Enhanced osteogenesis and bactericidal performance of additively manufactured MgO-and Cu-added CpTi for load-bearing implants
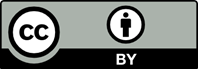
The bio-inertness of titanium, which is the ultimate choice of metallic material for implant applications, causes delayed bone–tissue integration at the implant site and prevents expedited healing for the patient. This can result in a severe issue for patients with immunocompromised bone health as titanium does not offer inherent antimicrobial properties, and thus, infections at the implant site are another concern. Current strategies addressing the issues above include using cemented implants as a coating on Ti6Al4V bulk material for orthopedic applications. Roadblock arises with coating failure due to weak interfacial bond at the Ti–cement interface, which necessitates revision surgeries. In this study, we added osteogenic MgO and antibacterial Cu to commercially pure titanium (CpTi) and processed them using metal additive manufacturing. Mg, an essential trace element in the body, has been proven to enhance osseointegration in vivo. Cu has been popular for its bactericidal capabilities. With the addition of 1 wt.% of MgO to the CpTi matrix, we observed a four-fold increase in the mineralized bone formation at the bone–implant interface in vivo. The addition of 3 wt.% of Cu did not result in cytotoxicity, and adding Cu to CpTi-MgO chemical makeup yielded in vivo performance similar to that in CpTi-MgO. In in vitro bacterial studies with gram-positive Staphylococcus aureus, CpTi-MgO-Cu displayed an antibacterial efficacy of 81% at the end of 72 h of culture. Our findings highlight the synergistic benefits of CpTi-MgO-Cu, which exhibit superior early-stage osseointegration and antimicrobial capabilities.
- Liu X, Chu PK, Ding C, 2004, Surface modification of titanium, titanium alloys, and related materials for biomedical applications. Mater Sci Eng R Rep, 47(3): 49–121. https://doi.org/10.1016/j.mser.2004.11.001
- Niinomi M, 2007, Recent research and development in metallic materials for biomedical, dental and healthcare products applications. Mater Sci Forum, 539: 193–200. https://doi.org/10.4028/0-87849-428-6.193
- Van Noort R, 1987, Titanium: The implant material of today. J Mater Sci, 22(11): 3801–3811. https://doi.org/10.1007/BF01133326
- Dong YP, Tang JC, Wang DW, et al., 2020, Additive manufacturing of pure Ti with superior mechanical performance, low cost, and biocompatibility for potential replacement of Ti-6Al-4V. Mater Des, 196(2020): 109142. https://doi.org/10.1016/j.matdes.2020.109142
- Feng J, Wei D, Zhang P, et al., 2013, Preparation of TiNbTaZrMo high-entropy alloy with tunable Young’s modulus by selective laser melting. J Manuf Process, 85(2023): 160–165. https://doi.org/10.1016/j.jmapro.2022.11.046
- Cui Y-W, Chen L-Y, Chu Y-H, et al., 2023, Metastable pitting corrosion behavior and characteristics of passive film of laser powder bed fusion produced Ti–6Al–4V in NaCl solutions with different concentrations. Corros Sci, 126(2022): 111017. https://doi.org/10.1016/j.corsci.2023.111017
- Ciliveri S, Bandyopadhyay A, 2021, Influence of strut-size and cell-size variations on porous Ti6Al4V coated structures for load-bearing implants. J Mech Behav Biomed Mater, 105023. https://doi.org/10.1016/j.jmbbm.2021.105023
- Ke D, Robertson SF, Dernell WS, et al., 2017, Effects of MgO and SiO2 on plasma-sprayed hydroxyapatite coating: An in vivo study in rat distal femoral defects. ACS Appl Mater Interfaces, 9(31): 25731–25737. https://doi.org/10.1021/acsami.7b05574
- Bandyopadhyay A, Bernard S, Xue W, et al., 2006, Calcium phosphate-based resorbable ceramics: Influence of MgO, ZnO, and SiO2 dopants. J Am Ceram Soc, 89(9): 2675–2688. https://doi.org/10.1111/j.1551-2916.2006.01207.x
- Fielding G, Bose S, 2013, SiO2 and ZnO dopants in three-dimensionally printed tricalcium phosphate bone tissue engineering scaffolds enhance osteogenesis and angiogenesis in vivo. Acta Biomater, 9(11): 9137–9148. https://doi.org/10.1016/j.actbio.2013.07.009
- Libraty DH, Patkar C, Torres B, 2012, Staphylococcus aureus reactivation osteomyelitis after 75 years. N Engl J Med, 366(5): 481–482. https://doi.org/10.1056/NEJMc1111493
- Macheras GA, Kateros K, Galanakos SP, et al., 2011, The long-term results of a two-stage protocol for revision of an infected total knee replacement. J Bone Joint Surg Br, 93(11): 1487–1492. https://doi.org/10.1302/0301-620X.93B11.27319
- Windsor RE, Insall JN, Urs WK, et al., 1990, Two-stage reimplantation for the salvage of total knee arthroplasty complicated by infection. Further follow-up and refinement of indications. J Bone Joint Surg Am, 72(2): 272–278.
