Hydrogel-based materials for mandibular reconstruction
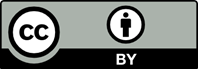
Mandibular reconstruction remains a significant clinical challenge due to irregular defect geometries, high functional restoration requirements, and complex oral environments. Traditional vascularized bone grafts, while effective, are limited by donor site complications and poor osseointegration. Hydrogel-based materials have emerged as promising alternatives due to their biocompatibility, tunable mechanical properties, and capacity to mimic the extracellular matrix for osteogenesis and angiogenesis. This review highlights recent advances in hydrogel design strategies tailored for mandibular regeneration. Key considerations include mechanical reinforcement through nanocomposites and dual-network architectures, which enhance compressive strength and toughness to withstand masticatory loads. Injectable hydrogels demonstrate minimally invasive delivery and shape adaptability for irregular defects, while biomimetic wet adhesives achieve robust tissue integration through covalent and coordination bonding mechanisms. Functionalization with bioactive factors and stem cells promotes spatiotemporal regulation of osteogenesis and angiogenesis, as evidenced by successful mandibular regeneration in preclinical models. Antibacterial strategies integrating metal ions, antibacterial agents, or peptides can contribute to addressing oral microbial challenges. This review underscores the potential of multifunctional hydrogels to bridge structural and functional regeneration in craniofacial reconstruction while identifying critical research gaps for future innovation.

- Hayden RE, Mullin DP, Patel AK. Reconstruction of the segmental mandibular defect: Current state of the art. Curr Opin Otolaryngol Head Neck Surg. 2012;20(4):231-236. doi: 10.1097/MOO.0b013e328355d0f3
- Paré A, Bossard A, Laure B, Weiss P, Gauthier O, Corre P. Reconstruction of segmental mandibular defects: Current procedures and perspectives. Laryngoscope Investig Otolaryngol. 2019;4(6):587-596. doi: 10.1002/lio2.325
- Al Maruf DSA, Ghosh YA, Xin H, et al. Hydrogel: A potential material for bone tissue engineering repairing the segmental mandibular defect. Polymers (Basel). 2022;14(19):4186. doi: 10.3390/polym14194186
- Zhou B, Jiang X, Zhou X, et al. GelMA-based bioactive hydrogel scaffolds with multiple bone defect repair functions: therapeutic strategies and recent advances. Biomater Res. 2023;27(1):86. doi: 10.1186/s40824-023-00422-6
- Kumar A, Rao KM, Han SS. Synthesis of mechanically stiff and bioactive hybrid hydrogels for bone tissue engineering applications. Chem Eng J. 2017;317:119-131. doi: 10.1016/j.cej.2017.02.065
- Bai X, Gao M, Syed S, Zhuang J, Xu X, Zhang XQ. Bioactive hydrogels for bone regeneration. Bioact Mater. 2018;3(4):401-417. doi: 10.1016/j.bioactmat.2018.05.006
- Yue S, He H, Li B, Hou T. Hydrogel as a biomaterial for bone tissue engineering: A review. Nanomaterials (Basel). 2020;10(8):1511. doi: 10.3390/nano10081511
- Al Maruf DSA, Parthasarathi K, Cheng K, et al. Current and future perspectives on biomaterials for segmental mandibular defect repair. Int J Polym Mater. 2022;72(9):725-737. doi: 10.1080/00914037.2022.2052729
- Spiller KL, Maher SA, Lowman AM. Hydrogels for the repair of articular cartilage defects. Tissue Eng Part B Rev. 2011;17(4):281-299. doi: 10.1089/ten.TEB.2011.0077
