Study on drug screening multicellular model for colorectal cancer constructed by three-dimensional bioprinting technology
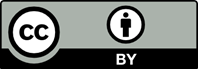
The existing in vitro models for antitumor drug screening have significant limitations. Many compounds that inhibit two-dimensional (2D) cultured cells do not exhibit the same pharmacological effects in vivo, thereby wasting human and material resources and time during drug development. Therefore, it is crucial to develop new models. Three-dimensional (3D) bioprinting technology has greater advantages in constructing human tissues than sandwich culture and organoid construction. We used 3D bioprinting technology to construct a 3D multicellular model of SW480 cells, tumor-associated macrophages, and endothelial cells. The biological activities of the model were evaluated by immunofluorescence, hematoxylin and eosin staining of frozen pathological sections, and transcriptome sequencing. Compared with 3D bioprinted single-cell model (3D printing-S), 3D bioprinted multicellular models (3D printing-M) showed significantly improved expression of tumor-related genes, including hub genes IL1B, FCGR2A, FCGR3A, CYBB, SPI1, CCL2, ITGAM, and ITGB2. Antitumor drug screening experiment showed that the IC50 values of 5-FU, oxaliplatin, and irinotecan in 3D printing-S group/2D culture group were 31.13 µM/12.79 µM, 26.79 µM/0.80 µM, and 16.73 µM/10.45 µM, respectively. Compared with the 3D printing-S group, 3D printing-M group was significantly more resistant to chemotherapy.
1. Biller LH, Schrag D, 2021, Diagnosis and treatment of metastatic colorectal cancer: A review. JAMA, 325: 669–685. https://doi.org/10.1001/jama.2021.0106
2. Giglio MC, Troisi RI, 2021, Laparoscopic surgery for colorectal liver metastases: Moving forward while keeping feet on the ground. Hepatobiliary Surg Nutr, 10: 107–109. https://doi.org/10.21037/hbsn.2020.04.06
3. Schimanski CC, Staib F, Göhler T, et al., 2017, Dermatux: Phase IV trial of Cetuximab plus FOLFIRI in first–line metastatic colorectal cancer receiving a pre–defined skin care. J Cancer Res Clin Oncol, 143: 1023–1034. https://doi.org/10.1007/s00432–017–2344–3
4. Rouwkema J, Khademhosseini A, 2016, Vascularization and angiogenesis in tissue engineering: Beyond creating static networks. Trends Biotechnol, 34: 733–745. https://doi.org/10.1016/j.tibtech.2016.03.002
5. Swift B, Pfeifer ND, Brouwer KL, 2010, Sandwich–cultured hepatocytes: An in vitro model to evaluate hepatobiliary transporter–based drug interactions and hepatotoxicity. Drug Metab Rev, 42: 446–471. https://doi.org/10.3109/03602530903491881
6. Drost J, Clevers H, 2018, Organoids in cancer research. Nat Rev Cancer, 18: 407–418. https://doi.org/10.1038/s41568–018–0007–6
7. Hidalgo M, Amant F, Biankin AV, et al., 2014, Patient– derived xenograft models: An emerging platform for translational cancer research. Cancer Discov, 4: 998–1013. https://doi.org/10.1158/2159–8290.Cd–14–0001
8. Mak IW, Evaniew N, Ghert M, 2014, Lost in translation: Animal models and clinical trials in cancer treatment. Am J Transl Res, 6: 114–118.
