Of balls, inks and cages: Hybrid biofabrication of 3D tissue analogs
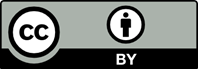
The overarching principle of three-dimensional (3D) bioprinting is the placing of cells or cell clusters in the 3D space to generate a cohesive tissue microarchitecture that comes close to in vivo characteristics. To achieve this goal, several technical solutions are available, generating considerable combinatorial bandwidth: (i) Support structures are generated first, and cells are seeded subsequently; (ii) alternatively, cells are delivered in a printing medium, so-called “bioink,” that contains them during the printing process and ensures shape fidelity of the generated structure; and (iii) a “scaffold-free” version of bioprinting, where only cells are used and the extracellular matrix is produced by the cells themselves, also recently entered a phase of accelerated development and successful applications. However, the scaffold-free approaches may still benefit from secondary incorporation of scaffolding materials, thus expanding their versatility. Reversibly, the bioink-based bioprinting could also be improved by adopting some of the principles and practices of scaffold-free biofabrication. Collectively, we anticipate that combinations of these complementary methods in a “hybrid” approach, rather than their development in separate technological niches, will largely increase their efficiency and applicability in tissue engineering
1. Moroni L, Boland T, Burdick J A, et al., 2017, Biofabrication: A guide to technology and terminology. Trends Biotechnol, 36(4): 384-402. https://doi.org/10.1016/j.tibtech.2017.10.015.
2. Atala A, Yoo, J, 2015, Essentials of 3D Biofabrication and Translation. Amsterdam: Elsevier.
3. Mironov V, 2005, The second international workshop on bioprinting, biopatterning and bioassembly. Expert Opin Biol Ther, 5(8): 1111-1115. https://doi. org/10.1517/14712598.5.8.1111.
4. Groll J, Boland T, Blunk T, et al., 2016, Biofabrication: Reappraising the definition of an evolving field. Biofabrication, 8(1): 13001. https://doi.org/10.1088/1758- 5090/aaec52.
5. Yu Y, Zhang Y, Ozbolat IT, 2016, Three-dimensional bioprinting using self-assembling scalable scaffold-free “tissue strands” as a new bioink. Sci Rep, 6: 28714. https:// doi.org/10.1038/srep28714.
6. Takahashi H, Okano T, 2015, Cell sheet-based tissue engineering for organizing anisotropic tissue constructs produced using microfabricated thermoresponsive substrates. Adv Healthc Mater, 4(16): 2388-2407. https://doi. org/10.1002/adhm.201500194.
7. Moldovan N I, Hibino N, Nakayama K, 2017, Principles of the kenzan method for robotic cell spheroid-based three-dimensional bioprinting. Tissue Eng Part B Rev, 23(3): 237- 244. https://doi.org/10.1089/ten.teb.2016.0322.
8. Ovsianikov A, Khademhosseini A, Mironov V, 2018, The synergy of scaffold-based and scaffold-free tissue engineering strategies. Trends Biotechnol, 36(4): 348-357. https://doi. org/10.1016/j.tibtech.2018.01.005.
9. Moldovan L, 2017, Comparison of biomaterial-dependent and independent bioprinting methods for cardiovascular medicine. Curr Opin Biomed Eng, 2: 124-131. https://doi. org/10.1016/j.cobme.2017.05.009.
10. Zhu W, Ma X, Gou M, et al., 2016, 3D printing of functional biomaterials for tissue engineering. Curr Opin Biotechnol, 40: 103-112. https://doi.org/10.1016/j.copbio.2016.03.014.
11. Muylaert D E, Fledderus J O, Bouten C V, et al., 2014, Combining tissue repair and tissue engineering; bioactivating implantable cell-free vascular scaffolds. Heart, 100(23): 1825-1830. https://doi.org/10.1136/heartjnl-2014-306092.
12. Jeong, C.G., Atala A, 2015, 3D printing and biofabrication for load bearing tissue engineering. Adv Exp Med Biol, 881: 3-14. https://doi.org/10.1007/978-3-319-22345-2_1.
13. Banu A, Tatara A M, Sutradhar A, 2018, Large animal models of an in vivo bioreactor for engineering vascularized bone. Tissue Eng Part B, 24(4): 317-325. https://doi.org/10.1089/ ten.teb.2018.0005.