- Bashir S, Hina M, Iqbal J, et al. Fundamental concepts of hydrogels: Synthesis, properties, and their applications. Polymers (Basel). 2020;12(11):2702. doi: 10.3390/polym12112702
- Cheng C, Sun Q, Wang X, He B, Jiang T. Enzyme-manipulated hydrogelation of small molecules for biomedical applications. Acta Biomater. 2022;151:88-105. doi: 10.1016/j.actbio.2022.08.016
- Li Y, Wang X, Han Y, Sun HY, Hilborn J, Shi L. Click chemistry-based biopolymeric hydrogels for regenerative medicine. Biomed Mater. 2021;16(2):022003. doi: 10.1088/1748-605X/abc0b3
- Zhang Y, Wang S, Tian Y, et al. Multi-physically cross-linked hydrogels for flexible sensors with high strength and self-healing properties. Polymers (Basel). 2023;15(18):3748. doi: 10.3390/polym15183748
- Hu W, Wang Z, Xiao Y, Zhang S, Wang J. Advances in crosslinking strategies of biomedical hydrogels. Biomater Sci. 2019;7(3):843-855. doi: 10.1039/c8bm01246f
- Salma-Ancane K, Sceglovs A, Tracuma E, et al. Effect of crosslinking strategy on the biological, antibacterial and physicochemical performance of hyaluronic acid and e-polylysine based hydrogels. Int J Biol Macromol. 2022;208:995-1008. doi: 10.1016/j.ijbiomac.2022.03.207
- Niewiadomski K, Szopa D, Pstrowska K, Wróbel P, Witek- Krowiak A. Comparative analysis of crosslinking methods and their impact on the physicochemical properties of SA/ PVA hydrogels. Materials (Basel). 2024;17(8):1816. doi: 10.3390/ma17081816
- Chuang CH, Lin RZ, Melero-Martin JM, Chen YC. Comparison of covalently and physically cross-linked collagen hydrogels on mediating vascular network formation for engineering adipose tissue. Artif Cells Nanomed Biotechnol. 2018;46(sup3):S434-S447. doi: 10.1080/21691401.2018.1499660
- El-Husseiny HM, Mady EA, El-Dakroury WA, Doghish AS, Tanaka R. Stimuli-responsive hydrogels: Smart state of-the-art platforms for cardiac tissue engineering. Front Bioeng Biotechnol. 2023;11:1174075. doi: 10.3389/fbioe.2023.1174075
- Aghajanzadeh MS, Imani R, Nazarpak MH, McInnes SJP. Augmented physical, mechanical, and cellular responsiveness of gelatin-aldehyde modified xanthan hydrogel through incorporation of silicon nanoparticles for bone tissue engineering. Int J Biol Macromol. 2024;259(Pt 2):129231. doi: 10.1016/j.ijbiomac.2024.129231
- Rauner N, Meuris M, Zoric M, Tiller JC. Enzymatic mineralization generates ultrastiff and tough hydrogels with tunable mechanics. Nature. 2017;543(7645):407-410. doi: 10.1038/nature21392
- Yu T, Zhang L, Dou X, et al. Mechanically robust hydrogels facilitating bone regeneration through epigenetic modulation. Adv Sci (Weinh). 2022;9(32):e2203734. doi: 10.1002/advs.202203734
- Wu P, Shen L, Liu HF, et al. The marriage of immunomodulatory, angiogenic, and osteogenic capabilities in a piezoelectric hydrogel tissue engineering scaffold for military medicine. Mil Med Res. 2023;10(1):35. doi: 10.1186/s40779-023-00469-5
- Li W, Wang C, Wang Z, et al. Physically cross-linked DNA hydrogel-based sustained cytokine delivery for in situ diabetic alveolar bone rebuilding. ACS Appl Mater Interfaces. 2022;14(22):25173-25182. doi: 10.1021/acsami.2c04769
- Takallu S, Mirzaei E, Azadi A, Karimizade A, Tavakol S. Plate-shape carbonated hydroxyapatite/collagen nanocomposite hydrogel via in situ mineralization of hydroxyapatite concurrent with gelation of collagen at pH = 7.4 and 37°C. J Biomed Mater Res B Appl Biomater. 2019;107(6):1920-1929. doi: 10.1002/jbm.b.34284
