Micron track chitosan conduit fabricated by 3D-printed model topography provides bionic microenvironment for peripheral nerve regeneration
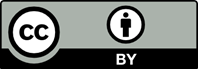
Correction for this article is available: view correction
The micron track conduit (MTC) and nerve factor provide a physical and biological model for simulating peripheral nerve growth and have potential applications for nerve injury. However, it has rarely been reported that they synergize on peripheral nerves. In this study, we used bioderived chitosan as a substrate to design and construct a neural repair conduit with micron track topography using threedimensional (3D) printing topography. We loaded the MTC with neurotrophin-3 (NT-3) to promote the regeneration of sensory and sympathetic neurons in the peripheral nervous system. We found that the MTC@NT3 composite nerve conduit mimicked the microenvironment of peripheral nerves and promoted axonal regeneration while inducing the targeted growth of Schwann cells, which would promote functional recovery in rats with peripheral nerve injury. Artificial nerve implants with functional properties can be developed using the strategy presented in this study.
Houdek MT, Shin AY, 2015, Management and complications of traumatic peripheral nerve injuries. Hand Clin, 31(2): 151–63. https://doi.org/10.1016/j.hcl.2015.01.007
Taylor CA, Braza D, Rice JB, et al., 2008, The incidence of peripheral nerve injury in extremity trauma. Am J Phys Med Rehabil, 87(5): 381–385. https://doi.org/10.1097/PHM.0b013e31815e6370
Grijalvo S, Díaz DD, 2021, Graphene-based hybrid materials as promising scaffolds for peripheral nerve regeneration. Neurochem Int, 147: 105005. https://doi.org/10.1016/j.neuint.2021.105005
Yi S, Xu L, Gu X, 2019, Scaffolds for peripheral nerve repair and reconstruction. Exp Neurol, 319: 112761. https://doi.org/10.1016/j.expneurol.2018.05.016
Yin G, Peng Y, Lin Y, et al., 2021, Long non-coding RNA MSTRG.24008.1 regulates the regeneration of the sciatic nerve via the miR-331-3p-NLRP3/MAL axis. Front Cell Dev Biol, 9: 641603. https://doi.org/10.3389/fcell.2021.641603
Bombeiro AL, Santini JC, Thomé R, et al., 2016, Enhanced immune response in immunodeficient mice improves peripheral nerve regeneration following axotomy. Front Cell Neurosci, 10: 151. https://doi.org/10.3389/fncel.2016.00151
Jiang H, Wang X, Li X, et al., 2022, A multifunctional ATP-generating system by reduced graphene oxide-based scaffold repairs neuronal injury by improving mitochondrial function and restoring bioelectricity conduction. Mater Today Bio, 13: 100211. https://doi.org/10.1016/j.mtbio.2022.100211
Mendes PM, 2008, Stimuli-responsive surfaces for bio-applications. Chem Soc Rev, 37(11): 2512–2529. https://doi.org/10.1039/b714635n
Zhu L, Jia S, Liu T, et al., 2020, Aligned PCL fiber conduits immobilized with nerve growth factor gradients enhance and direct sciatic nerve regeneration. 30(39): 2002610. https://doi.org/10.1002/adfm.202002610
Houshyar S, Bhattacharyya A, Shanks R, 2019, Peripheral nerve conduit: Materials and Structures. ACS Chem Neurosci, 10(8): 3349–3365. https://doi.org/10.1021/acschemneuro.9b00203
Zhang J, Zhang X, Wang C, et al., 2021, Conductive composite fiber with optimized alignment guides neural regeneration under electrical stimulation. 10(3): 2000604. https://doi.org/10.1002/adhm.202000604
Zhang X, Qu W, Li D, et al., 2020, Functional polymer-based nerve guide conduits to promote peripheral nerve regeneration. Adv Mater Interfaces, 7(14): 2000225. https://doi.org/10.1002/admi.202000225
