Evolution of bioprinting and current applications
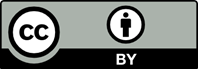
Bioprinting is a very useful tool that has a huge application potential in different fields of science and biotechnology. In medicine, advances in bioprinting are focused on the printing of cells and tissues for skin regeneration and the manufacture of viable human organs, such as hearts, kidneys, and bones. This review provides a chronological overview of some of the most relevant developments of bioprinting technique and its current status. A search was carried out in SCOPUS, Web of Science, and PubMed databases, and a total of 31,603 papers were found, of which 122 were finally chosen for analysis. These articles cover the most important advances in this technique at the medical level, its applications, and current possibilities. Finally, the paper ends with conclusions about the use of bioprinting and our expectations of this technique. This paper presents a review on the tremendous progress of bioprinting from 1998 to the present day, with many promising results indicating that our society is getting closer to achieving the total reconstruction of damaged tissues and organs and thus solving many healthcare-related problems, including the shortage of organ and tissue donors.
Ozbolat IT, Yu Y, 2013, Bioprinting toward organ fabrication: Challenges and future trends. IEEE Trans Biomed Eng, 60(3): 691–699. http://doi.org/10.1109/TBME.2013.2243912.
Park A, Wu B, Griffith LG, 1998, Integration of surface modification and 3D fabrication techniques to prepare patterned poly(L-lactide) substrates allowing regionally selective cell adhesion. J Biomater Sci Polym Ed, 9(2): 89–110. http://doi.org/10.1163/156856298X00451.
Kim SS, Utsunomiya H, Koksi JA, et al., 1998, Survival and function of hepatocytes on a novel three-dimensional synthetic biodegradable polymer scaffold with an intrinsic network of channels. Ann Surg, 228(1): 8. http://doi.org/10.1097/00000658-199807000-00002.
Bhatia SN, Chen CS, 1999, Tissue engineering at the micro-scale. Biomed Microdevices, 2(2): 131–144. http://doi.org/10.1023/A:1009949704750.
Vozzi G, Ahluwalia A, de Rossi D, et al., 2000, Microsyringe based fabrication of high resolution organic structures for bioengineering applications. Proceedings of the First Annual International IEEE-EMBS Special Topic Conference on Microtechnologies in Medicine and Biology, 141–145.
Quirk RA, Briggs D, Davies MC, et al., 2001, Characterization of the spatial distributions of entrapped polymers following the surface engineering of poly(lactic acid). Surf Interface Anal, 31(1): 46–50. http://doi.org/10.1002/SIA.951.
Zeltinger J, Sherwood JK, Graham DA, et al., 2001, Effect of pore size and void fraction on cellular adhesion, proliferation, and matrix deposition. Tissue Eng, 7(5): 557–572. http://doi.org/10.1089/107632701753213183.
Ringeisen BR, Chrisey DB, Krizman DB, et al., 2002, Cell-by-cell construction of living tissue by ambient laser transfer. Proceedings of the Second Annual International IEEE-EMBS Special Topic Conference on Microtechnologies in Medicine and Biology, 120–125. http://doi.org/10.1109/MMB.2002.1002277.
Mironov V, Boland T, Trusk T, et al., 2003, Organ printing: Computer-aided jet-based 3D tissue engineering. Trends Biotechnol, 21(4): 157–161. http://doi.org/10.1016/S0167-7799(03)00033-7.
Boland T, Mironov V, Gutowska A, et al., 2003, Cell and organ printing 2: Fusion of cell aggregates in three-dimensional gels. Anat Rec, 272(2): 497–502. http://doi.org/10.1002/AR.A.10059.
ben Hsieh H, Fitch J, White D, et al., 2004, Ultra-high-throughput microarray generation and liquid dispensing using multiple disposable piezoelectric ejectors. J Biomol Screen, 9(2): 85–94. http://doi.org/10.1177/1087057103260943.
Pfister A, Landers R, Laib A, et al., 2004, Biofunctional rapid prototyping for tissue-engineering applications: 3D bioplotting versus 3D printing. J Polym Sci A Polym Chem, 42(3): 624–638. http://doi.org/10.1002/POLA.10807.
