Co-culture bioprinting of tissue-engineered bone-periosteum biphasic complex for repairing critical-sized skull defects in rabbits
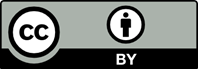
Tissue engineering based on bioprinting technology has broad prospects in the treatment of critical-sized bone defect. Nevertheless, it is challenging to construct composite tissues or organs with structural integrity. Periosteum and stem cells are important in bone regeneration, and it has been shown that co-culture engineering system could successfully repair bone defects. Here, a strategy of co-culture bioprinting was proposed, and a tissue-engineered bone-periosteum biphasic complex was designed. Poly-L-lactic acid/hydroxyapatite (PLLA/HA) was used to construct the supporting scaffold of bone phase. Gelatin methacryl (GelMA) loaded with rabbit bone mesenchymal stem cells (BMSCs) and periosteum-derived stem cells (PDSCs) were used to simulate the extracellular matrix and cellular components of bone and periosteum, respectively, and a co-culture layer was formed between the bone and the periosteum phase. By adjusting material ratios of PLLA/HA and crosslinking time of GelMA, a complex with good mechanical strength and cell activity was constructed and then implanted into the defect area of rabbit skull. The quantitative results of imaging and histology showed that the repair effect of bone-periosteum biphasic complex group was significantly better than that of other control groups, which demonstrated that the bone-periosteum biphasic complex was advantageous to both bone repair and regeneration. In general, using the co-culture bioprinting to construct engineered tissue is a very promising strategy, which is expected to be applied in the construction of more complex tissues and solid organs for tissue repair and organ transplantation.
1. Roddy E, DeBaun MR, Daoud-Gray A, et al., 2018, Treatment of critical-sized bone defects: Clinical and tissue engineering perspectives. Eur J Orthop Surg Traumatol, 28(3):351–362. https://doi.org/10.1007/s00590-017-2063-0
2. Zhao D, Wang Y, Han D, 2016, Periosteal distraction osteogenesis: An effective method for bone regeneration. Biomed Res Int, 2016:2075317. https://doi.org/10.1155/2016/2075317
3. Bakhshandeh B, Zarrintaj P, Oftadeh M O, et al., 2017, Tissue engineering; strategies, tissues, and biomaterials. Biotechnol Genet Eng Rev, 33(2):144-172. https://doi.org/10.1080/02648725.2018.1430464.
4. Mercado-Pagan AE, Stahl AM, Shanjani Y, et al., 2015, Vascularization in bone tissue engineering constructs. Ann Biomed Eng, 43(3):718–729. https://doi.org/10.1007/s10439-015-1253-3
5. Moradi A, Pakizeh M, Ghassemi T, 2021, A review on bovine hydroxyapatite; extraction and characterization. Biomed Phys Eng Express, 8(1):2057-1976. https://doi.org/10.1088/2057-1976/ac414e
6. Alizadeh-Osgouei M, Li Y, Wen C, 2019, A comprehensive review of biodegradable synthetic polymer-ceramic composites and their manufacture for biomedical applications. Bioact Mater, 4(1):22–36. https://doi.org/10.1016/j.bioactmat.2018.11.003
7. Fitzgerald R, Bass LM, Goldberg DJ, et al., 2018, Physiochemical characteristics of poly-L-lactic acid (PLLA). Aesthet Surg J, 38(suppl_1):S13–S17. https://doi.org/10.1093/asj/sjy012
8. Blanpain C, Fuchs E, 2014, Stem cell plasticity. Plasticity of epithelial stem cells in tissue regeneration. Science, 344(6189):1242281. https://doi.org/10.1126/science.1242281