Gong B, Zhang X, Zahrani AA, et al., 2022, Neural tissue engineering: From bioactive scaffolds and in situ monitoring to regeneration. Exploration, 2(3): 20210035. https://doi.org/10.1002/EXP.20210035
Li G, Li S, Zhang L, et al., 2019, Construction of biofunctionalized anisotropic hydrogel micropatterns and their effect on schwann cell behavior in peripheral nerve regeneration. ACS Appl Mater Interfaces, 11(41): 37397–37410. https://doi.org/10.1021/acsami.9b08510
Grasman JM, Ferreira JA, Kaplan DL, 2018, Tissue models for neurogenesis and repair in 3D. Adv Funct Mater, 28(48): 1803822. https://doi.org/10.1002/adfm.201803822
Liu C, Chan C, 2016, An approach to enhance alignment and myelination of dorsal root ganglion neurons. J Vis Exp, (114): 54085. https://doi.org/10.3791/54085
Jia Y, Yang W, Zhang K, et al., 2019, Nanofiber arrangement regulates peripheral nerve regeneration through differential modulation of macrophage phenotypes. Acta Biomater, 83: 291–301. https://doi.org/10.1016/j.actbio.2018.10.040
Hoffman-Kim D, Mitchel JA, Bellamkonda RV, 2010, Topography, cell response, and nerve regeneration. Annu Rev Biomed Eng, 12: 203–231. https://doi.org/10.1146/annurev-bioeng-070909-105351
Huang C, Ouyang Y, Niu H, et al., 2015, Nerve guidance conduits from aligned nanofibers: Improvement of nerve regeneration through longitudinal nanogrooves on a fiber surface. ACS Appl Mater Interfaces, 7(13): 7189–7196. https://doi.org/10.1021/am509227t
Zhang Y, Xu Y, Kong H, et al., 2023, Microneedle system for tissue engineering and regenerative medicine. Exploration, 3(1): 20210170. https://doi.org/10.1002/EXP.20210170
Liu K, Yan L, Li R, et al., 3D printed personalized nerve guide conduits for precision repair of peripheral nerve defects. Adv Sci, 9(12): 2103875. https://doi.org/10.1002/advs.202103875
Ding J, Zhang J, Li J, et al., 2019, Electrospun polymer biomaterials. Prog Polym Sci, 90: 1–34. https://doi.org/10.1016/j.progpolymsci.2019.01.002
Wan X, Zhao Y, Li Z, et al., 2022, Emerging polymeric electrospun fibers: From structural diversity to application in flexible bioelectronics and tissue engineering. Exploration, 2(1): 20210029. https://doi.org/10.1002/EXP.20210029
Zhao C, Wang X, Gao L, et al., 2018, The role of the micro-pattern and nano-topography of hydroxyapatite bioceramics on stimulating osteogenic differentiation of mesenchymal stem cells. Acta Biomater, 73: 509–521. https://doi.org/10.1016/j.actbio.2018.04.030
Mansouri N, Bagheri S, 2016, The influence of topography on tissue engineering perspective. Mater Sci Eng C Mater Biol Appl, 61: 906–921. https://doi.org/10.1016/j.msec.2015.12.094
Klymov A, Rodrigues Neves CT, te Riet J, et al., 2015, Nanogrooved surface-patterns induce cellular organization and axonal outgrowth in neuron-like PC12-cells. Hear Res, 320: 11–17. https://doi.org/10.1016/j.heares.2014.12.009
Zhang D, Yao Y, Duan Y, et al., 2020, Surface-anchored graphene oxide nanosheets on cell-scale micropatterned poly(d,l-lactide-co-caprolactone) conduits promote peripheral nerve regeneration. ACS Appl Mater Interfaces, 12(7): 7915–7930. https://doi.org/10.1021/acsami.9b20321
Omidinia-Anarkoli A, Ephraim JW, Rimal R, et al., 2020, Hierarchical fibrous guiding cues at different scales influence linear neurite extension. Acta Biomater, 113: 350–359. https://doi.org/10.1016/j.actbio.2020.07.014
Li G, Zheng T, Wu L, et al., 2021, Bionic microenvironment-inspired synergistic effect of anisotropic micro-nanocomposite topology and biology cues on peripheral nerve regeneration. Sci Adv, 7(28): 5812. https://doi.org/10.1126/sciadv.abi5812