Jakab K, Neagu A, Mironov V, et al., 2004, Engineering biological structures of prescribed shape using self-assembling multicellular systems. Proc Natl Acad Sci U S A, 101(9): 2864. http://doi.org/10.1073/PNAS.0400164101.
Ackermann J, Videlot C, Nguyen TN, et al., 2004, Gel-layer-assisted directional electropolymerization: A versatile method for high-resolution volume and surface patterning of flexible substrates with conjugated polymers. Adv Mater, 16(19): 1709–1712. http://doi.org/10.1002/ADMA.200306705.
Fridman G, Li M, Friedman G, et al., 2004, Non-thermal plasma bioPrinter with nano-scale precision. Proceedings of the IEEE International Conference on Plasma Science, 170. http://doi.org/10.1109/PLASMA.2004.1339724.
Hackney PM, Pancholi KP, 2004, Application of the Z-Corps three-dimensional printing processes using novel material to manufacture bio-scaffold for bone replacement. Proceedings of the Fifth National Conference on Rapid Design, Prototyping, and Manufacturing, 53–60.
Komatsu H, Iwasawa N, Citterio D, et al., 2004, Design and synthesis of highly sensitive and selective fluorescein-derived magnesium fluorescent probes and application to intracellular 3D Mg2+ imaging. J Am Chem Soc, 126(50): 16353–16360. http://doi.org/10.1021/JA049624L.
Ball P, 2005, Body painting. Nat Mater, 4(8): 582. http://doi.org/10.1038/nmat1439.
Mironov V, 2005, The Second International Workshop on bioprinting, biopatterning and bioassembly. Expert Opin Biol Ther, 5(8): 1111–1115. http://doi.org/10.1517/14712598.5.8.1111.
. Leukers B, Gülkan H, Irsen SH, et al., 2005, Biocompatibility of ceramic scaffolds for bone replacement made by 3D printing. Materwiss Werksttech, 36(12): 781–787. http://doi.org/10.1002/MAWE.200500968.
Leukers B, Gülkan H, Irsen SH, et al., 2005, Hydroxyapatite scaffolds for bone tissue engineering made by 3D printing. J Mater Sci Mater Med, 16(12): 1121–1124. http://doi.org/10.1007/S10856-005-4716-5.
Chang R, Starly B, Sun W, et al., 2006, Freeform bioprinting of liver encapsulated in alginate hydrogels tissue constructs for pharmacokinetic study. Proceedings of the 2006 International Solid Freeform Fabrication Symposium.
Mironov V, Reis N, Derby B, 2006, Review: Bioprinting: A beginning. Tissue Eng, 12(4): 631–634. http://doi.org/10.1089/TEN.2006.12.631.
Mironov V, 2006, Toward human organ printing: Charleston Bioprinting Symposium. ASAIO J, 52(6): 27–30. http://doi.org/10.1097/01.MAT.0000248999.25334.6A.
Fakhrzadeh H, Larikani B, 2007, Bioprinting: Prospects of a rapidly growing technology. J Diabetes Lipid Disord, 7(1): 1–7.
Mironov V, Kasyanov V, Markwald R, 2007, Bioprinting: Directed tissue self-assembly. Chem Eng Prog, 103: S12–S17.
IEEE, 2007, 2007 International Symposium on VLSI Technology, Systems and Applications (VLSI-TSA), Hsinchu, Taiwan IEEE. http://lib.ugent.be/catalog/ebk01:1000000000525455
Suwanprateeb J, Sanngam R, Suwanpreuk W, 2008, Fabrication of bioactive hydroxyapatite/bis-GMA based composite via three dimensional printing. J Mater Sci Mater Med, 19(7): 2637–2645. http://doi.org/10.1007/S10856-007-3362-5.
Igawa K, Chung U-i, Tei Y, 2008, Custom-made artificial bones fabricated by an inkjet printing technology. Clin Calcium, 18(12): 1737–1743. http://doi.org/clica081217371743.