- Gwak GH, Choi AJ, Bae YS, Choi HJ, Oh JM. Electrophoretically prepared hybrid materials for biopolymer hydrogel and layered ceramic nanoparticles. Biomater Res. 2016;20:1. doi: 10.1186/s40824-016-0048-4
- Shin SR, Jung SM, Zalabany M, et al. Carbon-nanotube-embedded hydrogel sheets for engineering cardiac constructs and bioactuators. ACS Nano. 2013;7(3):2369-2380. doi: 10.1021/nn305559j
- Kayalvizhy E, Pazhanisamy P. Swelling behavior of poly(N-cyclohexylacrylamide-co-acrylamide/AMPSNa) gold nanocomposite hydrogels. Int J Biol Macromol. 2016;86:721-727. doi: 10.1016/j.ijbiomac.2016.01.047
- Kumar A, Jaiswal M. Design and in vitro investigation of nanocomposite hydrogel based in situ spray dressing for chronic wounds and synthesis of silver nanoparticles using green chemistry. J Appl Polym Sci. 2015;133(14):43260. doi: 10.1002/app.43260
- Thoniyot P, Tan MJ, Karim AA, Young DJ, Loh XJ. Nanoparticle-hydrogel composites: Concept, design, and applications of these promising, multi-functional materials. Adv Sci (Weinh). 2015;2(1-2):1400010. doi: 10.1002/advs.201400010
- Li Z, Mi W, Wang H, Su Y, He C. Nano-hydroxyapatite/ polyacrylamide composite hydrogels with high mechanical strengths and cell adhesion properties. Colloids Surf B Biointerfaces. 2014;123:959-964. doi: 10.1016/j.colsurfb.2014.10.050
- Guo J, Yao H, Chang L, et al. Magnesium nanocomposite hydrogel reverses the pathologies to enhance mandible regeneration. Adv Mater. 2025;37(2):e2312920. doi: 10.1002/adma.202312920
- Matricardi P, Di Meo C, Coviello T, Hennink WE, Alhaique F. Interpenetrating polymer networks polysaccharide hydrogels for drug delivery and tissue engineering. Adv Drug Deliv Rev. 2013;65(9):1172-1187. doi: 10.1016/j.addr.2013.04.002
- Zhang G, Wang X, Meng G, et al. Enzyme-mineralized PVASA hydrogels with combined toughness and strength for bone tissue engineering. ACS Appl Mater Interfaces. 2024;16(1):178-189. doi: 10.1021/acsami.3c14006
- Li G, Gao F, Yang D, et al. ECM-mimicking composite hydrogel for accelerated vascularized bone regeneration. Bioact Mater. 2024;42:241-256. doi: 10.1016/j.bioactmat.2024.08.035
- Zhang X, Huang P, Jiang G, et al. A novel magnesium ion-incorporating dual-crosslinked hydrogel to improve bone scaffold-mediated osteogenesis and angiogenesis. Mater Sci Eng C Mater Biol Appl. 2021;121:111868. doi: 10.1016/j.msec.2021.111868
- Zhou X, Sun J, Wo K, et al. nHA-loaded gelatin/ alginate hydrogel with combined physical and bioactive features for maxillofacial bone repair. Carbohydr Polym. 2022;298:120127. doi: 10.1016/j.carbpol.2022.120127
- Wu Y, Li X, Sun Y, et al. Multiscale design of stiffening and ROS scavenging hydrogels for the augmentation of mandibular bone regeneration. Bioact Mater. 2023;20:111-125. doi: 10.1016/j.bioactmat.2022.05.021
- Qiao M, Xu Z, Pei X, et al. Nano SIM@ZIF-8 modified injectable High-intensity biohydrogel with bidirectional regulation of osteogenesis and Anti-adipogenesis for bone repair. Chem Eng J. 2022;434:134583. doi: 10.1016/j.cej.2022.134583
- Zheng A, Wang X, Xin X, et al. Promoting lacunar bone regeneration with an injectable hydrogel adaptive to the microenvironment. Bioact Mater. 2023;21:403-421. doi: 10.1016/j.bioactmat.2022.08.031
