High-precision depth-controlled laser bioprinting of cells in extracellular matrix for three-dimensional structures
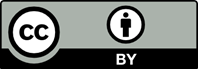
Bioprinting is an emerging additive manufacturing process that offers great potential for fabricating living tissue by precisely printing cells and biomaterials onto various substrates. This technique can imitate native tissue functions, enabling clinical trials to explore new pathways for regenerative medicine. Among various bioprinting techniques, laser-induced forward transfer (LIFT) offers a high spatial resolution, accurate and controlled bio-ink deposition, and high post-printing cell viability. Effective bioprinting requires a deep understanding of material properties, especially the rheological behavior of bio-inks, which is critical for achieving the desired outcomes. Rheological characterization of these materials is essential for understanding their behavior under bioprinting conditions. The LIFT technique utilizes a wide range of soft biomaterials, generating printed structures containing cells, which proliferate for several days post-printing. These biomaterials can be controllably deposited in a variety of substrates. In this study, two cell-laden bio-inks with low and high number cell densities were printed at controlled depths within an extracellular matrix (ECM) by adjusting the laser energy. This process allows precise immobilization of cells at desired depths within the ECM using light and a proper optical setup. The rheological behavior of all bio-inks was analyzed using a microfabricated rheometer–viscometer on a chip. To investigate the transfer dynamics, a high-speed camera was integrated into the LIFT setup, monitoring the immobilization phenomenon within the ECM, and highlighting important characteristics of the jet propagations during printing. The morphological characteristics of the two sequential and distinct cell-laden jets were examined in detail during the printing process. This study showcases the ability to precisely deposit cells up to 2.5 mm deep within a soft matrix substrate, fabricating any desired cell-laden architecture for bio-engineering applications.

- Chliara MA, Elezoglou S, Zergioti I. Bioprinting on organ-on-chip: development and applications. Biosensors. 2022;12(12):1135. doi: 10.3390/bios12121135
- Delrot P, Hauser SP, Krizek J, Moser C. Depth-controlled laser-induced jet injection for direct three-dimensional liquid delivery. Appl Phys A. 2018;124(9):616. doi: 10.1007/s00339-018-2030-6
- Hinton TJ, Jallerat Q, Palchesko RN, et al. Three-dimensional printing of complex biological structures by freeform reversible embedding of suspended hydrogels. Sci Adv. 2015;1(9):e1500758. doi: 10.1126/sciadv.1500758
- Catros S, Guillotin B, Bačáková M, Fricain J-C, Guillemot F. Effect of laser energy, substrate film thickness and bioink viscosity on viability of endothelial cells printed by Laser- Assisted Bioprinting. Appl Surf Sci. 2011;257(12):5142-514. doi: 10.1016/j.apsusc.2010.11.049
- Elkaseer A, Chen KJ, Kuchta M, Scholz SG. On the quantitative assessment of the effect of multiple process parameters on the printed layer height in 3D inkjet printing. Virtual Phys Prototyp. 2023;18(1):e2269898. doi: 10.1080/17452759.2023.2269898
- Gruene M, Pflaum M, Deiwick A, et al. Adipogenic differentiation of laser-printed 3D tissue grafts consisting of human adipose-derived stem cells. Biofabrication. 2011;3(1):015005. doi: 10.1088/1758-5082/3/1/015005
- Gruene M, Pflaum M, Hess C, et al. Laser printing of three-dimensional multicellular arrays for studies of cell-cell and cell-environment interactions. Tissue Eng Part C Methods. 2011;17(10):973-982. doi: 10.1089/ten.TEC.2011.0185
- Catros S, Fricain JC, Guillotin B, et al. Laser-assisted bioprinting for creating on-demand patterns of human osteoprogenitor cells and nano-hydroxyapatite. Biofabrication. 2011;3(2):025001. doi: 10.1088/1758-5082/3/2/025001
- Koch L, Deiwick A, Franke A, et al. Laser bioprinting of human induced pluripotent stem cells-the effect of printing and biomaterials on cell survival, pluripotency, and differentiation. Biofabrication. 2018;10(3):035005. doi: 10.1088/1758-5090/aab981
- Sorkio A, Koch L, Koivusalo L, et al. Human stem cell based corneal tissue mimicking structures using laser-assisted 3D bioprinting and functional bioinks. Biomaterials. 2018;171:57-71. doi: 10.1016/j.biomaterials.2018.04.034
