Programmable and printable formaldehyde dehydrogenase as an excellent catalyst for biodegradation of formaldehyde
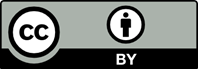
As an environmental pollutant, formaldehyde can cause serious harm to the human body. Among many degradation methods, formaldehyde dehydrogenase from Pseudomonas putida (PFDH) exhibits broad potential because of its strong catalytic specificity and high degradation efficiency. However, the real application of PFDH in industry is limited by its instability and difficulties in recycling. In this work, the suitable printing conditions for immobilizing PFDH by three-dimensional (3D) printing technology were studied: the concentration of sodium alginate (SA) was 1.635 wt%, the concentration of CaCl2 was 7.4 wt%, the crosslinking time with CaCl2 was 8 min, and the temperature of the reaction was 31.5°C. 3D-printed PFDH/calcium alginate (CA) microspheres have 210% relative enzyme activity after seven repeated uses. Dried PFDH/CA particles were characterized by scanning electron microscope (SEM), Fourier transform infrared spectrometer (FT-IR), EDS elemental mapping, and thermogravimetric analysis (TGA) which proved that the enzyme was immobilized by the material. In addition, the recycling ability of 3D printing to immobilize different objects was explored and different shapes were designed by computer-aided design (CAD). In conclusion, 3D printing technology was applied to immobilize PFDH in this work, which provides a new idea to biodegrade formaldehyde in a green way.
1. Jiang W, Pei R, Zhou SF, 2020, 3D-printed xylanase within biocompatible polymers as excellent catalyst for lignocellulose degradation. Chem Eng J, 400:125920– 125928.
2. Cogliano VJ, Baan R, Ghissassi FE, et al., 2006, Formaldehyde, 2-butoxyethanol and 1-tert-butoxypropan-2-ol. Iarc Monogr Eval Carcinog Risks Hum, 88:1–478.
3. Yuan Z, Yang C, Meng F, 2021, Strategies for improving the sensing performance of semiconductor gas sensors for high-performance formaldehyde detection: A review. Chemosensors, 9(7):179–204.
4. Ws A, Lc B, Lei WA, et al., 2021, The influence of doping amount on the catalytic oxidation of formaldehyde by Mn- CeO2 mixed oxide catalyst: A combination of DFT and microkinetic study. J Hazard Mater, 425:127985.
5. Ma L, Seo CY, Chen X, et al., 2018, Schwank, sodium-promoted Ag/CeO2 nanospheres for catalytic oxidation of formaldehyde. Chem Eng J, 350:419–428.
6. Xu Z, Qin N, Wang J, et al., 2010, Formaldehyde biofiltration as affected by spider plant. Biores Technol, 101(18):6930–6934.
7. Rong S, Zhang P, Wang J, et al., 2016, Ultrathin manganese dioxide nanosheets for formaldehyde removal and regeneration performance. Chem Eng J, 306:1172–1179.
8. Huang H, Hu P, Huang H, et al., 2014, Highly dispersed and active supported Pt nanoparticles for gaseous formaldehyde oxidation: Influence of particle size. Chem Eng J, 252:320–326.
9. Zhu X, Wang D, Hui S, 2021, Research progress of adsorption and photocatalysis of formaldehyde on TiO2/AC. Adsorpt Sci Technol, 2021(7):1–16.
10. Wu X, Zuo S, Qiu M, et al., 2021, Atomically defined Co on two-dimensional TiO2 nanosheet for photocatalytic hydrogen evolution. Chem Eng J, 420(2):1–22.
11. Soni V, Goel V, Singh P, et al., 2021, Abatement of formaldehyde with photocatalytic and catalytic oxidation: A review. Int J Chem React Eng, 19(1):1–29.
12. Robert B, Nallathambi G, 2021, Indoor formaldehyde removal by catalytic oxidation, adsorption and nanofibrous membranes: A review. Environ Chem Lett, 19(3):2551–2579.
13. Chen M, Wang H, Chen X, et al., 2020, High-performance of Cu-TiO2 for photocatalytic oxidation of formaldehyde under visible light and the mechanism study. Chem Eng J, 390:1–43.
14. Sun S, Wu X, Huang Z, et al., 2022, Engineering stable Pt nanoclusters on defective two-dimensional TiO2 nanosheets by introducing SMSI for efficient ambient formaldehyde oxidation. Chem Eng J, 435(3):1–49.
15. Tanaka N, Kusakabe Y, Ito K, et al., 2002, Crystal structure of formaldehyde dehydrogenase from Pseudomonas putida: The structural origin of the tightly bound cofactor in nicotinoprotein dehydrogenases. J Mol Biol, 324(3): 519–533.
16. Teierskyt V, Urbonaviius J, Ratautas D, 2021, A direct electron transfer formaldehyde dehydrogenase biosensor for the determination of formaldehyde in river water. Talanta, 234:122657.
17. Liese A, Hilterhaus L, 2013, Evaluation of immobilized enzymes for industrial applications. Chem Soc Rev, 42(15):6236–6249.
18. Mokhtar NF, Rahman R, Noor N, et al., 2020, The immobilization of lipases on porous support by adsorption and hydrophobic interaction method, Catalysts, 10(7):1–17.
19. Paolo Z, Roberto FL, Enrico S, 2016, Agarose and its derivatives as supports for enzyme immobilization. Molecules, 21(11):1–25.
20. Datta D, Tiwari O, Gupta MK, 2019, Self-assembly of diphenylalanine-peptide uncleic acid conjugates. ACS Omega, 4(6):10715–10728.