- Tang Z, Zhao M, Wang Y, et al. Mussel-inspired cellulose-based adhesive with biocompatibility and strong mechanical strength via metal coordination. Int J Biol Macromol. 2020;144:127-134. doi: 10.1016/j.ijbiomac.2019.12.076
- Hu S, Wang S, He Q, et al. A mechanically reinforced super bone glue makes a leap in hard tissue strong adhesion and augmented bone regeneration. Adv Sci (Weinh). 2023;10(11):e2206450. doi: 10.1002/advs.202206450
- Yang R, Chen B, Zhang X, Bao Z, Yan Q, Luan S. Degradable nanohydroxyapatite-reinforced superglue for rapid bone fixation and promoted osteogenesis. ACS Nano. 2024;18(11):8517-8530. doi: 10.1021/acsnano.4c01214
- Yang G, Li Y, Zhang S, et al. Double-cross-linked hydrogel with long-lasting underwater adhesion: Enhancement of maxillofacial in situ and onlay bone retention. ACS Appl Mater Interfaces. 2023;15(40):46639-46654. doi: 10.1021/acsami.3c09117
- Deng H, Dong A, Song J, Chen X. Injectable thermosensitive hydrogel systems based on functional PEG/PCL block polymer for local drug delivery. J Control Release. 2019;297:60-70. doi: 10.1016/j.jconrel.2019.01.026
- Wang W, Shi Y, Lin G, et al. Advances in mechanical properties of hydrogels for cartilage tissue defect repair. Macromol Biosci. 2023;23(7):e2200539. doi: 10.1002/mabi.202200539
- Ghandforoushan P, Alehosseini M, Golafshan N, et al. Injectable hydrogels for cartilage and bone tissue regeneration: A review. Int J Biol Macromol. 2023;246:125674. doi: 10.1016/j.ijbiomac.2023.125674
- Zhou L, Fan L, Zhang FM, et al. Hybrid gelatin/oxidized chondroitin sulfate hydrogels incorporating bioactive glass nanoparticles with enhanced mechanical properties, mineralization, and osteogenic differentiation. Bioact Mater. 2021;6(3):890-904. doi: 10.1016/j.bioactmat.2020.09.012
- Zhao F, Yang Z, Xiong H, Yan Y, Chen X, Shao L. A bioactive glass functional hydrogel enhances bone augmentation via synergistic angiogenesis, self-swelling and osteogenesis. Bioact Mater. 2023;22:201-210. doi: 10.1016/j.bioactmat.2022.09.007
- Bai X, Lü S, Cao Z, et al. Self-reinforcing injectable hydrogel with both high water content and mechanical strength for bone repair. Chem Eng J. 2016;288:546-556. doi: 10.1016/j.cej.2015.12.021
- Baker JL, Mark Welch JL, Kauffman KM, McLean JS, He X. The oral microbiome: Diversity, biogeography and human health. Nat Rev Microbiol. 2024;22(2):89-104. doi: 10.1038/s41579-023-00963-6
- Dewhirst FE, Chen T, Izard J, et al. The human oral microbiome. J Bacteriol. 2010;192(19):5002-5017. doi: 10.1128/jb.00542-10
- Belibasakis GN, Manoil D. Microbial community-driven etiopathogenesis of peri-implantitis. J Dent Res. 2021;100(1):21-28. doi: 10.1177/0022034520949851
- Fonder MA, Lazarus GS, Cowan DA, Aronson-Cook B, Kohli AR, Mamelak AJ. Treating the chronic wound: A practical approach to the care of nonhealing wounds and wound care dressings. J Am Acad Dermatol. 2008;58(2):185-206. doi: 10.1016/j.jaad.2007.08.048
- Werner S, Grose R. Regulation of wound healing by growth factors and cytokines. Physiol Rev. 2003;83(3):835-870. doi: 10.1152/physrev.2003.83.3.835
- Schreml S, Szeimies RM, Prantl L, Karrer S, Landthaler M, Babilas P. Oxygen in acute and chronic wound healing. Br J Dermatol. 2010;163(2):257-268. doi: 10.1111/j.1365-2133.2010.09804.x