Sachlos E, Wahl DA, Triffitt JT, et al., 2008, The impact of critical point drying with liquid carbon dioxide on collagen-hydroxyapatite composite scaffolds. Acta Biomater, 4(5): 1322–1331. http://doi.org/10.1016/J.ACTBIO.2008.03.016.
Fedorovich NE, de Wijn JR, Verbout AJ, et al., 2008, Three-dimensional fiber deposition of cell-laden, viable, patterned constructs for bone tissue printing. Tissue Eng Part A, 14(1): 127–133. http://doi.org/10.1089/TEN.A.2007.0158.
Ryan GE, Pandit AS, Apatsidis DP, 2008, Porous titanium scaffolds fabricated using a rapid prototyping and powder metallurgy technique. Biomaterials, 29(27): 3625–3635. http://doi.org/10.1016/J.BIOMATERIALS.2008.05.032.
Fierz FC, Beckmann F, Huser M, et al., The morphology of anisotropic 3D-printed hydroxyapatite scaffolds. Biomaterials, 29(28): 3799–3806. http://doi.org/10.1016/J.BIOMATERIALS.2008.06.012.
Wu W, Zheng Q, Guo X, et al., 2009, A programmed release multi-drug implant fabricated by three-dimensional printing technology for bone tuberculosis therapy. Biomed Mater, 4(6): 065005. http://doi.org/10.1088/1748-6041/4/6/065005.
Nishiyama Y, Nakamura M, Henmi C, et al., 2009, Development of a three-dimensional bioprinter: Construction of cell supporting structures using hydrogel and state-of-the-art inkjet technology. J Biomech Eng, 131(3): 035001. http://doi.org/10.1115/1.3002759.
Lee W, Debasitis JC, Lee VK, et al., 2009, Multi-layered culture of human skin fibroblasts and keratinocytes through three-dimensional freeform fabrication. Biomaterials, 30(8): 1587–1595. http://doi.org/10.1016/J.BIOMATERIALS.2008.12.009.
Barry RA, Shepherd R, Hanson JN, et al., 2009, Direct-write assembly of 3D hydrogel scaffolds for guided cell growth. Adv Mater, 21(23): 2407–2410. http://doi.org/10.1002/ADMA.200803702.
Fedorovich NE, Swennen I, Girones J, et al., 2009, Evaluation of photocrosslinked Lutrol hydrogel for tissue printing applications. Biomacromolecules, 10(7): 1689–1696. http://doi.org/10.1021/BM801463Q.
Cui X, Boland T, 2009, Human microvasculature fabrication using thermal inkjet printing technology. Biomaterials, 30(31): 6221–6227. http://doi.org/10.1016/J.BIOMATERIALS.2009.07.056.
Norotte C, Marga FS, Niklason LE, et al., 2009, Scaffold-free vascular tissue engineering using bioprinting. Biomaterials, 30(30): 5910–5917. http://doi.org/10.1016/J.BIOMATERIALS.2009.06.034.
Guillemot F, Souquet A, Catros S, et al., 2010, High-throughput laser printing of cells and biomaterials for tissue engineering. Acta Biomater, 6(7): 2494–2500. http://doi.org/10.1016/J.ACTBIO.2009.09.029.
Nakamura M, Iwanaga S, Henmi C, et al., 2010, Biomatrices and biomaterials for future developments of bioprinting and biofabrication. Biofabrication, 2(1): 014110. http://doi.org/10.1088/1758-5082/2/1/014110.
Lee W, Lee V, Polio S, et al., 2010, On-demand three-dimensional freeform fabrication of multi-layered hydrogel scaffold with fluidic channels. Biotechnol Bioeng, 105(6): 1178–1186. http://doi.org/10.1002/BIT.22613.
Chang R, Emami K, Wu H, et al., 2010, Biofabrication of a three-dimensional liver micro-organ as an in vitro drug metabolism model. Biofabrication, 2(4): 045004. http://doi.org/10.1088/1758-5082/2/4/045004.