14. Ho S S, Murphy K C, Binder B Y, et al., 2016, Increased survival and function of mesenchymal stem cell spheroids entrapped in instructive alginate hydrogels. Stem Cells Transl Med, 5(6): 773-781. https://doi.org/10.5966/sctm.2015-0211.
15. Skardal A, Mack D, Kapetanovic E, et al., 2012, Bioprinted amniotic fluid-derived stem cells accelerate healing of large skin wounds. Stem Cells Transl Med, 1(11): 792-802. https:// doi.org/10.5966/sctm.2012-0088.
16. Laternser S, Keller H, Leupin O, et al., 2018, A novel microplate 3D bioprinting platform for the engineering of muscle and tendon tissues. Slas Technol, 3: 2472630318776594. https:// doi.org/10.1177/2472630318776594.
17. Rapoport S, 2013, Electrospinning tubular scaffolds with tissue-like mechanical properties and biomimetic surface features. Methods Mol Biol, 1001: 153-165. https://doi. org/10.1007/978-1-62703-363-3_13.
18. Udelsman B, Hibino N, Villalona G A, et al., 2011, Development of an operator-independent method for seeding tissue-engineered vascular grafts. Tissue Eng Part C. Methods, 17(7): 731-736. https://doi.org/10.1089/ten. tec.2010.0581.
19. Fu W J, Xu Y D, Wang Z X, et al., 2012, New ureteral scaffold constructed with composite poly(L-lactic acid)-collagen and urothelial cells by new centrifugal seeding system. J Biomed Mater Res A, 100(7): 1725-1733. https://doi.org/10.1002/ jbm.a.34134.
20. Thomas J, Jones D, Moldovan L, et al., 2018., Labeling of endothelial cells with magnetic microbeads by angiophagy. Biotechnol Lett, 2018. http//:doi: 10.1007/s10529-018-2581-9.
21. Murphy S V, Atala A, 2014, 3D bioprinting of tissues and organs. Nat Biotechnol, 32(8): 773-785. https://doi. org/10.1038/nbt.2958.
22. Murphy S V, Skardal A, Atala A, 2013, Evaluation of hydrogels for bio-printing applications. J Biomed Mater Res A, 101(1): 272-284. https://doi.org/10.1002/jbm.a.34326.
23. Boland T, Xu T, Damon B, et al., 2006, Application of inkjet printing to tissue engineering. Biotechnol J, 1(9): 910-917. https://doi.org/10.1002/biot.200600081.
24. Pirlo R K, Dean D, Knapp D R, et al., 2006, Cell deposition system based on laser guidance. Biotechnol J, 1(9): 1007- 1013. https://doi.org/10.1002/biot.200600127.
25. Xiong R, Zhang Z, Chai W, et al., 2015, Freeform drop-on-demand laser printing of 3D alginate and cellular constructs. Biofabrication, 7(4): 45011. https://doi.org/10.1088/1758- 5090/7/4/045011.
26. Hospodiuk M, Dey M, Sosnoski D, et al., 2017, The bioink: A comprehensive review on bioprintable materials. Biotechnol Adv, 35(2): 217-239. https://doi.org/10.1016/j. biotechadv.2016.12.006.
27. Pourchet L J, Thepot A, Albouy M, et al., 2017, Human skin 3D bioprinting using scaffold-free approach. Adv Healthc Mater, 6(4):345. https://doi.org/10.1002/adhm.201601101.
28. Kolesky D B, Homan K A, Skylar-Scott M A, et al., 2016, Three-dimensional bioprinting of thick vascularized tissues. Proc Natl Acad Sci U S A, 113(12): 3179-3184. https://doi. org/10.1073/pnas.1521342113.
29. Lee S J, Lim G J, Lee J W, et al., 2006, In vitro evaluation of a poly(lactide-co-glycolide)–collagen composite scaffold for bone regeneration. Biomaterials, 27(18): 3466-3472. https:// doi.org/10.1016/j.biomaterials.2006.01.059.
30. Kang H W, Lee S J, Ko I K, et al., 2016, A 3D bioprinting system to produce human-scale tissue constructs with structural integrity. Nat Biotechnol, 34(3): 312-319. https:// doi.org/10.1038/nbt.3413.