- Buechel FF, 2004, The infected total knee arthroplasty: Just when you thought it was over. J Arthroplasty, 19(4 Suppl 1): 51–55. https://doi.org/10.1016/j.arth.2004.03.001
- Zmistowski BM, 2013, A Quarter of Patients Treated for PJI Dead Within 5 Years. https://www.healio.com/news/orthopedics/20130104/a-quarter-of-patients-treated-for-pji-dead-within-5-years (accessed 2023-06-11).
- O’Neill J, 2016, Tackling Drug-Resistant Infections Globally: Final Report and Recommendations. Government of the United Kingdom, United Kingdom. https://apo.org.au/node/63983 (accessed 2023-06-11).
- Nishiguchi S, Nakamura T, Kobayashi M, et al., 1999, The effect of heat treatment on bone-bonding ability of alkali-treated titanium. Biomaterials, 20(5): 491–500. https://doi.org/10.1016/S0142-9612(98)90203-4
- Tang J, Wu Z, Yao X, et al., 2022, From bio-inertness to osseointegration and antibacterial activity: A one-step micro-arc oxidation approach for multifunctional Ti implants fabricated by additive manufacturing. Mater Des, 221: 110962. https://doi.org/10.2139/ssrn.4121117
- Costello RB, Rosanoff A, 2020, Magnesium, in Present Knowledge in Nutrition (Eleventh Edition). Marriott BP, Birt DF, Stallings VA, et al., eds., Academic Press, Cambridge, MA, 349–373. https://doi.org/10.1016/B978-0-323-66162-1.00021-4
- Staiger MP, Pietak AM, Huadmai J, et al., 2006, Magnesium and its alloys as orthopedic biomaterials: A review. Biomaterials, 27(9): 1728–1734. https://doi.org/10.1016/j.biomaterials.2005.10.003
- Xia X, Huang J, Wei J, et al., 2022, Magnesium oxide regulates the degradation behaviors and improves the osteogenesis of poly(lactide-co-glycolide) composite scaffolds. Compos Sci Technol, 222: 109368. https://doi.org/10.1016/j.compscitech.2022.109368
- Zhao Y, Liang H, Zhang S, et al., 2020, Effects of magnesium oxide (MgO) shapes on in vitro and in vivo degradation behaviors of PLA/MgO composites in long term. Polymers, 12(5): 1074. https://doi.org/10.3390/polym12051074
- Zreiqat H, Howlett CR, Zannettino A, et al., 2002, Mechanisms of magnesium-stimulated adhesion of osteoblastic cells to commonly used orthopaedic implants. J Biomed Mater Res, 62(2): 175–184. https://doi.org/10.1002/jbm.10270
- Niknam Z, Golchin A, Rezaei-Tavirani M, et al., 2022, Osteogenic differentiation potential of adipose-derived mesenchymal stem cells cultured on magnesium oxide/ polycaprolactone nanofibrous scaffolds for improving bone tissue reconstruction. Adv Pharm Bull, 12(1): 142–154. https://doi.org/10.34172/apb.2022.015
- Roy M, Balla VK, Bandyopadhyay A, et al., 2012, MgO-doped tantalum coating on Ti: Microstructural study and biocompatibility evaluation. ACS Appl Mater Interfaces, 4(2): 577–580. https://doi.org/10.1021/am201365e
- Xue W, Dahlquist K, Banerjee A, et al., 2008, Synthesis and characterization of tricalcium phosphate with Zn and Mg based dopants. J Mater Sci Mater Med, 19(7): 2669–2677. https://doi.org/10.1007/s10856-008-3395-4
- Kim D-H, Shin K-K, Jung JS, et al., 2015, The role of magnesium ion substituted biphasic calcium phosphate spherical micro-scaffolds in osteogenic differentiation of human adipose tissue-derived mesenchymal stem cells. J Nanosci Nanotechnol, 15(8): 5520–5523. https://doi.org/10.1166/jnn.2015.10463
