Biomimetic mineralization of 3D-printed polyhydroxyalkanoate-based microbial scaffolds for bone tissue engineering
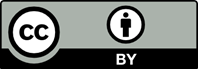
Polyhydroxyalkanoates (PHAs) have gained much attention as a potential alternative to conventional plastic bone scaffolds due to their biocompatibility and biodegradability, among a diverse range of advantageous properties. However, the water resistance of PHA creates an environment that can interfere with cell interactions. In this study, a three-dimensional-printed PHA scaffold was fabricated through fused deposition modeling printing considering the physical properties of PHA. The PHA bone scaffolds were then coated with polydopamine (pDA) and/or hydroxyapatite (HA) in various configurations using a relatively simple and rapid process involving only immersion. The PHA–pDA– HA scaffold showed enhanced cell viability, proliferation, and differentiation, and could thus serve as a versatile platform for bone tissue engineering applications.
- Lee JM, Yeong WY. Design and printing strategies in 3D bioprinting of cell-hydrogels: a review. Adv Healthc Mater. 2016;5(22):2856-2865. doi: 10.1002/adhm.201600435
- Naser AZ, Deiab I, Darras BM. Poly(lactic acid) (PLA) and polyhydroxyalkanoates (PHAs), green alternatives to petroleum-based plastics: a review. RSC Adv. 2021;11(28):17151-17196. doi: 10.1039/d1ra02390j
- Tomietto P, Russo F, Galiano F, et al. Sustainable fabrication and pervaporation application of bio-based membranes: combining a polyhydroxyalkanoate (PHA) as biopolymer and CyreneTM as green solvent. J Memb Sci. 2022;643:120061. doi: 10.1016/j.memsci.2021.120061
- Dwivedi R, Pandey R, Kumar S, Mehrotra D. Poly hydroxyalkanoates (PHA): role in bone scaffolds. J Oral Biol Craniofacial Res. 2020;10(1):389-392. doi: 10.1016/j.jobcr.2019.10.004
- Misra SK, Ansari TI, Valappil SP, et al. Poly(3- hydroxybutyrate) multifunctional composite scaffolds for tissue engineering applications. Biomaterials. 2010;31(10):2806-2815. doi: 10.1016/j.biomaterials.2009.12.045
- Guo W, Wang X, Yang C, Huang R, Wang H, Zhao Y. Microfluidic 3D printing polyhydroxyalkanoates-based bionic skin for wound healing. Mater Futur. 2022;1(1):015401. doi: 10.1088/2752-5724/ac446b
- Bao G, Jiang T, Ravanbakhsh H, et al. Triggered micropore-forming bioprinting of porous viscoelastic hydrogels. Mater Horiz. 2020;7(9):2336-2347. doi: 10.1039/d0mh00813c
- Gu BK, Choi DJ, Park SJ, Kim MS, Kang CM, Kim CH. 3-Dimensional bioprinting for tissue engineering applications. Biomater Res. 2016;20(1):1-8. doi: 10.1186/s40824-016-0058-2
- Li W, Mille LS, Robledo JA, Uribe T, Huerta V, Zhang YS. Recent advances in formulating and processing biomaterial inks for vat polymerization-based 3D printing. Adv Healthc Mater. 2020;9(15):2000156. doi: 10.1002/adhm.202000156
- Ng WL, Huang X, Shkolnikov V, Suntornnond R, Yeong WY. Polyvinylpyrrolidone-based bioink: influence of bioink properties on printing performance and cell proliferation during inkjet-based bioprinting. Bio-Design Manuf. 2023;6:676-690. doi: 10.1007/s42242-023-00245-3
- Fu Z, Naghieh S, Xu C, Wang C, Sun W, Chen X. Printability in extrusion bioprinting. Biofabrication. 2021;13(3). doi: 10.1088/1758-5090/abe7ab