Arai K, Iwanaga S, Toda H, et al., 2011, Three-dimensional inkjet biofabrication based on designed images. Biofabrication, 3(3): 034113. http://doi.org/10.1088/1758-5082/3/3/034113.
Skardal A, Mack D, Kapetanovic E, et al., 2012, Bioprinted amniotic fluid-derived stem cells accelerate healing of large skin wounds. Stem Cells Transl Med, 1(11): 792–802. http://doi.org/10.5966/SCTM.2012-0088.
Miller JS, Stevens KR, Yang MT, et al., 2012, Rapid casting of patterned vascular networks for perfusable engineered three-dimensional tissues. Nat Mater, 11(9): 768–774. http://doi.org/10.1038/NMAT3357.
Miller JS, Stevens KR, Yang MT, et al, 2012, Effects of silica and zinc oxide doping on mechanical and biological properties of 3D printed tricalcium phosphate tissue engineering scaffolds. Dent Mater, 28(2): 113–122. http://doi.org/10.1016/J.DENTAL.2011.09.010.
Hockaday LA, Kang KH, Colangelo NW, et al., 2012, Rapid 3D printing of anatomically accurate and mechanically heterogeneous aortic valve hydrogel scaffolds. Biofabrication, 4(3): 035005. http://doi.org/10.1088/1758-5082/4/3/035005.
Zopf DA, Hollister SJ, Nelson ME, et al., 2013, Bioresorbable airway splint created with a three-dimensional printer. N Engl J Med, 368(21): 2043–2045. http://doi.org/10.1056/NEJMC1206319.
Xu T, Zhao W, Zhu JM, et al., 2013, Complex heterogeneous tissue constructs containing multiple cell types prepared by inkjet printing technology. Biomaterials, 34(1): 130–139. http://doi.org/10.1016/J.BIOMATERIALS.2012.09.035.
Michael S, Sorg H, Peck CT, et al., 2013, Tissue engineered skin substitutes created by laser-assisted bioprinting form skin-like structures in the dorsal skin fold chamber in mice. PLoS One, 8(3): 0057741. http://doi.org/10.1371/JOURNAL.PONE.0057741.
Duan B, Hockaday LA, Kang KH, et al., 2013, 3D bioprinting of heterogeneous aortic valve conduits with alginate/gelatin hydrogels. J Biomed Mater Res A, 101(5): 1255–1264. http://doi.org/10.1002/JBM.A.34420.
Schuurman W, Levett PA, Pot MW, et al., 2013, Gelatin-methacrylamide hydrogels as potential biomaterials for fabrication of tissue-engineered cartilage constructs. Macromol Biosci, 13(5): 551–561. http://doi.org/10.1002/MABI.201200471.
Mannoor MS, Jiang Z, James T, et al., 2013, 3D printed bionic ears. Nano Lett, 13(6): 2634–2639. http://doi.org/10.1021/NL4007744.
Lee VK, Lanzi AM, Ngo H, et al., 2014, Generation of multi-scale vascular network system within 3D hydrogel using 3D bio-printing technology. Cell Mol Bioeng, 7(3): 460–472. http://doi.org/10.1007/S12195-014-0340-0.
Lee VK, Kim DY, Ngo H, et al., 2014, Creating perfused functional vascular channels using 3D bio-printing technology. Biomaterials, 35(28): 8092–8102. http://doi.org/10.1016/J.BIOMATERIALS.2014.05.083.
Kolesky DB, Truby RL, Gladman AS, et al., 2014, 3D bioprinting of vascularized, heterogeneous cell-laden tissue constructs. Adv Mater, 26(19): 3124–3130. http://doi.org/10.1002/ADMA.201305506.
Zhao Y, Yao R, Ouyang L, et al., 2014, Three-dimensional printing of Hela cells for cervical tumor model in vitro. Biofabrication, 6(3): 035001. http://doi.org/10.1088/1758-5082/6/3/035001.
