3D-printed gradient scaffolds for osteochondral defects: Current status and perspectives
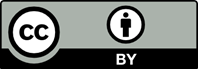
Articular osteochondral defects are quite common in clinical practice, and tissue engineering techniques can offer a promising therapeutic option to address this issue.The articular osteochondral unit comprises hyaline cartilage, calcified cartilage zone (CCZ), and subchondral bone.As the interface layer of articular cartilage and bone, the CCZ plays an essentialpart in stress transmission and microenvironmental regulation.Osteochondral scaffolds with the interface structure for defect repair are the future direction of tissue engineering. Three-dimensional (3D) printing has the advantages of speed, precision, and personalized customization, which can satisfy the requirements of irregular geometry, differentiated composition, and multilayered structure of articular osteochondral scaffolds with boundary layer structure. This paper summarizes the anatomy, physiology, pathology, and restoration mechanisms of the articular osteochondral unit, and reviews the necessity for a boundary layer structure in osteochondral tissue engineering scaffolds and the strategy for constructing the scaffolds using 3D printing. In the future, we should not only strengthen the basic research on osteochondral structural units, but also actively explore the application of 3D printing technology in osteochondral tissue engineering. This will enable better functional and structural bionics of the scaffold, which ultimately improve the repair of osteochondral defects caused by various diseases.
1. Armiento AR, Alini M, Stoddart MJ, 2019, Articular fibrocartilage—Why does hyaline cartilage fail to repair? Adv Drug Deliv Rev, 146:289–305.
2. Yasui Y, Wollstein A, Murawski CD, et al., 2017, Operative treatment for osteochondral lesions of the talus: Biologics and scaffold-based therapy. Cartilage, 8(1):42–49.
3. Gobbi A, Lane JG, Dallo I, 2020, Editorial commentary: Cartilage restoration—What is currently available? 36(6): 1625–1628.
4. Assenmacher AT, Pareek A, Reardon PJ, et al., 2016, Long-term outcomes after osteochondral allograft: A systematic review at long-term follow-up of 12.3 years. Arthroscopy, 32(10):2160–2168.
5. Hoshi K, Fujihara Y, Yamawaki T, et al., 2018, Biological aspects of tissue-engineered cartilage. Histochem Cell Biol, 149(4):375–381.
6. Kok A, van Bergen C, Tuijthof G, et al., 2015, Macroscopic ICRS poorly correlates with O Driscoll histological cartilage repair assessment in a goat model. Clin Res Foot Ankle:1–7.
7. Galperin A, Floreani RA, Florczyk SJ, et al., 2013, Integrated bi‐layered scaffold for osteochondral tissue engineering. Adv Healthc Mater, 2(6):872–883.
8. Camarero-Espinosa S, Cooper-White J, 2017, Tailoring biomaterial scaffolds for osteochondral repair. Int J Pharm, 523(2):476–489.
9. Duraine G, Hu J, Athanasiou K, 2011, Bioengineering in the oral cavity: Insights from articular cartilage tissue engineering. Int J Oral Maxillofac Implant, 26(Suppl):11.
10. Grogan SP, Miyaki S, Asahara H, et al., 2009, Mesenchymal progenitor cell markers in human articular cartilage: Normal distribution and changes in osteoarthritis. Arthritis Res Ther, 11(3):1–13.
11. Boushell MK, Hung CT, Hunziker EB, et al., 2017, Current strategies for integrative cartilage repair. Connect Tissue Res, 58(5):393–406.
12. Kuyinu EL, Narayanan G, Nair LS, et al., 2016, Animal models of osteoarthritis: Classification, update, and measurement of outcomes. J Orthop Surg Res, 11(1):1–27.
13. Fujioka R, Aoyama T, Takakuwa T, 2013, The layered structure of the articular surface. Osteoarthritis Cartilage, 21(8):1092–1098.
14. Hunziker EB, Michel M, Studer D, 1997, Ultrastructure of adult human articular cartilage matrix after cryotechnical processing. Microsc Res Tech, 37(4):271–284.
15. Hoemann CD, Lafantaisie-Favreau C-H, Lascau-Coman V, et al., 2012, The cartilage-bone interface. J Knee Surg, 25(02):085–098.
16. Mente P, Lewis J, 1994, Elastic modulus of calcified cartilage is an order of magnitude less than that of subchondral bone. J Orthop Res, 12(5):637–647.