- Mehrpouya M, Vahabi H, Barletta M, Laheurte P, Langlois V. Additive manufacturing of polyhydroxyalkanoates (PHAs) biopolymers: materials, printing techniques, and applications. Mater Sci Eng C. 2021;127:112216. doi: 10.1016/j.msec.2021.112216
- Tarrahi R, Fathi Z, Seydibeyoğlu MÖ, Doustkhah E, Khataee A. Polyhydroxyalkanoates (PHA): from production to nanoarchitecture. Int J Biol Macromol. 2020;146:596-619. doi: 10.1016/j.ijbiomac.2019.12.181
- Ryu J, Ku SH, Lee H, Park CB. Mussel-inspired polydopamine coating as a universal route to hydroxyapatite crystallization. Adv Funct Mater. 2010;20(13):2132-2139. doi: 10.1002/adfm.200902347
- Wang N, Yu X, Kong Q, et al. Nisin-loaded polydopamine/ hydroxyapatite composites: biomimetic synthesis, and in vitro bioactivity and antibacterial activity evaluations. Colloids Surf A Physicochem Eng Asp. 2020;602:125101. doi: 10.1016/j.colsurfa.2020.125101
- Liu Z, Qu S, Zheng X, et al. Effect of polydopamine on the biomimetic mineralization of mussel-inspired calcium phosphate cement in vitro. Mater Sci Eng C. 2014;44:44-51. doi: 10.1016/j.msec.2014.07.063
- Tseng SJ, Cheng CH, Lee TM, Lin JC. Studies of osteoblast-like MG-63 cellular proliferation and differentiation with cyclic stretching cell culture system on biomimetic hydrophilic layers modified polydimethylsiloxane substrate. Biochem Eng J. 2021;168:107946. doi: 10.1016/j.bej.2021.107946
- Zhang Y, Andrukhov O, Berner S, et al. Osteogenic properties of hydrophilic and hydrophobic titanium surfaces evaluated with osteoblast-like cells (MG63) in coculture with human umbilical vein endothelial cells (HUVEC). Dent Mater. 2010;26(11):1043-1051. doi: 10.1016/j.dental.2010.07.003
- Owji N, Mandakhbayar N, Gregory DA, et al. Mussel inspired chemistry and bacteria derived polymers for oral mucosal adhesion and drug delivery. Front Bioeng Biotechnol. 2021;9:1-13. doi: 10.3389/fbioe.2021.663764
- Luo H, Gu C, Zheng W, Dai F, Wang X, Zheng Z. Facile synthesis of novel size-controlled antibacterial hybrid spheres using silver nanoparticles loaded with poly-dopamine spheres. RSC Adv. 2015;5(18):13470-13477. doi: 10.1039/c4ra16469e
- Kim H, Lee K, Ko CY, et al. The role of nacreous factors in preventing osteoporotic bone loss through both osteoblast activation and osteoclast inactivation. Biomaterials. 2012;33(30):7489-7496. doi: 10.1016/j.biomaterials.2012.06.098
- Zhang X, Li J, Chen J, et al. Enhanced bone regeneration via PHA scaffolds coated with polydopamine-captured BMP2. J Mater Chem B. 2022;10(32):6214-6227. doi: 10.1039/d2tb01122k
- Auclair-Daigle C, Bureau MN, Legoux JG, Yahia L. Bioactive hydroxyapatite coatings on polymer composites for orthopedic implants. J Biomed Mater Res A. 2005;73(4):398-408. doi: 10.1002/jbm.a.30284
- Múzquiz-Ramos EM, Cortés-Hernández DA, Escobedo- Bocardo J. Biomimetic apatite coating on magnetite particles. Mater Lett. 2010;64(9):1117-1119. doi: 10.1016/j.matlet.2010.02.025
- Park JH, Lee DY, Oh KT, Lee YK, Kim KM, Kim KN. Bioactivity of calcium phosphate coatings prepared by electrodeposition in a modified simulated body fluid. Mater Lett. 2006;60(21-22):2573-2577. doi: 10.1016/j.matlet.2005.07.091