- 2017, Long-lived biomaterials. Nat Biomed Eng, 1(6): 1–1. https://www.nature.com/articles/s41551-017-0095#citeas
- Mohammadi H, Sepantafar M, 2015, Ion-doped silicate bioceramic coating of Ti-based implant. Iran Biomed J, 20: 189–200. https://doi.org/10.7508/ibj.2016.04.002
- Breme J, Zhou Y, Groh L, 1995, Development of a titanium alloy suitable for an optimized coating with hydroxyapatite. Biomaterials, 16(3): 239–244. https://doi.org/10.1016/0142-9612(95)92123-N
- Guo L, Ataollah Naghavi S, Wang Z, et al., 2022, On the design evolution of hip implants: A review. Mater Des, 216: 110552. https://doi.org/10.1016/j.matdes.2022.110552
- Lionberger D, Conlon C, Wattenbarger L, et al., 2019, Unacceptable failure rate of a ceramic-coated posterior cruciate-substituting total knee arthroplasty. Arthroplast Today, 5(2): 187–192. https://doi.org/10.1016/j.artd.2019.02.002
- Bandyopadhyay A, Ciliveri S, Bose S, 2022, Metal additive manufacturing for load-bearing implants. J Indian Inst Sci, 102.1(2022): 561–584. https://doi.org/10.1007/s41745-021-00281-x
- Traxel KD, Bandyopadhyay A, 2022, Selective laser melting of Ti6Al4V-B4C-BN in situ reactive composites. J Mater Res Technol, 18: 2654–2671. https://doi.org/10.1016/j.jmrt.2022.03.092
- Taniguchi N, Fujibayashi S, Takemoto M, et al., 2016, Effect of pore size on bone ingrowth into porous titanium implants fabricated by additive manufacturing: An in vivo experiment. Mater Sci Eng C, 59: 690–701. https://doi.org/10.1016/j.msec.2015.10.069
- Ouyang P, Dong H, He X, et al., 2019, Hydromechanical mechanism behind the effect of pore size of porous titanium scaffolds on osteoblast response and bone ingrowth. Mater Des, 183: 108151. https://doi.org/10.1016/j.matdes.2019.108151
- Imai K, Ikeshoji T-T, Sugitani Y, et al., 2020, Densification of pure copper by selective laser melting process. Mech Eng J, 7(2): 19-00272-19–00272. https://doi.org/10.1299/mej.19-00272
- Guschlbauer R, Burkhardt AK, Fu Z, et al., 2020, Effect of the oxygen content of pure copper powder on selective electron beam melting. Mater Sci Eng A, 779: 139106. https://doi.org/10.1016/j.msea.2020.139106
- Mitra I, Bose S, Dernell WS, et al., 2021, 3D printing in alloy design to improve biocompatibility in metallic implants. Mater Today, 45: 20–34. https://doi.org/10.1016/j.mattod.2020.11.021
- Hall M, Frank E, Holmes G, et al., 2009, The WEKA data mining software: An update. ACM SIGKDD Explor Newsl, 11(1): 10–18. https://doi.org/10.1145/1656274.1656278
- Arganda-Carreras I, Kaynig V, Rueden C, et al., 2017, Trainable Weka segmentation: A machine learning tool for microscopy pixel classification. Bioinformatics, 33(15): 2424–2426. https://doi.org/10.1093/bioinformatics/btx180
- Afrouzian A, Groden CJ, Field DP, et al., 2002, Additive manufacturing of Ti-Ni bimetallic structures. Mater Des, 215: 110461. https://doi.org/10.1016/j.matdes.2022.110461
- Newby E, Yadroitsava I, Krakhmalev P, et al., 2019, Investigation of in-situ alloying grade 23 Ti with 5 at . % Cu by laser powder bed fusion for biomedical applications. Mater Sci, 197859609.