17. Schinagl RM, Gurskis D, Chen AC, et al., 1997, Depth-dependent confined compression modulus of full‐thickness bovine articular cartilage. J Orthop Res, 15(4): 499–506.
18. Blumer MJ, Longato S, Fritsch H, 2008, Structure, formation and role of cartilage canals in the developing bone. Ann Anat Anatom Anzeiger, 190(4):305–315.
19. Youn I, Choi J, Cao L, et al., 2006, Zonal variations in the three-dimensional morphology of the chondron measured in situ using confocal microscopy. Osteoarthritis Cartilage, 14(9):889–897.
20. Hunziker EB, 2002, Articular cartilage repair: Basic science and clinical progress. A review of the current status and prospects. Osteoarthritis Cartilage, 10(6):432–463.
21. Buckwalter J, Mankin H, 1998, Articular cartilage: Degeneration and osteoarthritis, repair, regeneration, and transplantation. Instr Course Lect, 47:487–504.
22. Mort JS, Billington CJ, 2001, Articular cartilage and changes in arthritis: Matrix degradation. Arthritis Res Ther, 3(6):1–5.
23. Mcculloch K, Litherland GJ, Rai TS, 2017, Cellular senescence in osteoarthritis pathology. Aging Cell, 16(2):210–218.
24. Jeon OH, David N, Campisi J, et al., 2018, Senescent cells and osteoarthritis: A painful connection. J Clin Investig, 128(4):1229–1237.
25. Di Micco R, Krizhanovsky V, Baker D, et al., 2021, Cellular senescence in ageing: From mechanisms to therapeutic opportunities. Nat Rev Mol Cell Biol, 22(2):75–95.
26. Martin JA, Buckwalter JA, 2002, Human chondrocyte senescence and osteoarthritis. Biorheology, 39(1-2): 145–152.
27. Price JS, Waters JG, Darrah C, et al., 2002, The role of chondrocyte senescence in osteoarthritis. Aging Cell, 1(1):57–65.
28. Loeser RF, 2009, Aging and osteoarthritis: The role of chondrocyte senescence and aging changes in the cartilage matrix. Osteoarthritis Cartilage, 17(8):971–979.
29. Gao SG, Zeng C, Li LJ, et al., 2016, Correlation between senescence‐associated beta‐galactosidase expression in articular cartilage and disease severity of patients with knee osteoarthritis. Int J Rheum Dis, 19(3):226–232.
30. Jacob J, Aggarwal A, Aggarwal A, et al., 2022, Senescent chondrogenic progenitor cells derived from articular cartilage of knee osteoarthritis patients contributes to senescence-associated secretory phenotype via release of IL-6 and IL-8. Acta Histochem, 124(3):151867.
31. Childs BG, Gluscevic M, Baker DJ, et al., 2017, Senescent cells: An emerging target for diseases of ageing. Nat Rev Drug Discov, 16(10):718–735.
32. Demaria M, Ohtani N, Youssef SA, et al., 2014, An essential role for senescent cells in optimal wound healing through secretion of PDGF-AA. Dev Cell, 31(6):722–733.
33. Yao Z, Chen P, Wang S, et al., 2019, Reduced PDGF‐AA in subchondral bone leads to articular cartilage degeneration after strenuous running. J Cell Physiol, 234(10):17946– 17958.
34. Udalova IA, Mantovani A, Feldmann M, 2016, Macrophage heterogeneity in the context of rheumatoid arthritis. Nat Rev Rheumatol, 12(8):472–485.
35. Boutet M-A, Courties G, Nerviani A, et al., 2021, Novel insights into macrophage diversity in rheumatoid arthritis synovium. Autoimmun Rev, 20(3):102758.
36. Moelants EA, Mortier A, van Damme J, et al., 2013, Regulation of TNF‐α with a focus on rheumatoid arthritis. Immunol Cell Biol, 91(6):393–401.
37. Romas E, Gillespie M, Martin T, 2002, Involvement of receptor activator of NFκB ligand and tumor necrosis factor-α in bone destruction in rheumatoid arthritis. Bone, 30(2):340–346.
38. Yang P, Qian FY, Zhang MF, et al., 2019, Th17 cell pathogenicity and plasticity in rheumatoid arthritis. J Leukoc Biol, 106(6):1233–1240.
39. Niu Q, Cai B, Huang Z-C, et al., 2012, Disturbed Th17/Treg balance in patients with rheumatoid arthritis. Rheumatol Int, 32(9):2731–2736.