Li G, Zhao X, Zhang L, et al., 2014, Regulating Schwann cells growth by chitosan micropatterning for peripheral nerve regeneration in vitro. Macromol Biosci, 14(8): 1067–1075. https://doi.org/10.1002/mabi.201400098
Iadecola C, 2010, The overlap between neurodegenerative and vascular factors in the pathogenesis of dementia. Acta Neuropathol, 120(3): 287–296. https://doi.org/10.1007/s00401-010-0718-6
Martínez G, Khatiwada S, Costa-Mattioli M, et al., 2018, ER proteostasis control of neuronal physiology and synaptic function. Trends Neurosci, 41(9): 610–624. https://doi.org/10.1016/j.tins.2018.05.009
Wang T, Zhong X, Wang S, et al., 2012, Molecular mechanisms of RADA16-1 peptide on fast stop bleeding in rat models. Int J Mol Sci, 13(11): 15279–15290. https://doi.org/10.3390/ijms131115279
Hu G, Xiao L, Tong P, et al., 2012, Antibacterial hemostatic dressings with nanoporous bioglass containing silver. Int J Nanomed, 7: 2613–2620. https://doi.org/10.2147/ijn.S31081
Dong H, Li Z, Bian S, et al., 2022, Culture of patient-derived multicellular clusters in suspended hydrogel capsules for pre-clinical personalized drug screening. Bioact Mater, 18: 164–177. https://doi.org/10.1016/j.bioactmat.2022.03.020
Yu X, Zhu D, Luo B, et al., 2022, IFNγ enhances ferroptosis by increasing JAK STAT pathway activation to suppress SLCA711 expression in adrenocortical carcinoma. Oncol Rep, 47(5): 97. https://doi.org/10.3892/or.2022.8308
Nika MC, Ntaiou K, Elytis K, et al., 2020, Wide-scope target analysis of emerging contaminants in landfill leachates and risk assessment using Risk Quotient methodology. J Hazard Mater, 394: 122493. https://doi.org/10.1016/j.jhazmat.2020.122493
Xia W, Zhu J, Wang X, et al., 2020, Overexpression of Foxc1 regenerates crushed rat facial nerves by promoting Schwann cells migration via the Wnt/β-catenin signaling pathway. J Cell Phys, 235(12): 9609–9622. https://doi.org/10.1002/jcp.29772
Rao F, Wang Y, Zhang D, et al., 2020, Aligned chitosan nanofiber hydrogel grafted with peptides mimicking bioactive brain-derived neurotrophic factor and vascular endothelial growth factor repair long-distance sciatic nerve defects in rats. Theranostics, 10(4): 1590–1603. https://doi.org/10.7150/thno.36272
Torres-Mejía E, Trümbach D, Kleeberger C, et al., 2020, Sox2 controls Schwann cell self-organization through fibronectin fibrillogenesis. Sci Rep, 10(1): 1984. https://doi.org/10.1038/s41598-019-56877-y
Riggio C, Calatayud MP, Hoskins C, et al., 2012, Poly-l-lysine-coated magnetic nanoparticles as intracellular actuators for neural guidance. Int J Nanomed, 7: 3155–3166. https://doi.org/10.2147/ijn.S28460
Cai J, Peng X, Nelson KD, et al., 2005, Permeable guidance channels containing microfilament scaffolds enhance axon growth and maturation. J Biomed Mater Res A, 75(2): 374–386. https://doi.org/10.1002/jbm.a.30432
Puhl DL, Funnell JL, D’Amato AR, et al., 2020, Aligned fingolimod-releasing electrospun fibers increase dorsal root ganglia neurite extension and decrease schwann cell expression of promyelinating factors. Front Bioeng Biotechnol, 8: 937. https://doi.org/10.3389/fbioe.2020.00937
Morano M, Wrobel S, Fregnan F, et al., 2014, Nanotechnology versus stem cell engineering: In vitro comparison of neurite inductive potentials. Int J Nanomed, 9: 5289–5306. https://doi.org/10.2147/ijn.S71951
Li M, Tang Z, Lv S, et al., 2014, Cisplatin crosslinked pH-sensitive nanoparticles for efficient delivery of doxorubicin. Biomaterials, 35(12): 3851–3864. https://doi.org/10.1016/j.biomaterials.2014.01.018
