Three-dimensional-printed scaffolds functionalized with stem cell recruitment and cell respiration regulation for diabetic bone defects
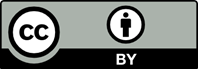
High-glucose microenvironment in diabetic patients is a source of damage to the cellular mitochondrial respiratory chain (MRC), which results in the generation of reactive oxygen species (ROS) and leads to mitochondrial dysfunction, cellular senescence, and enhanced apoptosis, eventually causing weakened cellular migration and differentiation as well as physical dysfunction. In patients with diabetic bone defects, the high-glucose microenvironment induces intracellular mitochondrial dysfunction and diminished migration and differentiation of bone marrow mesenchymal stem cells (BMSCs), leading to impaired bone regeneration. In this study, polycaprolactone (PCL) porous scaffolds were prepared by three-dimensional (3D) printing. The EPLQLKM (E7) and SS31 peptides were modified onto the surface of PCL porous scaffolds by chemical bonding to construct a 3D-printed porous scaffold system (PCL@SS31@E7) capable of stem cell recruitment and regulation of cellular MRC to treat diabetic bone defects. In vitro cellular energy metabolism and molecular biology experiments demonstrated that the scaffold system could continuously release E7 and SS31 peptides to recruit BMSCs, improve MRC function, reduce proton leakage, protect mitochondria, and promote proliferation and osteogenic differentiation of BMSCs to regenerate bone tissue in a high-glucose environment. In vivo experiments confirmed that the PCL@SS31@E7 porous scaffold induced regeneration of normal bone tissue in the area of femoral condylar bone defects in diabetic rats. The 3D-printed porous scaffold constructed in this study is a novel biomaterial with the functions of stem cell recruitment and targeted regulation of MRC and provides a new direction for the treatment of various diseases related to diabetes and MRC dysfunction.
- Zheng Y, Ley SH, Hu FB. Global aetiology and epidemiology of type 2 diabetes mellitus and its complications. Nat Rev Endocrinol. 2017;14(2):88-98. doi: 10.1038/nrendo.2017.151
- Cho NH, Shaw JE, Karuranga S, et al. IDF diabetes atlas: global estimates of diabetes prevalence for 2017 and projections for 2045. Diabetes Res Clin Pract. 2018;138: 271-281. doi: 10.1016/j.diabres.2018.02.023
- Hofbauer LC, Busse B, Eastell R, et al. Bone fragility in diabetes: novel concepts and clinical implications. Lancet Diabetes Endocrinol. 2022;10(3):207-220. doi: 10.1016/S2213-8587(21)00347-8
- Jiao H, Xiao E, Graves DT. Diabetes and its effect on bone and fracture healing. Curr Osteoporos Rep. 2015;13(5): 327-335. doi: 10.1007/s11914-015-0286-8
- Sun X, Ma Z, Zhao X, et al. Three-dimensional bioprinting of multicell-laden scaffolds containing bone morphogenic protein-4 for promoting M2 macrophage polarization and accelerating bone defect repair in diabetes mellitus. Bioact Mater. 2021;6(3):757-769. doi: 10.1016/j.bioactmat.2020.08.030
- Yin M, Zhang Y, Yu H, Li X. Role of hyperglycemia in the senescence of mesenchymal stem cells. Front Cell Dev Biol. 2021;9. doi: 10.3389/fcell.2021.665412
- Ali D, Tencerova M, Figeac F, Kassem M, Jafari A. The pathophysiology of osteoporosis in obesity and type 2 diabetes in aging women and men: the mechanisms and roles of increased bone marrow adiposity. Front Endocrinol. 2022;13. doi: 10.3389/fendo.2022.981487
- Khosla S, Samakkarnthai P, Monroe DG, Farr JN. Update on the pathogenesis and treatment of skeletal fragility in type 2 diabetes mellitus. Nat Rev Endocrinol. 2021;17(11):685-697. doi: 10.1038/s41574-021-00555-5