40. Peal BT, Gagliardi R, Su J, et al., 2020, Synovial fluid lubricin and hyaluronan are altered in equine osteochondral fragmentation, cartilage impact injury, and full‐thickness cartilage defect models. J Orthop Res, 38(8):1826–1835.
41. Costouros JG, Dang AC, Kim HT, 2004, Comparison of chondrocyte apoptosis in vivo and in vitro following acute osteochondral injury. J Orthop Res, 22(3):678–683.
42. Bornes TD, Adesida AB, Jomha NM, 2014, Mesenchymal stem cells in the treatment of traumatic articular cartilage defects: A comprehensive review. Arthritis Res Ther, 16(5):1–19.
43. Carballo CB, Nakagawa Y, Sekiya I, et al., 2017, Basic science of articular cartilage. Clin Sports Med, 36(3):413–425.
44. Steadman JR, Briggs KK, Rodrigo JJ, et al., 2003, Outcomes of microfracture for traumatic chondral defects of the knee: Average 11-year follow-up. Arthroscopy, 19(5): 477–484.
45. Bajpayee AG, Grodzinsky AJ, 2017, Cartilage-targeting drug delivery: Can electrostatic interactions help? Nat Rev Rheumatol, 13(3):183–193.
46. Niu X, Li N, Du Z, et al., 2023, Integrated gradient tissue-engineered osteochondral scaffolds: Challenges, current efforts and future perspectives. Bioact Mater, 20:574–597.
47. O’Connell C, Ren J, Pope L, et al., 2020, Characterizing bioinks for extrusion bioprinting: Printability and rheology, in 3D Bioprinting, 111–133.
48. O’Connell CD, Konate S, Onofrillo C, et al., 2020, Free-form co-axial bioprinting of a gelatin methacryloyl bio-ink by direct in situ photo-crosslinking during extrusion. Bioprinting, 19:e00087.
49. Sodupe Ortega E, Sanz-Garcia A, Pernia-Espinoza A, et al., 2019, Efficient fabrication of polycaprolactone scaffolds for printing hybrid tissue-engineered constructs. Materials, 12(4):613.
50. Gong L, Li J, Zhang J, et al., 2020, An interleukin-4-loaded bi-layer 3D printed scaffold promotes osteochondral regeneration. Acta Biomater, 117:246–260.
51. Ginestra P, Pandini S, Ceretti E, 2020, Hybrid multi-layered scaffolds produced via grain extrusion and electrospinning for 3D cell culture tests. Rapid Prototyp J, 26(3):593–602.
52. Kilian D, Ahlfeld T, Akkineni AR, et al., 2020, 3D bioprinting of osteochondral tissue substitutes—In vitro-chondrogenesis in multi-layered mineralized constructs. Sci Rep, 10(1):1–17.
53. Diloksumpan P, De Ruijter M, Castilho M, et al., 2020, Combining multi-scale 3D printing technologies to engineer reinforced hydrogel-ceramic interfaces. Biofabrication, 12(2):025014.
54. Chen L, Deng C, Li J, et al., 2019, 3D printing of a lithium-calcium-silicate crystal bioscaffold with dual bioactivities for osteochondral interface reconstruction. Biomaterials, 196:138–150.
55. Gao F, Xu Z, Liang Q, et al., 2018, Direct 3D printing of high strength biohybrid gradient hydrogel scaffolds for efficient repair of osteochondral defect. Adv Funct Mater, 28(13):1706644.
56. Saidy NT, Wolf F, Bas O, et al., 2019, Biologically inspired scaffolds for heart valve tissue engineering via melt electrowriting. Small, 15(24):1900873.
57. Hejazi F, Bagheri-Khoulenjani S, Olov N, et al., 2021, Fabrication of nanocomposite/nanofibrous functionally graded biomimetic scaffolds for osteochondral tissue regeneration. J Biomed Mater Res A, 109(9):1657–1669.
58. Wortmann M, Frese N, Sabantina L, et al., 2019, New polymers for needleless electrospinning from low-toxic solvents. Nanomaterials, 9(1):52.
59. Saidy NT, Shabab T, Bas O, et al., 2020, Melt electrowriting of complex 3D anatomically relevant scaffolds. Front Bioeng Biotechnol, 8:793.
60. Wunner FM, Wille ML, Noonan TG, et al., 2018, Melt electrospinning writing of highly ordered large volume scaffold architectures. Adv Mater, 30(20):1706570.
