Optimization of a 3D bioprinting process using ultrashort peptide bioinks
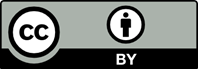
The field of three-dimensional (3D) bioprinting is rapidly emerging as an additive manufacturing method for tissue and organ fabrication. The demand for tissues and organ transplants is ever increasing, although donors are not as readily available. Consequently, tissue engineering is gaining much attention to alleviate this problem. The process of achieving well-structured 3D bioprinted constructs using hydrogel bioinks depends on symmetrical precision, regulated flow rates, and viability of cells. Even with the mentioned parameters optimized, the printed structures need additional refining by removing excessive liquids, as peptide hydrogel bioprints encapsulate water. However, it is challenging to eliminate the confined fluids without compromising the printing process. In this paper, we introduced a vacuum system to our 3D bioprinting robotic arm and thus optimized the printing quality for complex and refined 3D scaffolds. Moreover, the proposed vacuum system supports printing with cells. Our results show improved printing resolution which facilitates the printing of higher and more stable structures.
1. Chua C, Yeong W. Bioprinting. New Jersey: World Scientific; 2015. https://doi.org/10.1142/9193.
2. Organdonor.gov. Organ Donation Statistics. Organ Donor; 2018. Available from: https://www.organdonor.gov/statistics-stories/statistics.html. [Last accessed on 2018 Oct 30].
3. Sundaramurthi D, Rauf S, Hauser C, 2016, 3D bioprinting technology for regenerative medicine applications. Int J Bioprint, 2: 9–26. https://doi.org/10.18063/IJB.2016.02.010.
4. Fermeiro J, Calado M, Correia I, 2015, State of the Art and Challenges in Bioprinting Technologies, Contribution of the 3D Bioprinting in Tissue Engineering. 2015 IEEE 4th Portuguese Meeting on Bioengineering.
5. Choudhury D, Anand S, Naing M, 2018, The arrival of commercial bioprinters-towards 3D bioprinting revolution! Int J Bioprint, 4: 139. https://doi.org/10.18063/ijb.v4i2.139.
6. Mironov V, Kasyanov V, Markwald R, 2011, Organ printing: From bioprinter to organ biofabrication line. Curr Opin Biotechnol, 22: 667–673. https://doi.org/10.1016/j. copbio.2011.02.006.
7. Derakhshanfar S, Mbeleck R, Xu K, et al., 2018, 3D bioprinting for biomedical devices and tissue engineering: A review of recent trends and advances. Bioact Mater, 3: 144–156. https://doi.org/10.1016/j.bioactmat.2017.11.008.
8. Arab W, Rauf S, Al-Harbi O, et al., 2018, Novel ultrashort self-assembling peptide bioinks for 3D culture of muscle myoblast cells. Int J Bioprint, 4: 129. https://doi.org/10.18063/ijb.v4i1.129.
9. Costa R, Rauf S, Hauser C, 2017, Towards biologically relevant synthetic designer matrices in 3D bioprinting for tissue engineering and regenerative medicine. Curr Opin Biomed Eng, 2: 90–98. https://doi.org/10.1016/j. cobme.2017.05.001.
10. Hauser C, Deng R, Mishra A, et al., 2011, Natural tri- to hexapeptides self-assemble in water to amyloid-type fiber aggregates by unexpected-helical intermediate structures. Proc Natl Acad Sci, 108: 1361–1366. https://doi.org/10.1073/ pnas.1014796108.