9. Lin H, Sohn J, Shen H, et al., 2019, Bone marrow mesenchymal stem cells: Aging and tissue engineering applications to enhance bone healing. Biomaterials, 203:96–110. https://doi.org/10.1016/j.biomaterials.2018.06.026
10. Baldwin JG, Wagner F, Martine LC, et al., 2017, Periosteum tissue engineering in an orthotopic in vivo platform. Biomaterials, 121:193–204. https://doi.org/10.1016/j.biomaterials.2016.11.016
11. Zhang X, Awad HA, O’Keefe RJ, et al., 2008, A perspective: Engineering periosteum for structural bone graft healing. Clin Orthop Relat Res, 466(8):1777–1787. https://doi.org/10.1007/s11999-008-0312-6
12. Ferretti C, Mattioli-Belmonte M, 2014, Periosteum derived stem cells for regenerative medicine proposals: Boosting current knowledge. World J Stem Cells, 6(3):266–277. https://doi.org/10.4252/wjsc.v6.i3.266
13. Li N, Song J, Zhu G, et al., 2016, Periosteum tissue engineering-a review. Biomater Sci, 4(11):1554–1561. https://doi.org/10.1039/c6bm00481d
14. Lou Y, Wang H, Ye G, et al., 2021, Periosteal tissue engineering: Current developments and perspectives. Adv Healthc Mater, 10(12):e2100215. https://doi.org/10.1002/adhm.202100215
15. Chen D, Zhang X, He Y, et al., 2012, Co-culturing mesenchymal stem cells from bone marrow and periosteum enhances osteogenesis and neovascularization of tissue-engineered bone. J Tissue Eng Regen Med, 6(10):822–832. https://doi.org/10.1002/term.489
16. Liu Y, Chan JK, Teoh SH, 2015, Review of vascularised bone tissue-engineering strategies with a focus on co-culture systems. J Tissue Eng Regen Med, 9(2):85–105. https://doi.org/10.1002/term.1617
17. Borciani G, Montalbano G, Baldini N, et al., 2020, Co-culture systems of osteoblasts and osteoclasts: Simulating in vitro bone remodeling in regenerative approaches. Acta Biomater, 108:22–45. https://doi.org/10.1016/j.actbio.2020.03.043
18. Mandrycky C, Wang Z, Kim K, et al., 2016, 3D bioprinting for engineering complex tissues. Biotechnol Adv, 34(4): 422–434. https://doi.org/10.1016/j.biotechadv.2015.12.011
19. Murphy SV, Atala A, 2014, 3D bioprinting of tissues and organs. Nat Biotechnol, 32(8):773–785. https://doi.org/10.1038/nbt.2958
20. Yue K, Trujillo-de Santiago G, Alvarez MM, et al., 2015, Synthesis, properties, and biomedical applications of gelatin methacryloyl (GelMA) hydrogels. Biomaterials, 73: 254–271. https://doi.org/10.1016/j.biomaterials.2015.08.045
21. Sun M, Sun X, Wang Z, et al., 2018, Synthesis and properties of gelatin methacryloyl (GelMA) gydrogels and their recent applications in load-bearing tissue. Polymers (Basel), 10(11):1290. https://doi.org/10.3390/polym10111290
22. Zhao D, Jiang W, Wang Y, et al., 2020, Three-dimensional-printed poly-L-lactic acid scaffolds with different pore sizes influence periosteal distraction osteogenesis of a rabbit skull. Biomed Res Int, 2020:7381391. https://doi.org/10.1155/2020/7381391
23. Debnath S, Yallowitz AR, McCormick J, et al., 2018, Discovery of a periosteal stem cell mediating intramembranous bone formation. Nature, 562(7725):133–139. https://doi.org/10.1038/s41586-018-0554-8
24. Yin J, Yan M, Wang Y, et al., 2018, 3D bioprinting of low-concentration cell-laden gelatin methacrylate (GelMA) bioinks with a two-step cross-linking strategy. ACS Appl Mater Interfaces, 10(8):6849–6857. https://doi.org/10.1021/acsami.7b16059