21. Liang JF, Li YT, Yang VC, 2000, Biomedical application of immobilized enzymes. J Pharm Sci-US, 89(8):979–990.
22. Masuda Y, Kugimiya SI, Murai K, et al., 2013, Enhancement of activity and stability of the formaldehyde dehydrogenase by immobilizing onto phenyl-functionalized mesoporous silica. Colloid Surface B, 101:26–33.
23. Yoshimoto M, Yamashita T, Kinoshita S, 2011, Thermal stabilization of formaldehyde dehydrogenase by encapsulation in liposomes with nicotinamide adenine dinucleotide. Enzyme Microb Tech, 49(2):209–214.
24. Vianello F, Boscolo-Chio R, Signorini S, et al., 2007, On-line detection of atmospheric formaldehyde by a conductometric biosensor. Biosens Bioelectron, 22(6):920–925.
25. Marzuki N, Bakar A, Salleh A, et al., 2012, Electrochemical biosensor immobilization of formaldehyde dehydrogenase with nafion for determination of formaldehyde from Indian mackerel (rastrelliger kanagurta) fish. Curr Anal Chem, 8(4):534–542.
26. Shahrubudin N, Lee TC, Ramlan R, 2019, An overview on 3D printing technology: Technological, materials, and applications. Proc Manuf, 35(2019):1286–1296.
27. Tofail S, Koumoulos EP, Bandyopadhyay A, et al., 2018, Additive manufacturing: Scientific and technological challenges, market uptake and opportunities. Mater Today, 21:22–37.
28. Xu T, Jin J, Gregory C, et al., 2005, Inkjet printing of viable mammalian cells. Biomaterials, 26(1):93–99.
29. Guillotin B, Souquet A, Catros S, et al., 2010, Laser assisted bioprinting of engineered tissue with high cell density and microscale organization. Biomaterials, 31(28):7250–7256.
30. Demirci U, 2006, Acoustic picoliter droplets for emerging applications in semiconductor industry and biotechnology. J Microelectromech S, 15(4):957–966.
31. Smith CM, Stone AL, Parkhill RL, et al., 2004, Three-dimensional bio-assembly tool for generating viable tissue-engineered constructs. Tissue Eng, 10:1566.
32. Zhao Y, Li Y, Mao S, et al., 2015, The influence of printing parameters on cell survival rate and printability in microextrusion-based 3D cell printing technology. Biofabrication, 7(4):45002.
33. Schafer T, Borchert TW, Nielsen VS, et al., 2007, Industrial enzymes. Adv Biochem Eng Biot, 105:59–131.
34. Lu LA, Rui PA, Yl A, et al., 2022, 3D printing of recombinant Escherichia coli /Au nanocomposites as agitating paddles towards robust catalytic reduction of 4-nitrophenol. J Hazard Mater, 423:1–11.
35. Shao Y, Liao Z, Gao B, et al., 2022, Emerging 3D printing strategies for enzyme immobilization: Materials, methods, and applications. ACS Omega, 7(14):11530–11543.
36. Ito K, Takahashi M, Yoshimoto T, et al., 1994, Cloning and high-level expression of the glutathione-independent formaldehyde dehydrogenase gene from Pseudomonas putida. J Bacteriol, 176(9):2483–2491.
37. Suryawanshi RK, Jana UK, Prajapati BP, et al., 2019, Immobilization of Aspergillus quadrilineatus RSNK- 1 multi-enzymatic system for fruit juice treatment and mannooligosaccharide generation. Food Chem, 289:95–102.
38. Sdd A, Jin HB, Dkp A, et al., 2021, 3D-printed bioactive and biodegradable hydrogel scaffolds of alginate/gelatin/ cellulose nanocrystals for tissue engineering. Int J Biol Macromol, 167:644–658.
39. Hua HH, 2007, Study on determination of formaldehyde in mushroom and aquatic products by acetyl-acetone spectrophotometric method. Food Sci, 28(4):273–276.
40. Mueller JM, Dos Santos RL, Brigido RV, 2011, Alginate production by microorganisms. Polimeros, 21(4):305–310.
41. Lou CW, Lee MC, Chen CK, et al., 2016, Effects of the addition of sodium alginate and the concentration of calcium chloride on the properties of composite nonwoven fabrics. MATEC Web Conf, 67:1–7.
42. Silva V, Khatib W, Fleming PJ, 2006, Nonlinear control system design using variable complexity modelling and multiobjective optimization. Sba Control Autom Soc Brasilra Automat, 17(1):24–31.
43. Guo R, Zheng X, Wang Y, et al., 2021, Optimization of cellulase immobilization with sodium alginate-polyethylene for enhancement of enzymatic hydrolysis of microcrystalline cellulose using response surface methodology. Appl Biochem Biotech, 193(7):2043–2060.
44. Hua S, Ma H, Li X, et al., 2010, pH-sensitive sodium alginate/poly (vinyl alcohol) hydrogel beads prepared by combined Ca2+ crosslinking and freeze-thawing cycles for controlled release of diclofenac sodium. Int J Biol Macromol, 46(5):517–523.
45. Deng W, Tang Y, Mao J, et al., 2021, Cellulose nanofibril as a crosslinker to reinforce the sodium alginate/chitosan hydrogels. Int J Biol Macromol, 189:890–899.
46. Zhao G, 2021, Performance study of grass-derived nano-cellulose and polycaprolactone composites for 3D printing. Appl Sci (Basel), 11(3):1–16.
47. Zhou X, Liu C, 2017, Three-dimensional printing for catalytic applications: Current status and perspectives. Adv Funct Mater, 27:1–13.