- Bako J, Toth F, Gall J, et al. Combined release of antiseptic and antibiotic drugs from visible light polymerized biodegradable nanocomposite hydrogels for periodontitis treatment. Pharmaceutics. 2022;14(5):957. doi: 10.3390/pharmaceutics14050957
- Hu Z, Zhou Y, Wu H, et al. An injectable photopolymerizable chitosan hydrogel doped anti-inflammatory peptide for long-lasting periodontal pocket delivery and periodontitis therapy. Int J Biol Macromol. 2023;252:126060. doi: 10.1016/j.ijbiomac.2023.126060
- Chen X, Huang H, Guo C, et al. Controlling alveolar bone loss by hydrogel‐based mitigation of oral dysbiosis and bacteria‐triggered proinflammatory immune response. Adv Funct Mater. 2024;35(3):2409121. doi: 10.1002/adfm.202409121
- Liu L, Xiang Y, Wang Z, et al. Adhesive liposomes loaded onto an injectable, self-healing and antibacterial hydrogel for promoting bone reconstruction. NPG Asia Mater. 2019;11(1):81. doi: 10.1038/s41427-019-0185-z
- Yang K, Han Q, Chen B, et al. Antimicrobial hydrogels: Promising materials for medical application. Int J Nanomedicine. 2018;13:2217-2263. doi: 10.2147/IJN.S154748
- Eltawila AM, Hassan MN, Safaan SM, et al. Local treatment of experimental mandibular osteomyelitis with an injectable biomimetic gentamicin hydrogel using a new rabbit model. J Biomed Mater Res B Appl Biomater. 2021;109(11):1677-1688. doi: 10.1002/jbm.b.34824
- Xu C, Cao Y, Lei C, et al. Polymer-mesoporous silica nanoparticle core-shell nanofibers as a dual-drug-delivery system for guided tissue regeneration. ACS Appl Nano Mater. 2020;3(2):1457-1467. doi: 10.1021/acsanm.9b02298
- Miao Y, Shi X, Li Q, et al. Engineering natural matrices with black phosphorus nanosheets to generate multi-functional therapeutic nanocomposite hydrogels. Biomater Sci. 2019;7(10):4046-4059. doi: 10.1039/c9bm01072f
- Huja SS, Fernandez SA, Hill KJ, Li Y. Remodeling dynamics in the alveolar process in skeletally mature dogs. Anat Rec A Discov Mol Cell Evol Biol. 2006;288(12):1243-1249. doi: 10.1002/ar.a.20396
- Parsaee F, Alizadeh A, Rezaee M, Alavi O, Alipour H. Evaluation of the osteoconductive properties of scaffold containing platete-enriched-fibrin (PRF) with three calcium phosphate (TCP) in the alveolar socket repair after tooth extraction: An animal study. J Biomater Appl. 2023;37(10):1789-1800. doi: 10.1177/08853282231170346
- Vedadghavami A, Minooei F, Mohammadi MH, et al. Manufacturing of hydrogel biomaterials with controlled mechanical properties for tissue engineering applications. Acta Biomater. 2017;62:42-63. doi: 10.1016/j.actbio.2017.07.028
- Yanagawa F, Sugiura S, Kanamori T. Hydrogel microfabrication technology toward three dimensional tissue engineering. Regen Ther. 2016;3:45-57. doi: 10.1016/j.reth.2016.02.007
- Li J, Wu C, Chu PK, Gelinsky M. 3D printing of hydrogels: Rational design strategies and emerging biomedical applications. Mater Sci Eng R Rep. 2020;140:100543. doi: 10.1016/j.mser.2020.100543
- Bittner SM, Guo JL, Melchiorri A, Mikos AG. Three-dimensional printing of multilayered tissue engineering scaffolds. Mater Today (Kidlington). 2018;21(8):861-874. doi: 10.1016/j.mattod.2018.02.006
- Du M, Chen B, Meng Q, et al. 3D bioprinting of BMSC-laden methacrylamide gelatin scaffolds with CBD-BMP2- collagen microfibers. Biofabrication. 2015;7(4):044104. doi: 10.1088/1758-5090/7/4/044104