Billiet T, Gevaert E, de Schryver T, et al., 2014, The 3D printing of gelatin methacrylamide cell-laden tissue-engineered constructs with high cell viability. Biomaterials, 35(1): 49–62. http://doi.org/10.1016/J.BIOMATERIALS.2013.09.078.
Pati F, Jang J, Ha DH, et al., 2014, Printing three-dimensional tissue analogues with decellularized extracellular matrix bioink. Nat Commun, 5(1): 1–11. http://doi.org/10.1038/ncomms4935.
Visser J, Melchels FPW, Jeon JE, et al., 2015, Reinforcement of hydrogels using three-dimensionally printed microfibres. Nat Commun, 6(1): 1–10. http://doi.org/10.1038/ncomms7933.
Jakus AE, Secor EB, Rutz AL, et al., 2015, Three-dimensional printing of high-content graphene scaffolds for electronic and biomedical applications. ACS Nano, 9(4): 4636–4648. http://doi.org/10.1021/ACSNANO.5B01179.
Markstedt K, Mantas A, Tournier I, et al., 2015, 3D bioprinting human chondrocytes with nanocellulose-alginate bioink for cartilage tissue engineering applications. Biomacromolecules, 16(5): 1489–1496. http://doi.org/10.1021/ACS.BIOMAC.5B00188/ASSET/ IMAGES/LARGE/BM-2015-00188Z_0007.JPEG.
Hong S, Sycks D, Fai Chan H, et al., 2015, 3D printing of highly stretchable and tough hydrogels into complex, cellularized structures. Adv Mater, 27(27): 4035–4040. http://doi.org/10.1002/ADMA.201501099.
Lozano R, Stevens L, Thompson BC, et al., 2015, 3D printing of layered brain-like structures using peptide modified gellan gum substrates. Biomaterials, 67: 264–273. http://doi.org/10.1016/J.BIOMATERIALS.2015.07.022.
Yue K, Trujillo-de Santiago G, Alvarez MM, et al., 2015, Synthesis, properties, and biomedical applications of gelatin methacryloyl (GelMA) hydrogels. Biomaterials, 73: 254–271. http://doi.org/10.1016/J.BIOMATERIALS.2015.08.045.
Kang HW, Lee SJ, Ko IK, et al., 2016, A 3D bioprinting system to produce human-scale tissue constructs with structural integrity. Nat Biotechnol, 34(3): 312–319. http://doi.org/10.1038/nbt.3413.
Kolesky DB, Homan KA, Skylar-Scott MA, et al., 2016, Three-dimensional bioprinting of thick vascularized tissues. Proc Natl Acad Sci U S A, 113(12): 3179–3184. http://doi.org/10.1073/PNAS.1521342113.
Mandrycky C, Wang Z, Kim K, et al., 2016, 3D bioprinting for engineering complex tissues. Biotechnol Adv, 34(4): 422–434. http://doi.org/10.1016/J.BIOTECHADV.2015.12.011.
Jia W, Gungor-Ozkerim PS, Zhang YS, et al., 2016, Direct 3D bioprinting of perfusable vascular constructs using a blend bioink. Biomaterials, 106: 58–68. http://doi.org/10.1016/J.BIOMATERIALS.2016.07.038.
Ma X, Qu X, Zhu W, et al., 2016, Deterministically patterned biomimetic human iPSC-derived hepatic model via rapid 3D bioprinting. Proc Natl Acad Sci U S A, 113(8): 2206–2211. http://doi.org/10.1073/PNAS.1524510113.
Homan KA, Kolesky DB, Skylar-Scott MA, et al., 2016, Bioprinting of 3D convoluted renal proximal tubules on perfusable chips. Sci Rep, 6(1): 1–13. http://doi.org/10.1038/srep34845.
Bhise NS, Manoharan V, Massa S, et al., 2016, A liver-on-a-chip platform with bioprinted hepatic spheroids. Biofabrication, 8(1): 014101. http://doi.org/10.1088/1758-5090/8/1/014101.
Guo F, Mao Z, Chen Y, et al., 2016, Three-dimensional manipulation of single cells using surface acoustic waves. Proc Natl Acad Sci U S A, 113(6): 1522–1527. http://doi.org/10.1073/PNAS.1524813113.