- Nauth A, Schemitsch E, Norris B, Nollin Z, Watson JT. Critical-size bone defects: is there a consensus for diagnosis and treatment? J Orthop Trauma. 2018;32(3):S7-S11. doi: 10.1097/BOT.0000000000001115
- Zhou J, See CW, Sreenivasamurthy S, Zhu D. Customized additive manufacturing in bone scaffolds—the gateway to precise bone defect treatment. Research. 2023;6. doi: 10.34133/research.0239
- Wu M, Gu J, Zong S, Guo R, Liu T, Yang M. Research journey of respirasome. Protein Cell. 2020;11(5):318-338. doi: 10.1007/s13238-019-00681-x
- Yan Y, Chen H, Zhang H, et al. Vascularized 3D printed scaffolds for promoting bone regeneration. Biomaterials. 2019;190-191:97-110. doi: 10.1016/j.biomaterials.2018.10.033
- Zheng D, Chen W, Ruan H, et al. Metformin-hydrogel with glucose responsiveness for chronic inflammatory suppression. Chem Eng J. 2022;428. doi: 10.1016/j.cej.2021.131064
- Chen H, Jia P, Kang H, et al. Upregulating Hif‐1α by hydrogel nanofibrous scaffolds for rapidly recruiting angiogenesis relative cells in diabetic wound. Adv Healthc Mater. 2016;5(8):907-918. doi: 10.1002/adhm.201501018
- Chen W, Zheng D, Chen Y, et al. Electrospun fibers improving cellular respiration via mitochondrial protection. Small. 2021;17(46). doi: 10.1002/smll.202104012
- Brachet A, Bełżek A, Furtak D, et al. Application of 3D printing in bone grafts. Cells. 2023;12(6). doi: 10.3390/cells12060859
- Murphy SV, De Coppi P, Atala A. Opportunities and challenges of translational 3D bioprinting. Nat Biomed Eng. 2019;4(4):370-380. doi: 10.1038/s41551-019-0471-7
- Miszuk JM, Xu T, Yao Q, et al. Functionalization of PCL- 3D electrospun nanofibrous scaffolds for improved BMP2- induced bone formation. Appl Mater Today. 2018;10: 194-202. doi: 10.1016/j.apmt.2017.12.004
- Szeto HH. Mitochondria-targeted cytoprotective peptides for ischemia–reperfusion injury. Antioxid Redox Signal. 2008;10(3):601-620. doi: 10.1089/ars.2007.1892
- Wu J, Zhang M, Hao S, et al. Mitochondria-targeted peptide reverses mitochondrial dysfunction and cognitive deficits in sepsis-associated encephalopathy. Mol Neurobiol. 2014;52(1):783-791. doi: 10.1007/s12035-014-8918-z
- Escribano-Lopez I, Diaz-Morales N, Iannantuoni F, et al. The mitochondrial antioxidant SS-31 increases SIRT1 levels and ameliorates inflammation, oxidative stress and leukocyte-endothelium interactions in type 2 diabetes. Sci Rep. 2018;8(1). doi: 10.1038/s41598-018-34251-8
- Li Q, Xing D, Ma L, Gao C. Synthesis of E7 peptide-modified biodegradable polyester with the improving affinity to mesenchymal stem cells. Mater Sci Eng C. 2017;73:562-568. doi: 10.1016/j.msec.2016.12.088
- Chen Z, Lv Z, Zhuang Y, et al. Mechanical signal‐tailored hydrogel microspheres recruit and train stem cells for precise differentiation. Adv Mater. 2023;35(40). doi: 10.1002/adma.202300180
- Dell RB, Holleran S, Ramakrishnan R. Sample size determination. ILAR J. 2002;43(4):207-213. doi: 10.1093/ilar.43.4.207
- Cole JB, Florez JC. Genetics of diabetes mellitus and diabetes complications. Nat Rev Nephrol. 2020;16(7):377-390. doi: 10.1038/s41581-020-0278-5
- Krako Jakovljevic N, Pavlovic K, Jotic A, et al. Targeting mitochondria in diabetes. Int J Mol Sci. 2021;22(12). doi: 10.3390/ijms22126642
- Rovira-Llopis S, Bañuls C, Diaz-Morales N, Hernandez- Mijares A, Rocha M, Victor VM. Mitochondrial dynamics in type 2 diabetes: pathophysiological implications. Redox Biol. 2017;11:637-645. doi: 10.1016/j.redox.2017.01.013