- Catros S, Guillemot F, Nandakumar A, et al. Layer-by-layer tissue microfabrication supports cell proliferation in vitro and in vivo. Tissue Eng Part C Methods. 2012;18(1):62-70. doi: 10.1089/ten.TEC.2011.0382
- Gruene M, Deiwick A, Koch L, et al. Laser printing of stem cells for biofabrication of scaffold-free autologous grafts. Tissue Eng Part C Methods. 2011;17(1):79-87. doi: 10.1089/ten.TEC.2010.0359
- Gao G, Yonezawa T, Hubbell K, Dai G, Cui X. Inkjet-bioprinted acrylated peptides and PEG hydrogel with human mesenchymal stem cells promote robust bone and cartilage formation with minimal printhead clogging. Biotechnol J. 2015;10(10):1568-1577. doi: 10.1002/biot.201400635
- Gao G, Schilling AF, Hubbell K, et al. Improved properties of bone and cartilage tissue from 3D inkjet-bioprinted human mesenchymal stem cells by simultaneous deposition and photocrosslinking in PEG-GelMA. Biotechnol Lett. 2015;37(11):2349-2355. doi: 10.1007/s10529-015-1921-2
- Schuurman W, Levett PA, Pot MW, et al. Gelatin-methacrylamide hydrogels as potential biomaterials for fabrication of tissue-engineered cartilage constructs. Macromol Biosci. 2013;13(5):551-561. doi: 10.1002/mabi.201200471
- Levato R, Visser J, Planell JA, Engel E, Malda J, Mateos- Timoneda MA. Biofabrication of tissue constructs by 3D bioprinting of cell-laden microcarriers. Biofabrication. 2014;6(3):035020. doi: 10.1088/1758-5082/6/3/035020
- Tarassoli SP, Jessop ZM, Jovic T, Hawkins K, Whitaker IS. Candidate bioinks for extrusion 3D bioprinting-a systematic review of the literature. Front Bioeng Biotechnol. 2021;9:616753. doi: 10.3389/fbioe.2021.616753
- Fedorovich NE, De Wijn JR, Verbout AJ, Alblas J, Dhert WJ. Three-dimensional fiber deposition of cell-laden, viable, patterned constructs for bone tissue printing. Tissue Eng Part A. 2008;14(1):127-133. doi: 10.1089/ten.a.2007.0158
- Gaspar VM, Lavrador P, Borges J, Oliveira MB, Mano JF. Advanced bottom-up engineering of living architectures. Adv Mater. 2020;32(6):e1903975. doi: 10.1002/adma.201903975
- Zheng F, Xiao Y, Liu H, Fan Y, Dao M. Patient-specific organoid and organ-on-a-chip: 3D cell-culture meets 3D printing and numerical simulation. Adv Biol (Weinh). 2021;5(6):e2000024. doi: 10.1002/adbi.202000024
- Zhang W, Shi W, Wu S, et al. 3D printed composite scaffolds with dual small molecule delivery for mandibular bone regeneration. Biofabrication. 2020;12(3):035020. doi: 10.1088/1758-5090/ab906e
- Shi Y, Shi J, Sun Y, et al. A hierarchical 3D graft printed with nanoink for functional craniofacial bone restoration. Adv Funct Mater. 2023;33(40):2301099. doi: 10.1002/adfm.202301099
- Amler AK, Thomas A, Tuzuner S, et al. 3D bioprinting of tissue-specific osteoblasts and endothelial cells to model the human jawbone. Sci Rep. 2021;11(1):4876. doi: 10.1038/s41598-021-84483-4
- Kuss MA, Harms R, Wu S, et al. Short-term hypoxic preconditioning promotes prevascularization in 3D bioprinted bone constructs with stromal vascular fraction derived cells. RSC Adv. 2017;7(47):29312-29320. doi: 10.1039/c7ra04372d
- Zhang H, Sun H, Zhang L, et al. Coaxial 3D printing scaffolds with sequential antibacterial and osteogenic functions to effectively repair infected mandibular defects. Adv Funct Mater. 2024;34:2407483. doi: 10.1002/adfm.202407483
- Wu C, Zhou Y, Chang J, Xiao Y. Delivery of dimethyloxallyl glycine in mesoporous bioactive glass scaffolds to improve angiogenesis and osteogenesis of human bone marrow stromal cells. Acta Biomater. 2013;9(11):9159-9168. doi: 10.1016/j.actbio.2013.06.026