Lind JU, Busbee TA, Valentine AD, et al., 2017, Instrumented cardiac microphysiological devices via multi-material 3D printing. Nat Mater, 16(3): 303. http://doi.org/10.1038/NMAT4782.
Jang J, Park HJ, Kim SW, et al., 2017, 3D printed complex tissue construct using stem cell-laden decellularized extracellular matrix bioinks for cardiac repair. Biomaterials, 112: 264–274. http://doi.org/10.1016/J.BIOMATERIALS.2016.10.026.
Yao Q, Cosme JGL, Xu T, et al., 2017, Three dimensional electrospun PCL/PLA blend nanofibrous scaffolds with significantly improved stem cells osteogenic differentiation and cranial bone formation. Biomaterials, 115: 115–127. http://doi.org/10.1016/J.BIOMATERIALS.2016.11.018.
Laronda MM, Rutz AL, Xiao S, et al., 2017, A bioprosthetic ovary created using 3D printed microporous scaffolds restores ovarian function in sterilized mice. Nat Commun, 8(1): 15261. http://doi.org/10.1038/NCOMMS15261.
Schaffner M, Rühs PA, Coulter F, et al., 2017, 3D printing of bacteria into functional complex materials. Sci Adv, 3(12): eaao6804. http://doi.org/10.1126/SCIADV.AAO6804.
Bulanova EA, Koudan EV, Degosserie J, et al., 2017, Bioprinting of a functional vascularized mouse thyroid gland construct. Biofabrication, 9(3): 034105. http://doi.org/10.1088/1758-5090/AA7FDD.
Turnbull G, Clarke J, Picard F, et al., 2017, 3D bioactive composite scaffolds for bone tissue engineering. Bioact Mater, 3(3): 278–314. http://doi.org/10.1016/J.BIOACTMAT.2017.10.001.
Gopinathan J, Noh I, 2018, Recent trends in bioinks for 3D printing. Biomater Res, 22(1). http://doi.org/10.1186/S40824-018-0122-1.
Gao T, Gillipsie GJ, Copus JS, et al., 2018, Optimization of gelatin-alginate composite bioink printability using rheological parameters: A systematic approach. Biofabrication, 10(3): 034106. http://doi.org/10.1088/1758-5090/AACDC7.
Hoarau-Véchot J, Rafii A, Touboul C, et al., 2018, Halfway between 2D and animal models: Are 3D cultures the ideal tool to study cancer-microenvironment interactions? Int J Mol Sci, 19(1): 181. http://doi.org/10.3390/IJMS19010181.
Kim BS, Kwon YW, Kong JS, et al., 2018, 3D cell printing of in vitro stabilized skin model and in vivo pre-vascularized skin patch using tissue-specific extracellular matrix bioink: A step towards advanced skin tissue engineering. Biomaterials, 168: 38–53. http://doi.org/10.1016/J.BIOMATERIALS.2018.03.040.
Qian Y, Zhao X, Han Q, et al., 2018, An integrated multi-layer 3D-fabrication of PDA/RGD coated graphene loaded PCL nanoscaffold for peripheral nerve restoration. Nat Commun, 9(1): 1–16. http://doi.org/10.1038/s41467-017-02598-7.
Isaacson A, Swioklo S, Connon CJ, 2018, 3D bioprinting of a corneal stroma equivalent. Exp Eye Res, 173: 188–193. http://doi.org/10.1016/J.EXER.2018.05.010.
Joung D, Truong V, Neitzke CC, et al., 2018, 3D printed stem-cell derived neural progenitors generate spinal cord scaffolds. Adv Funct Mater, 28(39): 1801850. http://doi.org/10.1002/ADFM.201801850.
Lee A, Hudson AR, Shiwarski DJ, et al., 2019, 3D bioprinting of collagen to rebuild components of the human heart. Science, 365(6452): 482–487. http://doi.org/10.1126/SCIENCE.AAV9051.
