biomater Sci Eng, 3(3):399-408. DOI 10.1021/ acsbiomaterials.6b00643.
57. Attalla R, Ling C, Selvaganapathy P, 2016, Fabrication and Characterization of Gels with Integrated Channels using 3D Printing with Microfluidic Nozzle for Tissue Engineering Applications. Biomed Microdevices, 18(1):17. DOI 10.1007/ s10544-016-0042-6.
58. Ghorbanian S, Qasaimeh MA, Akbari M, et al., 2014, Microfluidic Direct Writer with Integrated Declogging Mechanism for Fabricating Cell-laden Hydrogel Constructs. Biomed Microdevices, 16(3):387-95. DOI 10.1007/s10544- 014-9842-8.
59. Hardin JO, Ober TJ, Valentine AD, et al., 2015, Microfluidic Printheads for Multimaterial 3D Printing of Viscoelastic Inks. Adv Mater, 27(21):3279-84. DOI 10.1002/adma.201570145.
60. Wei D, Sun J, Bolderson J, et al., 2017, Continuous Fabrication and Assembly of Spatial Cell-laden Fibers for a Tissue-like Construct via a Photolithographic-based Microfluidic Chip. ACS Appl Mater Interfaces, 9(17):14606-17. DOI 10.1021/acsami.7b00078.
61. Leng L, McAllister A, Zhang B, et al., 2012, Mosaic Hydrogels: One-step Formation of Multiscale Soft Materials. Adv Mater, 24(27):3650-8. DOI 10.1002/adma.201290166.
62. Ober TJ, Foresti D, Lewis JA, 2015, Active Mixing of Complex Fluids at the Microscale. Proc Natl Acad Sci, 112(40):12293-8. DOI 10.1073/pnas.1509224112.
63. Collino RR, Ray TR, Fleming RC, et al., 2016, Deposition of Ordered Two-phase Materials using Microfluidic Print Nozzles with Acoustic Focusing. Extreme Mech Lett, 8:96- 106. DOI 10.1016/j.eml.2016.04.003.
64. Li X, Zhang JM, Yi X, et al., 2018, Multimaterial Microfluidic 3D Printing of Textured Composites with Liquid Inclusions. Adv Sci, 6:1800730. DOI 10.1002/advs.201800730.
65. Visser CW, Kamperman T, Karbaat LP, et al., 2018, In-air Microfluidics Enables Rapid Fabrication of Emulsions, Suspensions, and 3D Modular (bio) Materials. Sci Adv, 4(1):eaao1175. DOI 10.1126/sciadv.aao1175.