Concentric bioprinting of alginate-based tubular constructs using multi-nozzle extrusion-based technique
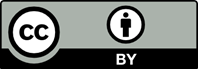
Bioprinting is a layer-by-layer additive fabrication technique for making three-dimensional (3D) tissue and organ constructs using biological products. The capability to fabricate 3D tubular structure in free-form or vertical configuration is the first step towards the possibility of organ printing in three dimensions. In this study, alginate-based tubular structures of varying viscosity were printed vertically using multi-nozzle extrusion-based technique. Manufacturing challenges associated with the vertical printing configurations are also discussed here. We have also proposed measurable parameters to quantify the quality of printing for systematic investigation in bioprinting. This study lays a foundation for the successful fabrication of viable 3D tubular constructs.
[1] P. Zorlutuna, N. Hasirci, and V. Hasirci, "Nanopatterned collagen tubes for vascular tissue engineering," Journal of Tissue Engineering and Regenerative Medicine, vol. 2, pp. 373-377, 2008.
[2] A. Hasan, A. Memic, N. Annabi, M. Hossain, A. Paul, M. R. Dokmeci, et al., "Electrospun scaffolds for tissue engineering of vascular grafts," Acta Biomaterialia, vol. 10, pp. 11-25, 2014.
[3] D. G. Seifu, A. Purnama, K. Mequanint, and D. Mantovani, "Small-diameter vascular tissue engineering," Nat Rev Cardiol, vol. 10, pp. 410-421, 2013.
[4] R. A. Penkala and S. S. Kim, "Gastrointestinal tissue engineering," Expert Review of Medical Devices, vol. 4, pp. 65-72, 2007.
[5] K. N. Bitar and S. Raghavan, "Intestinal Tissue Engineering: Current Concepts and Future Vision of Regenerative Medicine in the Gut," Neurogastroenterology and motility : the official journal of the European Gastrointestinal Motility Society, vol. 24, pp. 7-19, 2012.
[6] S. Ramakrishna, K. Fujihara, W.-E. Teo, T. Yong, Z. Ma, and R. Ramaseshan, "Electrospun nanofibers: solving global issues," Materials Today, vol. 9, pp. 40-50, 2006.
[7] S. Chung, N. P. Ingle, G. A. Montero, S. H. Kim, and M. W. King, "Bioresorbable elastomeric vascular tissue engineering scaffolds via melt spinning and electrospinning," Acta Biomaterialia, vol. 6, pp. 1958-1967, 2010.
[8] L. Cui, N. Zhang, W. Cui, P. Zhang, and X. Chen, "A Novel Nano/Micro-Fibrous Scaffold by Melt-Spinning Method for Bone Tissue Engineering," Journal of Bionic Engineering, vol. 12, pp. 117-128, 2015.
[9] J. An, C. Chua, K. Leong, C.-H. Chen, and J.-P. Chen, "Solvent-free fabrication of three dimensionally aligned polycaprolactone microfibers for engineering of anisotropic tissues," Biomedical Microdevices, vol. 14, pp. 863-872, 2012.
[10] S. Harding, A. Afoke, R. Brown, A. MacLeod, P. Shamlou, and P. Dunnill, "Engineering and cell attachment properties of human fibronectin–fibrinogen scaffolds for use in tissue engineered blood vessels," Bioprocess and Biosystems Engineering, vol. 25, pp. 53-59, 2002.
[11] D. Motlagh, J. Yang, K. Y. Lui, A. R. Webb, and G. A. Ameer, "Hemocompatibility evaluation of poly (glycerol-sebacate) in vitro for vascular tissue engineering," Biomaterials, vol. 27, pp. 4315-4324, 2006.
[12] C. Stamm, A. Khosravi, N. Grabow, K. Schmohl, N. Treckmann, A. Drechsel, et al., "Biomatrix/polymer composite material for heart valve tissue engineering," The Annals of thoracic surgery, vol. 78, pp. 2084-2093, 2004.
[13] W. Jeong, J. Kim, S. Kim, S. Lee, G. Mensing, and D. J. Beebe, "Hydrodynamic microfabrication via "on the fly" photopolymerization of microscale fibers and tubes," Lab on a Chip, vol. 4, pp. 576-580, 2004.
[14] Z. Sun, E. Zussman, A. L. Yarin, J. H. Wendorff, and A. Greiner, "Compound Core–Shell Polymer Nanofibers by Co-Electrospinning," Advanced Materials, vol. 15, pp. 1929-1932, 2003.
[15] R. Suntornnond, J. An, W. Y. Yeong, and C. K. Chua, "Biodegradable Polymeric Films and Membranes Processing and Forming for Tissue Engineering," Macromolecular Materials and Engineering, pp. n/a-n/a, 2015.
[16] F. Yang, C. G. Williams, D.-a. Wang, H. Lee, P. N. Manson, and J. Elisseeff, "The effect of incorporating RGD adhesive peptide in polyethylene glycol diacrylate hydrogel on osteogenesis of bone marrow stromal cells," Biomaterials, vol. 26, pp. 5991-5998, 2005.
[17] F. Wang, Y. Li, Y. Shen, A. Wang, S. Wang, and T. Xie, "The functions and applications of RGD in tumor therapy and tissue engineering," International journal of molecular sciences, vol. 14, pp. 13447-13462, 2013.
[18] L. G. Griffith, "Polymeric biomaterials," Acta Materialia, vol. 48, pp. 263-277, 2000.
[19] Y. Onuki, U. Bhardwaj, F. Papadimitrakopoulos, and D. J. Burgess, "A Review of the Biocompatibility of Implantable Devices: Current Challenges to Overcome Foreign Body Response," Journal of diabetes science and technology (Online), vol. 2, pp. 1003-1015, 2008.