25. Valtanen RS, Yang YP, Gurtner GC, et al., 2021, Synthetic and bone tissue engineering graft substitutes: What is the future? Injury, 52(Suppl 2):S72–S77. https://doi.org/10.1016/j.injury.2020.07.040
26. Zhang W, Wang N, Yang M, et al., 2022, Periosteum and development of the tissue-engineered periosteum for guided bone regeneration. J Orthop Translat, 33:41–54. https://doi.org/10.1016/j.jot.2022.01.002
27. Hadjidakis DJ, Androulakis, II, 2006, Bone remodeling. Ann N Y Acad Sci, 1092:385–396. https://doi.org/10.1196/annals.1365.035
28. Marx RE, 2007, Bone and bone graft healing. Oral Maxillofac Surg Clin North Am, 19(4):455–466, v. https://doi.org/10.1016/j.coms.2007.07.008
29. Colnot C, 2009, Skeletal cell fate decisions within periosteum and bone marrow during bone regeneration. J Bone Miner Res, 24(2):274–282. https://doi.org/10.1359/jbmr.081003
30. Chehelcheraghi F, Chien S, Bayat M, 2019, Mesenchymal stem cells improve survival in ischemic diabetic random skin flap via increased angiogenesis and VEGF expression. J Cell Biochem, 120(10):17491–17499. https://doi.org/10.1002/jcb.29013
31. Kanno T, Takahashi T, Ariyoshi W, et al., 2005, Tensile mechanical strain up-regulates Runx2 and osteogenic factor expression in human periosteal cells: Implications for distraction osteogenesis. J Oral Maxillofac Surg, 63(4):499–504. https://doi.org/10.1016/j.joms.2004.07.023
32. Veeriah V, Paone R, Chatterjee S, et al., 2019, Osteoblasts regulate angiogenesis in response to mechanical unloading. Calcif Tissue Int, 104(3):344–354. https://doi.org/10.1007/s00223-018-0496-z
33. Tabbaa SM, Horton CO, Jeray KJ, et al., 2014, Role of vascularity for successful bone formation and repair. Crit Rev Biomed Eng, 42(3-4):319–348. https://doi.org/10.1615/critrevbiomedeng.2014011662
34. Dixon DT, Gomillion CT, 2021, Conductive scaffolds for bone tissue engineering: Current state and future outlook. J Funct Biomater, 13(1):1. https://doi.org/10.3390/jfb13010001
35. Elhattab K, Bhaduri SB, Sikder P, 2022, Influence of fused deposition modelling nozzle temperature on the rheology and mechanical properties of 3D printed beta-tricalcium phosphate (TCP)/polylactic acid (PLA) composite. Polymers (Basel), 14(6):1222. https://doi.org/10.3390/polym14061222
36. Ngo HX, Bai Y, Sha J, et al., 2021, A narrative review of u-HA/PLLA, a bioactive resorbable reconstruction material: Applications in oral and maxillofacial surgery. Materials (Basel), 15(1):150. https://doi.org/10.3390/ma15010150
37. Hanafusa S, Matsusue Y, Yasunaga T, et al., 1995, Biodegradable plate fixation of rabbit femoral shaft osteotomies. A comparative study. Clin Orthop Relat Res, 315(6):262–271.
38. Bigham-Sadegh A, Oryan A, 2015, Selection of animal models for pre-clinical strategies in evaluating the fracture healing, bone graft substitutes and bone tissue regeneration and engineering. Connect Tissue Res, 56(3):175–194. https://doi.org/10.3109/03008207.2015.1027341
39. Xiao S, Zhao T, Wang J, et al., 2019, Gelatin methacrylate (GelMA)-based hydrogels for cell transplantation: An effective strategy for tissue engineering. Stem Cell Rev Rep, 15(5):664–679. https://doi.org/10.1007/s12015-019-09893-4
40. Van Den Bulcke AI, Bogdanov B, De Rooze N, et al., 2000, Structural and rheological properties of methacrylamide modified gelatin hydrogels. Biomacromolecules, 1(1):31–38.