- Ding H, Gao YS, Wang Y, Hu C, Sun Y, Zhang C. Dimethyloxaloylglycine increases the bone healing capacity of adipose-derived stem cells by promoting osteogenic differentiation and angiogenic potential. Stem Cells Dev. 2014;23(9):990-1000. doi: 10.1089/scd.2013.0486
- Genetos DC, Toupadakis CA, Raheja LF, et al. Hypoxia decreases sclerostin expression and increases Wnt signaling in osteoblasts. J Cell Biochem. 2010;110(2):457-467. doi: 10.1002/jcb.22559
- Qu ZH, Zhang XL, Tang TT, Dai KR. Promotion of osteogenesis through beta-catenin signaling by desferrioxamine. Biochem Biophys Res Commun. 2008; 370(2):332-337. doi: 10.1016/j.bbrc.2008.03.092
- Yao Q, Liu Y, Tao J, Baumgarten KM, Sun H. Hypoxia-mimicking nanofibrous scaffolds promote endogenous bone regeneration. ACS Appl Mater Interfaces. 2016; 8(47):32450-32459. doi: 10.1021/acsami.6b10538
- Zheng X, Zhang X, Wang Y, et al. Hypoxia-mimicking 3D bioglass-nanoclay scaffolds promote endogenous bone regeneration. Bioact Mater. 2021;6(10):3485-3495. doi: 10.1016/j.bioactmat.2021.03.011
- Zhang W, Li G, Deng R, Deng L, Qiu S. New bone formation in a true bone ceramic scaffold loaded with desferrioxamine in the treatment of segmental bone defect: A preliminary study. J Orthop Sci. 2012;17(3):289-298. doi: 10.1007/s00776-012-0206-z
- Stewart R, Goldstein J, Eberhardt A, Chu GT, Gilbert S. Increasing vascularity to improve healing of a segmental defect of the rat femur. J Orthop Trauma. 2011;25(8):472-476. doi: 10.1097/BOT.0b013e31822588d8
- Ning Z, Tan B, Chen B, et al. Precisely controlled delivery of abaloparatide through injectable hydrogel to promote bone regeneration. Macromol Biosci. 2019;19(6):e1900020. doi: 10.1002/mabi.201900020
- Greenblatt MB, Shim JH, Bok S, Kim JM. The extracellular signal-regulated kinase mitogen-activated protein kinase pathway in osteoblasts. J Bone Metab. 2022;29(1):1-15. doi: 10.11005/jbm.2022.29.1.1
- Greenblatt MB, Shim JH, Glimcher LH. Mitogen-activated protein kinase pathways in osteoblasts. Annu Rev Cell Dev Biol. 2013;29:63-79. doi: 10.1146/annurev-cellbio-101512-122347
- Zheng W, Gu X, Sun X, Wu Q, Dan H. FAK mediates BMP9- induced osteogenic differentiation via Wnt and MAPK signaling pathway in synovial mesenchymal stem cells. Artif Cells Nanomed Biotechnol. 2019;47(1):2641-2649. doi: 10.1080/21691401.2019.1631838
- Gehart H, Kumpf S, Ittner A, Ricci R. MAPK signalling in cellular metabolism: Stress or wellness? EMBO Rep. 2010;11(11):834-840. doi: 10.1038/embor.2010.160
- Sun Y, Liu WZ, Liu T, Feng X, Yang N, Zhou HF. Signaling pathway of MAPK/ERK in cell proliferation, differentiation, migration, senescence and apoptosis. J Recept Signal Transduct Res. 2015;35(6):600-604. doi: 10.3109/10799893.2015.1030412
- Yue J, López JM. Understanding MAPK signaling pathways in apoptosis. Int J Mol Sci. 2020;21(7):2346. doi: 10.3390/ijms21072346
- Peng S, Zhou G, Luk KD, et al. Strontium promotes osteogenic differentiation of mesenchymal stem cells through the Ras/MAPK signaling pathway. Cell Physiol Biochem. 2009;23(1-3):165-174. doi: 10.1159/000204105
- Yu X, Wang X, Li D, et al. Mechanically reinforced injectable bioactive nanocomposite hydrogels for in-situ bone regeneration. Chem Eng J. 2022;433:132799. doi: 10.1016/j.cej.2021.132799