- Svetlizky D, Das M, Zheng B, et al., 2021, Directed energy deposition (DED) additive manufacturing: Physical characteristics, defects, challenges and applications. Mater Today, 49: 271–295. https://doi.org/10.1016/j.mattod.2021.03.020
- Assael MJ, Kalyva AE, Antoniadis KD, et al., 2010, Reference data for the density and viscosity of liquid copper and liquid tin. J Phys Chem Ref Data, 39(3): 033105. https://doi.org/10.1063/1.3467496
- Paradis P-F, Ishikawa T, Yoda S, 2002, Non-contact measurements of surface tension and viscosity of niobium, zirconium, and titanium using an electrostatic levitation furnace. Int J Thermophys, 23(3): 825–842. https://doi.org/10.1023/A:1015459222027
- Mosallanejad MH, Niroumand B, Aversa A, et al., 2021, Laser powder bed fusion in-situ alloying of Ti-5%Cu alloy: Process-structure relationships. J Alloys Compd, 857: 157558. https://doi.org/10.1016/j.jallcom.2020.157558
- Ciliveri S, Mitra I, Bose S, et al., 2022, Effects of oxide ceramic addition on biocompatibility of titanium, in Metal-Matrix Composites, Srivatsan TS, Rohatgi PK, Hunyadi Murph S, eds., Springer International Publishing, Cham, 323–334. https://doi.org/10.1007/978-3-030-92567-3_20
- Onuike B, Heer B, Bandyopadhyay A, 2018, Additive Manufacturing of Inconel 718 – Copper Alloy Bimetallic Structure using Laser Engineered Net Shaping. Addit Manuf, 21: 133–140. https://doi.org/10.1016/j.addma.2018.02.007
- Kikuchi M, Takada Y, Kiyosue S, et al., 2003, Mechanical properties and microstructures of cast Ti–Cu alloys. Dent Mater, 19(3): 174–181. https://doi.org/10.1016/S0109-5641(02)00027-1
- Zhang L, Chang M, Beck CA, et al., 2016, Analysis of new bone, cartilage, and fibrosis tissue in healing murine allografts using whole slide imaging and a new automated histomorphometric algorithm. Bone Res, 4: 15037. https://doi.org/10.1038/boneres.2015.37
- Itabashi T, Narita K, Ono A, et al., 2017, Bactericidal and antimicrobial effects of pure titanium and titanium alloy treated with short-term, low-energy UV irradiation. Bone Jt Res, 6(2): 108–112.https://doi.org/10.1302/2046-3758.62.2000619
- Arciola CR, Campoccia D, Montanaro L, 2018, Implant infections: Adhesion, biofilm formation and immune evasion. Nat Rev Microbiol, 16(7): 397–409. https://doi.org/10.1038/s41579-018-0019-y
- O’Driscoll NH, Cushnie TPT, Matthews KH, et al., 2018, Colistin causes profound morphological alteration but minimal cytoplasmic membrane perforation in populations of Escherichia coli and Pseudomonas aeruginosa. Arch Microbiol, 200(5): 793–802. https://doi.org/10.1007/s00203-018-1485-3
- Centeno C, 2021, The Evidence Supporting Common Orthopedic Surgeries Is AWFUL. Regenexx. https://regenexx.com/blog/the-evidence-supporting-common-orthopedic-surgeries-is-awful/ (accessed 2023- 06-20).