[20] L. Gui, L. Zhao, R. W. Spencer, A. Burghouwt, M. S. Taylor, S. W. Shalaby, et al., "Development of Novel Biodegradable Polymer Scaffolds for Vascular Tissue Engineering," Tissue Engineering. Part A, vol. 17, pp. 1191-1200, 2011.
[21] R. Midha, C. A. Munro, P. D. Dalton, C. H. Tator, and M. S. Shoichet, "Growth factor enhancement of peripheral nerve regeneration through a novel synthetic hydrogel tube," Journal of neurosurgery, vol. 99, pp. 555-565, 2003.
[22] T. Freier, R. Montenegro, H. S. Koh, and M. S. Shoichet, "Chitin-based tubes for tissue engineering in the nervous system," Biomaterials, vol. 26, pp. 4624-4632, 2005.
[23] P. D. Dalton, L. Flynn, and M. S. Shoichet, "Manufacture of poly (2-hydroxyethyl methacrylate-co-methyl methacrylate) hydrogel tubes for use as nerve guidance channels," Biomaterials, vol. 23, pp. 3843-3851, 2002.
[24] V. Mironov, V. Kasyanov, X. Z. Shu, C. Eisenberg, L. Eisenberg, S. Gonda, et al., "Fabrication of tubular tissue constructs by centrifugal casting of cells suspended in an in situ crosslinkable hyaluronan-gelatin hydrogel," Biomaterials, vol. 26, pp. 7628-7635, 2005.
[25] B. G. Chung, K.-H. Lee, A. Khademhosseini, and S.-H. Lee, "Microfluidic fabrication of microengineered hydrogels and their application in tissue engineering," Lab on a Chip, vol. 12, pp. 45-59, 2012.
[26] S. Sakai, Y. Liu, E. J. Mah, and M. Taya, "Horseradish peroxidase/catalase-mediated cell-laden alginate-based hydrogel tube production in two-phase coaxial flow of aqueous solutions for filament-like tissues fabrication," Biofabrication, vol. 5, p. 015012, 2013.
[27] L. Zhang, Z. Cao, T. Bai, L. Carr, J.-R. Ella-Menye, C. Irvin, et al., "Zwitterionic hydrogels implanted in mice resist the foreign-body reaction," Nat Biotech, vol. 31, pp. 553-556, 06//print 2013.
[28] C. Xu, W. Chai, Y. Huang, and R. R. Markwald, "Scaffold‐free inkjet printing of three‐dimensional zigzag cellular tubes," Biotechnology and bioengineering, vol. 109, pp. 3152-3160, 2012.
[29] C. Norotte, F. S. Marga, L. E. Niklason, and G. Forgacs, "Scaffold-free vascular tissue engineering using bioprinting," Biomaterials, vol. 30, pp. 5910-5917, 2009.
[30] W. Y. Y. Chee Kai Chua, "Introduction to Tissue Engineering," in Bioprinting: Principles and Application, W. Y. Y. Chee Kai Chua, Ed., ed: World Scientific, 2015, pp. 1-15.
[31] A. Skardal, J. Zhang, and G. D. Prestwich, "Bioprinting vessel-like constructs using hyaluronan hydrogels crosslinked with tetrahedral polyethylene glycol tetracrylates," Biomaterials, vol. 31, pp. 6173-6181, 8// 2010.
[32] Y. Nishiyama, M. Nakamura, C. Henmi, K. Yamaguchi, S. Mochizuki, H. Nakagawa, et al., "Development of a Three-Dimensional Bioprinter: Construction of Cell Supporting Structures Using Hydrogel and State-Of-The-Art Inkjet Technology," Journal of Biomechanical Engineering, vol. 131, pp. 035001-035001, 2008.
[33] V. Jetze, P. Benjamin, J. B. Thijs, B. Jelle, J. A. D. Wouter, P. W. M. Ferry, et al., "Biofabrication of multi-material anatomically shaped tissue constructs," Biofabrication, vol. 5, p. 035007, 2013.
[34] R. Zwanzig and A. K. Harrison, "Modifications of the Stokes–Einstein formula," The Journal of Chemical Physics, vol. 83, pp. 5861-5862, 1985.
[35] B. Katzbauer, "Properties and applications of xanthan gum," Polymer Degradation and Stability, vol. 59, pp. 81-84, 1998.
[36] F. Garcıa-Ochoa, V. Santos, J. Casas, and E. Gomez, "Xanthan gum: production, recovery, and properties," Biotechnology advances, vol. 18, pp. 549-579, 2000.
[37] K. Shimada, K. Fujikawa, K. Yahara, and T. Nakamura, "Antioxidative properties of xanthan on the autoxidation of soybean oil in cyclodextrin emulsion," Journal of agricultural and food chemistry, vol. 40, pp. 945-948, 1992.
[38] W. F. Rencher, "Biocompatible drug delivery gel containing mixture of sodium carboxymethyl cellulose, xanthan gum and/or alginates, diluent," ed: Google Patents, 1995.
[39] W. F. Rencher, "Bioadhesive pharmaceutical carrier," ed: Google Patents, 1993.
[40] J. M. Lee and W. Y. Yeong, "A preliminary model of time-pressure dispensing system for bioprinting based on printing and material parameters," Virtual and Physical Prototyping, vol. 10, pp. 3-8, 2014.
[41] A. Mikkelsen and A. Elgsaeter, "Density distribution of calcium-induced alginate gels. A numerical study," Biopolymers, vol. 36, pp. 17-41, 1995.