61. Stansbury JW, Idacavage MJ, 2016, 3D printing with polymers: Challenges among expanding options and opportunities. Dent Mater, 32(1):54–64.
62. Derakhshanfar S, Mbeleck R, Xu K, et al., 2018, 3D bioprinting for biomedical devices and tissue engineering: A review of recent trends and advances. Bioact Mater, 3(2):144–156.
63. Kumar H, Sakthivel K, Mohamed MG, et al., 2021, Designing gelatin methacryloyl (GelMA)‐based bioinks for visible light stereolithographic 3D biofabrication. Macromol Biosci, 21(1):2000317.
64. Zhu S, Chen P, Chen Y, et al., 2020, 3D-printed extracellular matrix/polyethylene glycol diacrylate hydrogel incorporating the anti-inflammatory phytomolecule honokiol for regeneration of osteochondral defects. Am J Sports Med, 48(11):2808–2818.
65. Mano J, Reis R, 2007, Osteochondral defects: Present situation and tissue engineering approaches. J Tissue Eng Regen Med, 1(4):261–273.
66. Galois L, Freyria A, Grossin L, et al., 2004, Cartilage repair: Surgical techniques and tissue engineering using polysaccharide- and collagen-based biomaterials. Biorheology, 41(3-4):433–443.
67. Nair LS, Laurencin CT, 2007, Biodegradable polymers as biomaterials. Progr Polym Sci, 32(8-9):762–798.
68. Gonçalves AM, Moreira A, Weber A, et al., 2021, Osteochondral tissue engineering: The potential of electrospinning and additive manufacturing. Pharmaceutics, 13(7):983.
69. Qiao Z, Lian M, Han Y, et al., 2021, Bioinspired stratified electrowritten fiber-reinforced hydrogel constructs with layer-specific induction capacity for functional osteochondral regeneration. Biomaterials, 266:120385.
70. Li L, Duan X, Fan Z, et al., 2018, Mesenchymal stem cells in combination with hyaluronic acid for articular cartilage defects. Sci Rep, 8(1):1–11.
71. Huang X, Chen Z, Zhao G, et al., 2020, Combined culture experiment of mouse bone marrow mesenchymal stem cells and bioceramic scaffolds. Exp Ther Med, 20(5):19.
72. Zhang L, He A, Yin Z, et al., 2014, Regeneration of human-ear-shaped cartilage by co-culturing human microtia chondrocytes with BMSCs. Biomaterials, 35(18): 4878–4887.
73. Peng XB, Zhang Y, Wang YQ, et al., 2019, IGF‐1 and BMP‐7 synergistically stimulate articular cartilage repairing in the rabbit knees by improving chondrogenic differentiation of bone-marrow mesenchymal stem cells. J Cell Biochem, 120(4):5570–5582.
74. Xue J, He A, Zhu Y, et al., 2018, Repair of articular cartilage defects with acellular cartilage sheets in a swine model. Biomed Mater, 13(2):025016.
75. Koh Y-G, Kwon O-R, Kim Y-S, et al., 2016, Adipose-derived mesenchymal stem cells with microfracture versus microfracture alone: 2-year follow-up of a prospective randomized trial. Arthroscopy, 32(1):97–109.
76. Williams R, Khan IM, Richardson K, et al., 2010, Identification and clonal characterisation of a progenitor cellsub-population in normal human articular cartilage. PLoS One, 5(10):e13246.
77. Fellows CR, Williams R, Davies IR, et al., 2017, Characterisation of a divergent progenitor cell sub-populations in human osteoarthritic cartilage: The role of telomere erosion and replicative senescence. Sci Rep, 7(1):1–11.
78. Mccarthy HE, Bara JJ, Brakspear K, et al., 2012, The comparison of equine articular cartilage progenitor cells and bone marrow-derived stromal cells as potential cell sources for cartilage repair in the horse. Vet J, 192(3):345–351.
79. Wakitani S, Aoki H, Harada Y, et al., 2004, Embryonic stem cells form articular cartilage, not teratomas, in osteochondral defects of rat joints. Cell Transplant, 13(4):331–336.
80. Mckee C, Hong Y, Yao D, et al., 2017, Compression induced chondrogenic differentiation of embryonic stem cells in three-dimensional polydimethylsiloxane scaffolds. Tissue Eng Part A, 23(9-10):426–435.
81. Chan CK, Seo EY, Chen JY, et al., 2015, Identification and specification of the mouse skeletal stem cell. Cell, 160(1-2): 285–298.