- Shukla D, Patel S, Clack L, et al., 2021, Retrospective analysis of trends in surgery volumes between 2016 and 2019 and impact of the insurance deductible: Cross-sectional study. Ann Med Surg, 63: 102176. https://doi.org/10.1016/j.amsu.2021.02.022
- Orthopedic Surgery Fact Sheet from the National Ambulatory Medical Care Survey. https://www.cdc.gov/nchs/data/namcs/factsheets/NAMCS- 2015-16-Orthopedic-Surgery-508.pdf
- Clark D, Nakamura M, Miclau T, et al., 2017, Effects of aging on fracture healing. Curr Osteoporos Rep, 15(6): 601–608. https://doi.org/10.1007/s11914-017-0413-9
- Tarafder S, Dernell WS, Bandyopadhyay A, et al., 2015, SrO-and MgO-doped microwave sintered 3D printed tricalcium phosphate scaffolds: Mechanical properties and in vivo osteogenesis in a rabbit model. J Biomed Mater Res B Appl Biomater, 103(3): 679–690. https://doi.org/10.1002/jbm.b.33239
- Nandi SK, Roy M, Bandyopadhyay A, et al., 2023, In vivo biocompatibility of SrO and MgO doped brushite cements. J Biomed Mater Res B Appl Biomater, 111(3): 599–609. https://doi.org/10.1002/jbm.b.35177
- Schmitz C, Deason F, Perraud A-L, 2007, Molecular components of vertebrate Mg2+-homeostasis regulation. Magnes Res, 20(1): 6–18.
- Singh S, Vashisth P, Shrivastav A, et al., 2019, Synthesis and characterization of a novel open cellular Mg-based scaffold for tissue engineering application. J Mech Behav Biomed Mater, 94(2019): 54–62. https://doi.org/10.1016/j.jmbbm.2019.02.010
- Chakraborty Banerjee P, Al-Saadi S, Choudhary L, et al., 2019, Magnesium implants: Prospects and challenges. Materials, 12(1): 136. https://doi.org/10.3390/ma12010136
- Dollwet HHA, Sorenson JRJ, 1985, Historic uses of copper compounds in medicine. Trace Elem Med, 2(2): 80–87.
- Grass G, Rensing C, Solioz M, 2011, Metallic copper as an antimicrobial surface. Appl Environ Microbiol, 77(5): 1541–1547. https://doi.org/10.1128/AEM.02766-10
- EPA Press Office, 2021, EPA Registers Copper Surfaces for Residual Use Against Coronavirus. https://www.epa.gov/newsreleases/epa-registers-copper-surfaces-residual-use-against-coronavirus (accessed 2023- 06-20).
- Tchounwou PB, Newsome C, Williams J, et al., 2008, Copper-induced cytotoxicity and transcriptional activation of stress genes in human liver carcinoma (HepG2) cells. Met Ions Biol Med, 10: 285–290.
- Liu R, Memarzadeh K, Chang B, et al., 2016, Antibacterial effect of copper-bearing titanium alloy (Ti-Cu) against Streptococcus Mutans and Porphyromonas gingivalis. Sci Rep, 6: 29985. https://doi.org/10.1038/srep29985
- Liu R, Tang Y, Zeng L, et al., 2018, In vitro and in vivo studies of antibacterial copper-bearing titanium alloy for dental application. Dent Mater, 34(8): 1112–1126. https://doi.org/10.1016/j.dental.2018.04.007
- Zhang E, Li F, Wang H, et al., 2013, A new antibacterial titanium–copper sintered alloy: Preparation and antibacterial property. Mater Sci Eng C, 33(7): 4280–4287. https://doi.org/10.1016/j.msec.2013.06.016
- Liu J, Li F, Liu C, et al., 2014, Effect of Cu content on the antibacterial activity of titanium–copper sintered alloys. Mater Sci Eng C, 35(2014): 392–400. https://doi.org/10.1016/j.msec.2013.11.028