31. Xu T, Binder K W, Albanna M Z, et al., 2013, Hybrid printing of mechanically and biologically improved constructs for cartilage tissue engineering applications. Biofabrication, 5(1): 15001. https://doi.org/10.1088/1758-5082/5/1/015001.
32. Kikuchi T, Shimizu T, Wada M, et al., 2014, Automatic fabrication of 3-dimensional tissues using cell sheet manipulator technique. Biomaterials, 35(8): 2428-2435. https://doi.org/10.1016/j.biomaterials.2013.12.014.
33. Kim K, Utoh R, Ohashi K, et al., 2015, Fabrication of functional 3D hepatic tissues with polarized hepatocytes by stacking endothelial cell sheets in vitro. J Tissue Eng Regen Med, 2015: 124-126.
34. Leung B M, Sefton M V, 2010, A modular approach to cardiac tissue engineering. Tissue Eng Part A, 16(10): 3207-3218. https://doi.org/10.1089/ten.tea.2009.0746.
35. Owaki T, Shimizu T, Yamato M, et al., 2014, Cell sheet engineering for regenerative medicine: Current challenges and strategies. Biotechnol J, 9(7): 904-914. https://doi. org/10.1002/biot.201300432.
36. Gartner Z J, Bertozzi C R, 2017, Programmed assembly of 3-dimensional microtissues with defined cellular connectivity. Proc Natl Acad Sci U S A, 106(12): 4606-4610. https://doi. org/10.1073/pnas.0900717106.
37. Bhang S H, Cho S W, La W G, et al., 2011, Angiogenesis in ischemic tissue produced by spheroid grafting of human adipose-derived stromal cells. Biomaterials, 32(11): 2734- 2747. https://doi.org/10.1016/j.biomaterials.2010.12.035.
38. Haji-Karim M, Carlsson J, 1978, Proliferation and viability in cellular spheroids of human origin. Cancer Res, 38(5): 1457-1464.
39. Takebe T, Enomura M, Yoshizawa E, et al., 2015, Vascularized and complex organ buds from diverse tissues via mesenchymal cell-driven condensation. Cell Stem Cell, 16(5): 556-565. https://doi.org/10.1016/j.stem.2015.03.004.
40. Shafiee A, 2015, Post-deposition bioink self-assembly: A quantitative study. Biofabrication, 7(4): 45005. https://doi. org/10.1088/1758-5090/7/4/045005.
41. Mironov V, Visconti R P, Kasyanov V, et al., 2009, Organ printing: Tissue spheroids as building blocks. Biomaterials, 30(12): 2164-2174. https://doi.org/10.1016/j. biomaterials.2008.12.084.
42. Bao B, Jiang J, Yanase T, et al., 2011, Connexon-mediated cell adhesion drives microtissue self-assembly. FASEB J, 25(1): 255-264. https://doi.org/10.1096/fj.10-155291.
43. Dean D M, Morgan J R, 2008, Cytoskeletal-mediated tension modulates the directed self-assembly of microtissues. Tissue Eng Part A, 14(12): 1989-1997. https://doi.org/10.1089/ten. tea.2007.0320.
44. Thomas G L, Nagy-Mehez V, Mombach A, 2014, Dynamics of cell aggregates fusion: Experiments and simulations. Physica, 395: 247-254. https://doi.org/10.1016/j.physa.2013.10.037.
45. Izaguirre J A, Chaturvedi R, Huang C, et al., 2004, Compucell, a multi-model framework for simulation of morphogenesis. Bioinformatics, 20(7): 1129-1137. https://doi.org/10.1093/ bioinformatics/bth050.
46. Sego T J, Kasacheuski U, Hauersperger D, et al., 2017, A heuristic computational model of basic cellular processes and oxygenation during spheroid-dependent biofabrication. Biofabrication, 9(2): 24104. https://doi.org/10.1088/1758- 5090/aa6ed4.
47. Gobaa S, Hoehnel S, Roccio M, et al., 2011, Artificial niche microarrays for probing single stem cell fate in high throughput. Nat Methods, 8(11): 949-955. https://doi. org/10.1038/nmeth.1732.
48. Ungrin M D, Joshi C, Nica A, et al., 2008, Reproducible, ultra high-throughput formation of multicellular organization from single cell suspension-derived human embryonic stem cell aggregates. PLoS One, 3(2): e1565. https://doi.org/10.1371/ journal.pone.0001565.