Cao F, Ju E, Zhang Y, et al., 2017, An efficient and benign antimicrobial depot based on silver-infused MoS(2). ACS Nano, 11(5): 4651–4659. https://doi.org/10.1021/acsnano.7b00343
Xu Y, Zhou J, Liu C, et al., 2021, Understanding the role of tissue-specific decellularized spinal cord matrix hydrogel for neural stem/progenitor cell microenvironment reconstruction and spinal cord injury. Biomaterials, 268: 120596. https://doi.org/10.1016/j.biomaterials.2020.120596
Alvarsson A, Jimenez-Gonzalez M, Li R, et al., 2020, A 3D atlas of the dynamic and regional variation of pancreatic innervation in diabetes. Sci Adv, 6(41): 9124. https://doi.org/10.1126/sciadv.aaz9124
Asbury AK, King RH, Reilly MM, et al., 2011, Professor P. K. Thomas: clinician, investigator, editor and leader--a retrospective appreciation. Brain, 134(Pt 2): 618–626. https://doi.org/10.1093/brain/awq230
Afshari FT, Kwok JC, White L, et al., 2010, Schwann cell migration is integrin-dependent and inhibited by astrocyte-produced aggrecan. Glia, 58(7): 857–869. https://doi.org/10.1002/glia.20970
Benoy V, Van Helleputte L, Prior R, et al., 2018, HDAC6 is a therapeutic target in mutant GARS-induced Charcot- Marie-Tooth disease. Brain, 141(3): 673–687. https://doi.org/10.1093/brain/awx375
Hu H, Jonas P, 2014, A supercritical density of Na(+) channels ensures fast signaling in GABAergic interneuron axons. Nat Neurosci, 17(5): 686–693. https://doi.org/10.1038/nn.3678
Eichel MA, Gargareta VI, D’Este E, et al., 2020, CMTM6 expressed on the adaxonal Schwann cell surface restricts axonal diameters in peripheral nerves. Nat Commun, 11(1): 4514. https://doi.org/10.1038/s41467-020-18172-7
Wang L, Lu C, Yang S, et al., 2020, A fully biodegradable and self-electrified device for neuroregenerative medicine. Sci Adv, 6(50): 6686. https://doi.org/10.1126/sciadv.abc6686
Jin B, Yu Y, Chen X, et al., 2023, Microtubes with gradient decellularized porcine sciatic nerve matrix from microfluidics for sciatic nerve regeneration. Bioact Mater, 21: 511–519. https://doi.org/10.1016/j.bioactmat.2022.08.027
Mayoral I, Bevilacqua E, Gómez G, et al., 2022, Tissue engineered in-vitro vascular patch fabrication using hybrid 3D printing and electrospinning. Mater Today Bio, 14: 100252. https://doi.org/10.1016/j.mtbio.2022.100252
Fan D, Yuan X, Wu W, et al., 2022, Self-shrinking soft demoulding for complex high-aspect-ratio microchannels. Nat Commun, 13(1): 5083. https://doi.org/10.1038/s41467-022-32859-z
Liu Y, Dabrowska C, Mavousian A, et al., 2021, Bio-assembling macro-scale, lumenized airway tubes of defined shape via multi-organoid patterning and fusion. Adv Sci, 8(9): 2003332. https://doi.org/10.1002/advs.202003332
Farhat W, Chatelain F, Marret A, et al., 2021, Trends in 3D bioprinting for esophageal tissue repair and reconstruction. Biomaterials, 267: 120465. https://doi.org/10.1016/j.biomaterials.2020.120465
Chen S, Li R, Li X, et al., 2018, Electrospinning: An enabling nanotechnology platform for drug delivery and regenerative medicine. Adv Drug Deliv Rev, 132: 188–213. https://doi.org/10.1016/j.addr.2018.05.001
Yao Z, Yuan W, Xu J, et al., 2022, Magnesium-encapsulated injectable hydrogel and 3D-engineered polycaprolactone conduit facilitate peripheral nerve regeneration. Adv Sci, 9(21): e2202102. https://doi.org/10.1002/advs.202202102
Zhu M, Li W, Dong X, et al., 2019, In vivo engineered extracellular matrix scaffolds with instructive niches for oriented tissue regeneration. Nat Commun, 10(1): 4620. https://doi.org/10.1038/s41467-019-12545-3