82. Murphy MP, Koepke LS, Lopez MT, et al., 2020, Articular cartilage regeneration by activated skeletal stem cells. Nat Med, 26(10):1583–1592.
83. Khader A, Arinzeh TL, 2020, Biodegradable zinc oxide composite scaffolds promote osteochondral differentiation of mesenchymal stem cells. Biotechnol Bioeng, 117(1):194–209.
84. Deng C, Lin R, Zhang M, et al., 2019, Micro/nanometer‐structured scaffolds for regeneration of both cartilage and subchondral bone. Adv Funct Mater, 29(4):1806068.
85. Chen G, Sato T, Tanaka J, et al., 2006, Preparation of a biphasic scaffold for osteochondral tissue engineering. Mater Sci Eng C, 26(1):118–123.
86. Seo S-J, Mahapatra C, Singh RK, et al., 2014, Strategies for osteochondral repair: Focus on scaffolds. J Tissue Eng, 5:2041731414541850.
87. Deng C, Yang J, He H, et al., 2021, 3D bio-printed biphasic scaffolds with dual modification of silk fibroin for the integrated repair of osteochondral defects. Biomater Sci, 9(14):4891–4903.
88. Simkin PA, 2012, Consider the tidemark. J Rheumatol: 890–892.
89. Goldring SR, Goldring MB, 2016, Changes in the osteochondral unit during osteoarthritis: Structure, function and cartilage–bone crosstalk. Nat Rev Rheumatol, 12(11):632–644.
90. Levingstone TJ, Matsiko A, Dickson GR, et al., 2014, A biomimetic multi-layered collagen-based scaffold for osteochondral repair. Acta Biomater, 10(5):1996–2004.
91. Zhu M, He X, Xin C, et al., 2020, 3D printing of an integrated triphasic MBG-alginate scaffold with enhanced interface bonding for hard tissue applications. J Mater Sci Mater Med, 31(12):1–8.
92. Huey DJ, Hu JC, Athanasiou KA, 2012, Unlike bone, cartilage regeneration remains elusive. Science, 338(6109):917–921.
93. Mancini IA, Vindas Bolaños RA, Brommer H, et al., 2017, Fixation of hydrogel constructs for cartilage repair in the equine model: A challenging issue. Tissue Eng C Methods, 23(11):804–814.
94. Mancini I, Schmidt S, Brommer H, et al., 2020, A composite hydrogel-3D printed thermoplast osteochondral anchor as example for a zonal approach to cartilage repair: In vivo performance in a long-term equine model. Biofabrication, 12(3):035028.
95. Zhang B, Huang J, Narayan RJ, 2020, Gradient scaffolds for osteochondral tissue engineering and regeneration. J Mater Chem B, 8(36):8149–8170.
96. Nooeaid P, Salih V, Beier JP, et al., 2012, Osteochondral tissue engineering: Scaffolds, stem cells and applications. J Cell Mol Med, 16(10):2247–2270.
97. Nooeaid P, Roether JA, Weber E, et al., 2014, Technologies for multilayered scaffolds suitable for interface tissue engineering. Adv Eng Mater, 16(3):319–327.
98. Di Luca A, Lorenzo‐Moldero I, Mota C, et al., 2016, Tuning cell differentiation into a 3D scaffold presenting a pore shape gradient for osteochondral regeneration. Adv Healthc Mater, 5(14):1753–1763.
99. Lowen JM, Leach JK, 2020, Functionally graded biomaterials for use as model systems and replacement tissues. Adv Funct Mater, 30(44):1909089.
100. Radhakrishnan J, Manigandan A, Chinnaswamy P, et al., 2018, Gradient nano-engineered in situ forming composite hydrogel for osteochondral regeneration. Biomaterials, 162:82–98.
101. Liu Z, Meyers MA, Zhang Z, et al., 2017, Functional gradients and heterogeneities in biological materials: Design principles, functions, and bioinspired applications. Progr Mater Sci, 88:467–498.
102. Guo J, Li C, Ling S, et al., 2017, Multiscale design and synthesis of biomimetic gradient protein/biosilica composites for interfacial tissue engineering. Biomaterials, 145:44–55.
103. Singh YP, Moses JC, Bandyopadhyay A, et al., 2022, 3D bioprinted silk‐based in vitro osteochondral model for osteoarthritis therapeutics. Adv Healthc Mater:2200209.