49. Murata D, Tokunaga S, Tamura T, et al., 2015, A preliminary study of osteochondral regeneration using a scaffold-free three-dimensional construct of porcine adipose tissue-derived mesenchymal stem cells. J Orthop Surg Res, 10: 35. https:// doi.org/10.1186/s13018-015-0173-0.
50. Noguchi R, Nakayama K, Itoh M, et al., 2016 Development of a three-dimensional pre-vascularized scaffold-free contractile cardiac patch for treating heart disease. J Heart Lung Transplant, 35(1): 137-145. https://doi.org/10.1016/j. healun.2015.06.001.
51. Tseng H, Gage J A, Shen T, et al., 2016, A high-throughput in vitro ring assay for vasoactivity using magnetic 3D bioprinting. Sci Rep, 6: 30640.
52. Jaganathan H, Gage J, Leonard F, et al., 2014, Three-dimensional in vitro co-culture model of breast tumor using magnetic levitation. Sci Rep, 4: 6468.
53. Dean D M, Sasagawa T, Shimizu T, et al., 2007, Rods, tori, and honeycombs: The directed self-assembly of microtissues with prescribed microscale geometries. FASEB J, 21(14): 4005-4012. https://doi.org/10.1096/fj.07-8710com.
54. Bulanova E A, Koudan E V, Degosserie J, et al., 2017, Bioprinting of a functional vascularized mouse thyroid gland construct. Biofabrication, 9(3): 34105. https://doi. org/10.1088/1758-5090/aa7fdd.
55. Jakab K, Damon B, Neagu A, et al., 2006, Three-dimensional tissue constructs built by bioprinting. Biorheology, 43(3-4): 509-513.
56. Lee W, 2012, Cellular hydrogel biopaper for patterned 3D cell culture and modular tissue reconstruction. Adv Healthc Mater, 1(5): 635-639. https://doi.org/10.1002/adhm.201290023.
57. Hakam M S, Imani R, Abolfathi N, et al., 2016, Evaluation of fibrin-gelatin hydrogel as biopaper for application in skin bioprinting: An in-vitro study. Biomed Mater Eng, 27(6): 669-682. https://doi.org/10.3233/BME-161617.
58. Olsen T R, Mattix B, Casco M, et al., 2015, Manipulation of cellular spheroid composition and the effects on vascular tissue fusion. Acta Biomater, 13: 188-198. https://doi. org/10.1016/j.actbio.2014.11.024.
59. Nguyen D G, Funk J, Robbins J B, et al., 2016, Bioprinted 3D primary liver tissues allow assessment of organ-level response to clinical drug induced toxicity in vitro. PLoS One, 11(7): e0158674. https://doi.org/10.1371/journal.pone.0158674.
60. Shimoto T, 2012, Building of HD MACs using cell processing robot for cartilage regeneration. J Robot Mechatr, 24(2): 347- 353. https://doi.org/10.20965/jrm.2012.p0347.
61. Itoh M, Nakayama K, Noguchi R, et al., 2015, Scaffold-free tubular tissues created by a bio-3D printer undergo remodeling and endothelialization when implanted in rat aortae. PLoS One, 10(9): e0136681. https://doi.org/10.1371/ journal.pone.0136681.
62. Machino R, Taniguchi D, Takeoka Y, et al., 2015, Scaffold-free trachea tissue engineering using bioprinting. Am J Respir Crit Care Med, 191: A5343.
63. Zhang X Y, Yanagi Y, Takeoka Y, et al., 2018, Regeneration of diaphragm with bio-3D cellular patch. Biomaterials, 167: 1-14. https://doi.org/10.1016/j.biomaterials.2018.03.055.
64. Mironov V, Kasyanov V, Drake C, et al., 2008, Organ printing: Promises and challenges. Regen Med, 3(1): 93-103. https://doi.org/10.2217/17460751.3.1.93.
65. Collin de H A, Takeishi K, Guzman-Lepe J, et al., 2016, Liver-regenerative transplantation: Regrow and reset. Am J Transplant, 16(6): 1688-1696. https://doi.org/10.1111/ ajt.13678.