Qian Y, Zhao X, Han Q, et al., 2018, An integrated multi-layer 3D-fabrication of PDA/RGD coated graphene loaded PCL nanoscaffold for peripheral nerve restoration. Nat Commun, 9(1): 323. https://doi.org/10.1038/s41467-017-02598-7
Cui X, Jing J, Wu R, et al., 2021, Adipose tissue-derived neurotrophic factor 3 regulates sympathetic innervation and thermogenesis in adipose tissue. Nat Commun, 12(1): 5362. https://doi.org/10.1038/s41467-021-25766-2
Han Q, Ordaz JD, Liu NK, et al., 2019, Descending motor circuitry required for NT-3 mediated locomotor recovery after spinal cord injury in mice. Nat Commun, 10(1): 5815. https://doi.org/10.1038/s41467-019-13854-3
Takano T, Wu M, Nakamuta S, et al., 2017, Discovery of long-range inhibitory signaling to ensure single axon formation. Nat Commun, 8(1): 33. https://doi.org/10.1038/s41467-017-00044-2
Lai BQ, Wang JM, Ling EA, et al., 2014, Graft of a tissue-engineered neural scaffold serves as a promising strategy to restore myelination after rat spinal cord transection. Stem Cells Dev, 23(8): 910–921. https://doi.org/10.1089/scd.2013.0426
Yoshimura T, Kawano Y, Arimura N, et al., 2005, GSK- 3beta regulates phosphorylation of CRMP-2 and neuronal polarity. Cell, 120(1): 137–149:. https://doi.org/10.1016/j.cell.2004.11.012
Liu D, Shu G, Jin F, et al., 2020, ROS-responsive chitosan- SS31 prodrug for AKI therapy via rapid distribution in the kidney and long-term retention in the renal tubule. Sci Adv, 6(41): 7422. https://doi.org/10.1126/sciadv.abb7422
Chan LW, Kim CH, Wang X, et al., 2016, PolySTAT-modified chitosan gauzes for improved hemostasis in external hemorrhage. Acta Biomater, 31: 178–185. https://doi.org/10.1016/j.actbio.2015.11.017
Zakhem E, Raghavan S, Bitar KN, 2014, Neo-innervation of a bioengineered intestinal smooth muscle construct around chitosan scaffold. Biomaterials, 35(6): 1882–1889. https://doi.org/10.1016/j.biomaterials.2013.11.049
Li J, Shu Y, Hao T, et al., 2013, A chitosan-glutathione based injectable hydrogel for suppression of oxidative stress damage in cardiomyocytes. Biomaterials, 34(36): 9071–9081. https://doi.org/10.1016/j.biomaterials.2013.08.031
Lu Q, Zhang F, Cheng W, et al., 2021, Nerve guidance conduits with hierarchical anisotropic architecture for peripheral nerve regeneration. Adv Healthc Mater, 10(14): e2100427. https://doi.org/10.1002/adhm.202100427
Hsueh YY, Chang YJ, Huang TC, et al., 2014, Functional recoveries of sciatic nerve regeneration by combining chitosan-coated conduit and neurosphere cells induced from adipose-derived stem cells. Biomaterials, 35(7): 2234– 2244. https://doi.org/10.1016/j.biomaterials.2013.11.081
Mekhail M, Almazan G, Tabrizian M, 2015, Purine-crosslinked injectable chitosan sponges promote oligodendrocyte progenitor cells’ attachment and differentiation. Biomater Sci, 3(2): 279–287. https://doi.org/10.1039/c4bm00215f
Li X, Yang Z, Zhang A, 2009, The effect of neurotrophin-3/ chitosan carriers on the proliferation and differentiation of neural stem cells. Biomaterials, 30(28): 4978–4985. https://doi.org/10.1016/j.biomaterials.2009.05.047
Liu K, Yan L, Li R, et al., 2022, 3D printed personalized nerve guide conduits for precision repair of peripheral nerve defects. Adv Sci, 9(12): e2103875. https://doi.org/10.1002/advs.202103875
Chen S, John JV, McCarthy A, et al., 2020, Fast transformation of 2D nanofiber membranes into pre-molded 3D scaffolds with biomimetic and oriented porous structure for biomedical applications. Appl Phys Rev, 7(2): 021406. https://doi.org/10.1063/1.5144808
Joung D, Lavoie NS, Guo SZ, et al., 2020, 3D printed neural regeneration devices. Adv Funct Mater, 30(1): 10. https://doi.org/10.1002/adfm.201906237