Noor N, Shapira A, Edri R, et al., 2019, 3D printing of personalized thick and perfusable cardiac patches and hearts. Adv Sci, 6(11): 1900344. http://doi.org/10.1002/ADVS.201900344.
Koffler J, Zhu W, Qu X, et al., 2019, Biomimetic 3D-printed scaffolds for spinal cord injury repair. Nat Med, 25(2): 263–269. http://doi.org/10.1038/S41591-018-0296-Z.
Yi HG, Jeong YH, Kim Y, et al., 2019, A bioprinted human-glioblastoma-on-a-chip for the identification of patient-specific responses to chemoradiotherapy. Nat Biomed Eng, 3(7): 509–519. http://doi.org/10.1038/S41551-019-0363-X.
Albanna M, Binder KW, Murphy SV, et al., 2019, In situ bioprinting of autologous skin cells accelerates wound healing of extensive excisional full-thickness wounds. Sci Rep, 9(1): 1856. http://doi.org/10.1038/S41598-018-38366-W.
Chen P, Zheng L, Wang Y, et al., 2019, Desktop-stereolithography 3D printing of a radially oriented extracellular matrix/ mesenchymal stem cell exosome bioink for osteochondral defect regeneration. Theranostics, 9(9): 2439–2459. http://doi.org/10.7150/THNO.31017.
Choi YJ, Jun YJ, Kim DY, et al., 2019, A 3D cell printed muscle construct with tissue-derived bioink for the treatment of volumetric muscle loss. Biomaterials, 206: 160–169. http://doi.org/10.1016/J.BIOMATERIALS.2019.03.036.
Wan Z, Zhang P, Liu Y, et al., 2020, Four-dimensional bioprinting: Current developments and applications in bone tissue engineering. Acta Biomater, 101: 26–42. http://doi.org/10.1016/J.ACTBIO.2019.10.038.
Pan S, Yin J, Yu L, et al., 2020, 2D MXene‐integrated 3D‐printing scaffolds for augmented osteosarcoma phototherapy and accelerated tissue reconstruction. Adv Sci, 7(2): 1901511. http://doi.org/10.1002/ADVS.201901511.
Datta P, Dey M, Ataie Z, et al., 2020, 3D bioprinting for reconstituting the cancer microenvironment. NPJ Precis Oncol, 4(1): 18. http://doi.org/10.1038/S41698-020-0121-2.
Zarrintaj P, Ramsey JD, Samadi A, et al., 2020, Poloxamer: A versatile tri-block copolymer for biomedical applications. Acta Biomater, 110: 37–67. http://doi.org/10.1016/J.ACTBIO.2020.04.028.
Sun Y, You Y, Jiang W, et al., 2020, Generating ready-to-implant anisotropic menisci by 3D-bioprinting protein-releasing cell-laden hydrogel-polymer composite scaffold. Appl Mater Today, 18: 100469. http://doi.org/10.1016/J.APMT.2019.100469.
Dong H, Hu B, Zhang W, et al., 2023, Robotic-assisted automated in situ bioprinting. Int J Bioprint, 9(1): 98–108. http://doi.org/10.18063/IJB.V9I1.629.
Klak M, Kosowska K, Bryniarski T, et al., 2022, Bioink based on the dECM for 3D bioprinting of bionic tissue, the first results obtained on murine model. Bioprinting, 28(1): e00233. http://doi.org/10.1016/J.BPRINT.2022.E00233.
Pavan Kalyan B, Kumar L, 2022, 3D printing: Applications in tissue engineering, medical devices, and drug delivery. AAPS PharmSciTech, 23(4): 92. http://doi.org/10.1208/S12249-022-02242-8.
Murphy Sv, Atala A, 2014, 3D bioprinting of tissues and organs. Nat Biotechnol, 32(8): 773–785. http://doi.org/10.1038/NBT.2958.
Ingber DE, Mow VC, Butler D, et al., 2006, Tissue engineering and developmental biology: Going biomimetic. Tissue Eng, 12(12): 3265–3283. http://doi.org/10.1089/TEN.2006.12.3265.