66. Yipeng J, Yongde X, Yuanyi W, et al., 2017, Microtissues enhance smooth muscle differentiation and cell viability of hADSCs for three dimensional bioprinting. Front Physiol, 8: 534. https://doi.org/10.3389/fphys.2017.00534.
67. Williams S K, Touroo J S, Church K H, et al., 2013, Encapsulation of adipose stromal vascular fraction cells in alginate hydrogel spheroids using a direct-write three-dimensional printing system. Biores Open Access, 2(6): 448- 454. https://doi.org/10.1089/biores.2013.0046.
68. Gettler B C, Zakhari J S, Gandhi P S, et al., 2017, Formation of adipose stromal vascular fraction cell-laden spheroids using a three-dimensional bioprinter and superhydrophobic surfaces. Tissue Eng Part C., 23(9): 516-524. https://doi. org/10.1089/ten.tec.2017.0056.
69. Izadifar Z, Chang T, Kulyk W, et al., 2016, Analyzing biological performance of 3D-printed, cell-impregnated hybrid constructs for cartilage tissue engineering. Tissue Eng Part C. Methods, 22(3): 173-188. https://doi.org/10.1089/ten.tec.2015.0307.
70. Castilho M, Feyen D, Flandes-Iparraguirre M, et al. 2017, Melt electrospinning writing of poly-hydroxymethylglycolide-co-epsilon-caprolactone-based scaffolds for cardiac tissue engineering. Adv Healthc Mater, 2017: 6-18.
71. Danilevicius P, Rezende R A, Pereira F D, et al., 2015, Burr-like, laser-made 3D microscaffolds for tissue spheroid encagement. Biointerphases, 10(2): 21011. https://doi. org/10.1116/1.4922646.
72. Ahmad T, Lee J, Shin Y M, et al., 2017, Hybrid-spheroids incorporating ECM like engineered fragmented fibers potentiate stem cell function by improved cell/cell and cell/ ECM interactions. Acta Biomater, 64: 161-175. https://doi. org/10.1016/j.actbio.2017.10.022.
73. Lareu R R, Arsianti I, Subramhanya H K, et al., 2007, In vitro enhancement of collagen matrix formation and crosslinking for applications in tissue engineering: A preliminary study. Tissue Eng, 13(2): 385-391. https://doi.org/10.1089/ ten.2006.0224.
74. Satyam A, Kumar P, Fan X, et al., 2014 Macromolecular crowding meets tissue engineering by self-assembly: A paradigm shift in regenerative medicine. Adv Mater, 26(19): 3024-3034. https://doi.org/10.1002/adma.201304428.
75. Chen C, Loe F, Blocki A, et al., 2011, Applying macromolecular crowding to enhance extracellular matrix deposition and its remodeling in vitro for tissue engineering and cell-based therapies. Adv Drug Deliv Rev, 63(4-5): 277- 290. https://doi.org/10.1016/j.addr.2011.03.003.
76. Dewavrin J Y, Hamzavi N, Shim V P, et al., 2014, Tuning the architecture of three-dimensional collagen hydrogels by physiological macromolecular crowding. Acta Biomater, 10(10): 4351-4359. https://doi.org/10.1016/j.actbio.2014.06.006.
77. Rashid R, Lim N S, Chee S M, et al., 2014, Novel use for polyvinylpyrrolidone as a macromolecular crowder for enhanced extracellular matrix deposition and cell proliferation. Tissue Eng Part C. Methods, 20(12): 994-1002. https://doi.org/10.1089/ten.tec.2013.0733.
78. Magno V, Friedrichs J, Weber H M, et al., 2017 Macromolecular crowding for tailoring tissue.-derived fibrillated matrices. Acta Biomater, 55: 109-119. https://doi. org/10.1016/j.actbio.2017.04.018.
79. Leung B M, Lesher-Perez S C, Matsuoka T, et al., 2015, Media additives to promote spheroid circularity and compactness in hanging drop platform. Biomater Sci, 3(2): 336-344. https:// doi.org/10.1039/C4BM00319E
80. Ng W L, Goh M H, Yeong W Y, et al., 2018, Applying macromolecular crowding to 3D bioprinting: Fabrication of 3D hierarchical porous collagen-based hydrogel constructs. Biomater Sci, 6(3): 562-574. https://doi.org/10.1039/ C7BM01015J.