- Mohammadalipour A, Dumbali SP, Wenzel PL. Mitochondrial transfer and regulators of mesenchymal stromal cell function and therapeutic efficacy. Front Cell Dev Biol. 2020;8. doi: 10.3389/fcell.2020.603292
- Rathinavelu S, Guidry-Elizondo C, Banu J. Molecular modulation of osteoblasts and osteoclasts in Type 2 diabetes. J Diabetes Res. 2018;2018:1-11. doi: 10.1155/2018/6354787
- Hu Z, Ma C, Liang Y, Zou S, Liu X. Osteoclasts in bone regeneration under type 2 diabetes mellitus. Acta Biomater. 2019;84:402-413. doi: 10.1016/j.actbio.2018.11.052
- Zhou B, Zhang J-Y, Liu X-S, et al. Tom20 senses iron-activated ROS signaling to promote melanoma cell pyroptosis. Cell Res. 2018;28(12):1171-1185. doi: 10.1038/s41422-018-0090-y
- Zhang Z, Nam HK, Crouch S, Hatch NE. Tissue nonspecific alkaline phosphatase function in bone and muscleprogenitor cells: control of mitochondrial respiration and ATP production. Int J Mol Sci. 2021;22(3). doi: 10.3390/ijms22031140
- Salmi M. Additive manufacturing processes in medical applications. Materials. 2021;14(1). doi: 10.3390/ma14010191
- Ng WL, Huang X, Shkolnikov V, Suntornnond R, Yeong WY. Polyvinylpyrrolidone-based bioink: influence of bioink properties on printing performance and cell proliferation during inkjet-based bioprinting. Bio-Des Manuf. 2023;6(6):676-690. doi: 10.1007/s42242-023-00245-3
- Ramesh S, Harrysson OLA, Rao PK, et al. Extrusion bioprinting: recent progress, challenges, and future opportunities. Bioprinting. 2021;21. doi: 10.1039/d0bm00973c
- Chartrain NA, Williams CB, Whittington AR. A review on fabricating tissue scaffolds using vat photopolymerization. Acta Biomater. 2018;74:90-111. doi: 10.1016/j.actbio.2018.05.010
- Afsana, Jain V, Haider N, Jain K. 3D printing in personalized drug delivery. Curr Pharm Des. 2019;24(42):5062-5071. doi: 10.2174/1381612825666190215122208
- Salehi-Nik N, Amoabediny G, Shokrgozar MA, Mottaghy K, Klein-Nulend J, Zandieh-Doulabi B. Surface modification of silicone tubes by functional carboxyl and amine, but not peroxide groups followed by collagen immobilization improves endothelial cell stability and functionality. Biomed Mater. 2015;10(1). doi: 10.1088/1748-6041/10/1/015024
- Lam CXF, Hutmacher DW, Schantz JT, Woodruff MA, Teoh SH. Evaluation of polycaprolactone scaffold degradation for 6 months in vitro and in vivo. J Biomed Mater Res Part A. 2008;90A(3):906-919. doi: 10.1002/jbm.a.32052
- Hemmatpour H, De Luca O, Crestani D, et al. New insights in polydopamine formation via surface adsorption. Nat Commun. 2023;14(1). doi: 10.1038/s41467-023-36303-8
- Sun DT, Peng L, Reeder WS, et al. Rapid, selective heavy metal removal from water by a metal–organic framework/ polydopamine composite. ACS Cent Sci. 2018;4(3):349-356. doi: 10.1021/acscentsci.7b00605
- Shao Z, Zhang X, Pi Y, et al. Polycaprolactone electrospun mesh conjugated with an MSC affinity peptide for MSC homing in vivo. Biomaterials. 2012;33(12):3375-3387. doi: 10.1016/j.biomaterials.2012.01.033
- Zhang H, Alder NN, Wang W, Szeto H, Marcinek DJ, Rabinovitch PS. Reduction of elevated proton leak rejuvenates mitochondria in the aged cardiomyocyte. eLife. 2020;9. doi: 10.7554/eLife.60827
- Chakrabarty RP, Chandel NS. Mitochondria as signaling organelles control mammalian stem cell fate. Cell Stem Cell. 2021;28(3):394-408. doi: 10.1016/j.stem.2021.02.011
- Khorsand B, Acri TM, Do AV, et al. A multi‐functional implant induces bone formation in a diabetic model. Adv Healthc Mater. 2020;9(18). doi: 10.1002/adhm.202000770
- Schall N, Garcia JJ, Kalyanaraman H, et al. Protein kinase G1 regulates bone regeneration and rescues diabetic fracture healing. JCI Insight. 2020;5(9). doi: 10.1172/jci.insight.135355