Derby B, 2012, Printing and prototyping of tissues and scaffolds. Science, 338(6109): 921–926. http://doi.org/10.1126/SCIENCE.1226340.
Kelm JM, Lorber V, Snedeker JG, et al., 2010, A novel concept for scaffold-free vessel tissue engineering: Self-assembly of microtissue building blocks. J Biotechnol, 148(1): 46–55.
http://doi.org/10.1016/J.JBIOTEC.2010.03.002.
Alajati A, Laib AM, Weber H, et al., 2008, Spheroid-based engineering of a human vasculature in mice. Nat Methods, 5(5): 439–445. http://doi.org/10.1038/NMETH.1198.
Gao G, Ahn M, Cho WW, et al., 2021, 3D printing of pharmaceutical application: Drug screening and drug delivery. Pharmaceutics, 13(9): 1373. http://doi.org/10.3390/PHARMACEUTICS13091373.
Vaidya M, 2015, Startups tout commercially 3D-printed tissue for drug screening. Nat Med, 21(1): 2. http://doi.org/10.1038/NM0115-2.
Zhang B, Korolj A, Lai BFL, et al., Advances in organ-on-a-chip engineering. Nat Rev Mater, 3(8): 257–278. http://doi.org/10.1038/S41578-018-0034-7.
Saleh FA, Genever PG, 2011, Turning round: Multipotent stromal cells, a three-dimensional revolution? Cytotherapy, 13(8): 903–912. http://doi.org/10.3109/14653249.2011.586998.
Joseph JS, Malindisa ST, Ntwasa M, 2018, Two-dimensional (2D) and three-dimensional (3D) cell culturing in drug discovery, in Cell Culture, IntechOpen. http://doi.org/10.5772/INTECHOPEN.81552.
Harb A, Fakhreddine M, Zaraket H, et al., 2021, Three-dimensional cell culture models to study respiratory virus infections including COVID-19. Biomimetics (Basel), 7(1): 3. http://doi.org/10.3390/BIOMIMETICS7010003.
Rijsbergen LC, van Dijk LLA, Engel MFM, et al., 2021, In vitro modelling of respiratory virus infections in human airway epithelial cells – A systematic review. Front Immunol, 12(1): 683002. http://doi.org/10.3389/FIMMU.2021.683002/BIBTEX.
Krüger J, Groß R, Conzelmann C, et al., 2021, Drug inhibition of SARS-CoV-2 replication in human pluripotent stem cell-derived intestinal organoids. Cell Mol Gastroenterol Hepatol, 11(4): 935–948. http://doi.org/10.1016/J.JCMGH.2020.11.003.
Ben-David U, Siranosian B, Ha G, et al., 2018, Genetic and transcriptional evolution alters cancer cell line drug response. Nature, 560(7718): 325–330. http://doi.org/10.1038/S41586-018-0409-3.
Neufeld L, Yeini E, Reisman N, et al., 2021, Microengineered perfusable 3D-bioprinted glioblastoma model for in vivo mimicry of tumor microenvironment. Sci Adv, 7(34): eabi9119. http://doi.org/10.1126/SCIADV.ABI9119.
Butcher DT, Alliston T, Weaver VM, 2009, A tense situation: Forcing tumour progression. Nat Rev Cancer, 9(2): 108–122. http://doi.org/10.1038/NRC2544.
Agarwal K, Srinivasan V, Lather V, et al., 2022, Insights of 3D bioprinting and focusing the paradigm shift towards 4D printing for biomedical applications. J Mater Res, 38(1): 112–141. http://doi.org/10.1557/S43578-022-00524-2.
Tamay DG, Usal TD, Alagoz AS, et al., 2019, 3D and 4D printing of polymers for tissue engineering applications. Front Bioeng Biotechnol, 7(JUL): 164. http://doi.org/10.3389/FBIOE.2019.00164/BIBTEX.
Aprecia, 2015, Spritam, viewed March 02, 2023, https://www.aprecia.com/technology/zipdose