- Antoshin AA, Churbanov SN, Minaev NV, et al. LIFT-bioprinting, is it worth it? Bioprinting. 2019;15:e00052. doi: 10.1016/j.bprint.2019.e00052
- Guillotin B, Souquet A, Catros S, et al. Laser assisted bioprinting of engineered tissue with high cell density and microscale organization. Biomaterials. 2010;31(28):7250-7256. doi: 10.1016/j.biomaterials.2010.05.055
- Karakaidos P, Kryou C, Simigdala N, Klinakis A, Zergioti I. Laser bioprinting of cells using UV and visible wavelengths: a comparative DNA damage study. Bioengineering. 2022;9(8):378. doi: 10.3390/bioengineering9080378
- Murphy SV, Atala A. 3D bioprinting of tissues and organs. Nat Biotechnol. 2014;32(8):773-785. doi: 10.1038/nbt.2958
- Santos SC, Custódio CA, Mano JF. Human protein-based porous scaffolds as platforms for Xeno-free 3D cell culture. Adv Healthc Mater. 2022;11(12):2102383. doi: 10.1002/adhm.202102383
- Skrepetos S, Ranella A, Farsari M. 3D injectable mechanical metamaterials for tissue engineering applications. In: Conference on Lasers and Electro-Optics/Europe (CLEO/ Europe 2023) and European Quantum Electronics Conference (EQEC 2023), paper cm_p_3. Optica Publishing Group; 2023: cm_p_3. Accessed: Sep. 20, 2024. [Online]. Available: https://opg.optica.org/abstract.cfm?uri=CLEO_Europe- 2023-cm_p_3
- Ren Y, Yang X, Ma Z, et al. Developments and Opportunities for 3D Bioprinted Organoids. Int J Bioprint. 2021; 7(3):364. doi: 10.18063/ijb.v7i3.364
- Richards DJ, Li Y, Kerr CM, et al. Human cardiac organoids for the modelling of myocardial infarction and drug cardiotoxicity. Nat Biomed Eng. 2020;4(4):446-462. doi: 10.1038/s41551-020-0539-4
- Leite SB, Roosens T, El Taghdouini A, et al. Novel human hepatic organoid model enables testing of drug-induced liver fibrosis in vitro. Biomaterials. 2016;78:1-10. doi: 10.1016/j.biomaterials.2015.11.026
- Paraskevopoulou V, Papafotiou G, Klinakis A. KRT14 marks bladder progenitors. Cell Cycle. 2016;15(23):3161-3162. doi: 10.1080/15384101.2016.1220722
- Daly AC, Davidson MD, Burdick JA. 3D bioprinting of high cell-density heterogeneous tissue models through spheroid fusion within self-healing hydrogels. Nat Commun. 2021;12(1):753. doi: 10.1038/s41467-021-21029-2
- Jalaal M, Li S, Klein Schaarsberg M, Qin Y, Lohse D. Destructive mechanisms in laser induced forward transfer. Appl Phys Lett. 2019;114(21):213703. doi: 10.1063/1.5095520
- Wagner NJ, Mewis J, eds. Theory and Applications of Colloidal Suspension Rheology, 1st ed. Cambridge University Press; 2021. doi: 10.1017/9781108394826
- Denn MM, Morris JF, Bonn D. Shear thickening in concentrated suspensions of smooth spheres in Newtonian suspending fluids. Soft Matter. 2018;14(2):170-184. doi: 10.1039/C7SM00761B
- Stickel JJ, Powell RL. Fluid mechanics and rheology of dense suspensions. Annu Rev Fluid Mech. 2005;37(1):129-149. doi: 10.1146/annurev.fluid.36.050802.122132
- Pal R. Recent Progress in the viscosity modeling of concentrated suspensions of unimodal hard spheres. ChemEngineering. 2023;7(4):70. doi: 10.3390/chemengineering7040070
- Patrascioiu A, Fernández-Pradas JM, Palla-Papavlu A, Morenza JL, Serra P. Laser-generated liquid microjets: correlation between bubble dynamics and liquid ejection. Microfluid Nanofluid. 2014;16(1–2):55-63. doi: 10.1007/s10404-013-1218-5
- Unger C, Gruene M, Koch L, Koch J, Chichkov BN. Time-resolved imaging of hydrogel printing via laser-induced forward transfer. Appl Phys A. 2011;103(2):271-277. doi: 10.1007/s00339-010-6030-4
- Chen RCC, Yu YT, Su KW, Chen JF, Chen YF. Exploration of water jet generated by Q-switched laser induced water breakdown with different depths beneath a flat free surface. Opt Express. 2013;21(1):445. doi: 10.1364/OE.21.000445
- Cerbus RT, Chraibi H, Tondusson M, et al. Experimental and numerical study of laser-induced secondary jetting. J Fluid Mech. 2022;934:A14. doi: 10.1017/jfm.2021.1117
- Ali M, Pages E, Ducom A, Fontaine A, Guillemot F. Controlling laser-induced jet formation for bioprinting mesenchymal stem cells with high viability and high resolution. Biofabrication. 2014;6(4):045001. doi: 10.1088/1758-5082/6/4/045001
- Kalaitzis A. Jetting dynamics of Newtonian and non- Newtonian fluids via laser-induced forward transfer: experimental and simulation studies. Appl Surf Sci. 2019;465:136-142. doi: 10.1016/j.apsusc.2018.09.084