- Guo W, Yang K, Qin X, Luo R, Wang H, Huang R. Polyhydroxyalkanoates in tissue repair and regeneration. Eng Regen. 2022;3(1):24-40. doi: 10.1016/j.engreg.2022.01.003
- Garot C, Bettega G, Picart C. Additive manufacturing of material scaffolds for bone regeneration: toward application in the clinics. Adv Funct Mater. 2021;31(5):2006967. doi: 10.1002/adfm.202006967
- Lichte P, Pape HC, Pufe T, Kobbe P, Fischer H. Scaffolds for bone healing: concepts, materials and evidence. Injury. 2011;42(6):569-573. doi: 10.1016/j.injury.2011.03.033
- Dhania S, Bernela M, Rani R, et al. Scaffolds the backbone of tissue engineering: advancements in use of polyhydroxyalkanoates (PHA). Int J Biol Macromol. 2022;208:243-259. doi: 10.1016/j.ijbiomac.2022.03.030
- Elmowafy E, Abdal-Hay A, Skouras A, Tiboni M, Casettari L, Guarino V. Polyhydroxyalkanoate (PHA): applications in drug delivery and tissue engineering. Exp Rev Med Dev. 2019;16(6):467-482. doi: 10.1080/17434440.2019.1615439
- Li L, Li Y, Yang L, et al. Polydopamine coating promotes early osteogenesis in 3D printing porous Ti6Al4V scaffolds. Ann Transl Med. 2019;7(11):240-240. doi: 10.21037/atm.2019.04.79
- Kao CT, Lin CC, Chen YW, Yeh CH, Fang HY, Shie MY. Poly(dopamine) coating of 3D printed poly(lactic acid) scaffolds for bone tissue engineering. Mater Sci Eng C. 2015;56:165-173. doi: 10.1016/j.msec.2015.06.028
- Jo S, Kang SM, Park SA, Kim WD, Kwak J, Lee H. Enhanced adhesion of preosteoblasts inside 3D PCL scaffolds by polydopamine coating and mineralization. Macromol Biosci. 2013;13(10):1389-1395. doi: 10.1002/mabi.201300203
- Im S, Choe G, Seok JM, et al. An osteogenic bioink composed of alginate, cellulose nanofibrils, and polydopamine nanoparticles for 3D bioprinting and bone tissue engineering. Int J Biol Macromol. 2022;205: 520-529. doi: 10.1016/j.ijbiomac.2022.02.012
- Park J, Lee SJ, Jung TG, et al. Surface modification of a three-dimensional polycaprolactone scaffold by polydopamine, biomineralization, and BMP-2 immobilization for potential bone tissue applications. Colloids Surf B Biointerfaces. 2021;199:111528. doi: 10.1016/j.colsurfb.2020.111528
- Loty C, Sautier JM, Boulekbache H, Kokubo T, Kim HM, Forest N. In vitro bone formation on a bone-like apatite layer prepared by a biomimetic process on a bioactive glass-ceramic. J Biomed Mater Res. 2000;49(4): 423-434. doi: 10.1002/(sici)1097-4636(20000315)49:4<423::aid-jbm1>3.0.co;2-7
- Konka J, Buxadera-Palomero J, Espanol M, Ginebra MP. 3D printing of hierarchical porous biomimetic hydroxyapatite scaffolds: adding concavities to the convex filaments. Acta Biomater. 2021;134:744-759. doi: 10.1016/j.actbio.2021.07.071
- Avanzi IR, Parisi JR, Souza A, et al. 3D-printed hydroxyapatite scaffolds for bone tissue engineering: a systematic review in experimental animal studies. J Biomed Mater Res B Appl Biomater. 2023;111(1):203-219. doi: 10.1002/jbm.b.35134
- Barba A, Maazouz Y, Diez-Escudero A, et al. Osteogenesis by foamed and 3D-printed nanostructured calcium phosphate scaffolds: effect of pore architecture. Acta Biomater. 2018;79:135-147. doi: 10.1016/j.actbio.2018.09.003