9. McGonigle P, Ruggeri B, 2014, Animal models of human disease: Challenges in enabling translation. Biochem Pharmacol, 87: 162–171. https://doi.org/10.1016/j.bcp.2013.08.006
10. Straussman R, Morikawa T, Shee K, et al., 2012, Tumour micro-environment elicits innate resistance to RAF inhibitors through HGF secretion. Nature, 487: 500–504. https://doi.org/10.1038/nature11183
11. Jackstadt R, van Hooff SR, Leach JD, et al., 2019, Epithelial NOTCH signaling rewires the tumor microenvironment of colorectal cancer to drive poor-prognosis subtypes and metastasis. Cancer Cell, 36: 319–336.e317. https://doi.org/10.1016/j.ccell.2019.08.003
12. Xu M, Xu X, Pan B, et al., 2019, LncRNA SATB2-AS1 inhibits tumor metastasis and affects the tumor immune cell microenvironment in colorectal cancer by regulating SATB2. Mol Cancer, 18: 135. https://doi.org/10.1186/s12943-019-1063-6
13. Xiong Y, Wang Y, Tiruthani K, 2019, Tumor immune microenvironment and nano-immunotherapeutics in colorectal cancer. Nanomedicine, 21: 102034. https://doi.org/10.1016/j.nano.2019.102034
14. Khalil S, Sun W, 2009, Bioprinting endothelial cells with alginate for 3D tissue constructs. J Biomech Eng, 131: 111002. https://doi.org/10.1115/1.3128729
15. Matai I, Kaur G, Seyedsalehi A, et al., 2020, Progress in 3D bioprinting technology for tissue/organ regenerative engineering. Biomaterials, 226: 119536. https://doi.org/10.1016/j.biomaterials.2019.119536
16. Lee A, Hudson AR, Shiwarski DJ, et al., 2019, 3D bioprinting of collagen to rebuild components of the human heart. Science, 365: 482–487. https://doi.org/10.1126/science.aav9051
17. Yang H, Sun L, Pang Y, et al., 2021, Three-dimensional bioprinted hepatorganoids prolong survival of mice with liver failure. Gut, 70: 567–574. https://doi.org/10.1136/gutjnl-2019-319960
18. Sun L, Yang H, Wang Y, et al., 2020, Application of a 3D bioprinted hepatocellular carcinoma cell model in antitumor drug research. Front Oncol, 10: 878. https://doi.org/10.3389/fonc.2020.00878
19. Xie F, Sun L, Pang Y, et al., 2021, Three-dimensional bio-printing of primary human hepatocellular carcinoma for personalized medicine. Biomaterials, 265: 120416. https://doi.org/10.1016/j.biomaterials.2020.120416
20. Nie J, Gao Q, Fu J, et al., 2020, Grafting of 3D bioprinting to in vitro drug screening: A review. Adv Healthc Mater, 9: e1901773. https://doi.org/10.1002/adhm.201901773
21. Datta P, Dey M, Ataie Z, et al., 2020, 3D bioprinting for reconstituting the cancer microenvironment. NPJ Precis Oncol, 4: 18. https://doi.org/10.1038/s41698-020-0121-2
22. Zhao Y, Yao R, Ouyang L, et al., 2014, Three-dimensional printing of Hela cells for cervical tumor model in vitro. Biofabrication, 6: 035001. https://doi.org/10.1088/1758–5082/6/3/035001
23. Chouhan D, Dey N, Bhardwaj N, et al., 2019, Emerging and innovative approaches for wound healing and skin regeneration: Current status and advances. Biomaterials, 216: 119267. https://doi.org/10.1016/j.biomaterials.2019.119267
24. Jia W, Gungor-Ozkerim PS, Zhang YS, et al., 2016, Direct 3D bioprinting of perfusable vascular constructs using a blend bioink. Biomaterials, 106: 58–68. https://doi.org/10.1016/j.biomaterials.2016.07.038
25. Ozbolat IT, Hospodiuk M, 2016, Current advances and future perspectives in extrusion-based bioprinting. Biomaterials, 76: 321–343. https://doi.org/10.1016/j.biomaterials.2015.10.076]
26. Paxton N, Smolan W, Böck T, et al., 2017, Proposal to assess printability of bioinks for extrusion-based bioprinting and evaluation of rheological properties governing bioprintability. Biofabrication, 9: 044107. https://doi.org/10.1088/1758-5090/aa8dd8
27. Zhong Y, Herrera-Úbeda C, Garcia-Fernàndez J, et al., 2020, Mutation of amphioxus Pdx and Cdx demonstrates conserved roles for ParaHox genes in gut, anus and tail patterning. BMC Biol, 18: 68. https://doi.org/10.1186/s12915-020-00796-2
28. Menon SS, Guruvayoorappan C, Sakthivel KM, et al., 2019, Ki-67 protein as a tumour proliferation marker. Clin Chim Acta, 491: 39–45. https://doi.org/10.1016/j.cca.2019.01.011
29. Väyrynen JP, Haruki K, Lau MC, et al., 2021, The prognostic role of macrophage polarization in the colorectal cancer microenvironment. Cancer Immunol Res, 9: 8–19. https://doi.org/10.1158/2326-6066.Cir-20-0527
30. Caligiuri G, 2020, CD31 as a therapeutic target in atherosclerosis. Circ Res, 126: 1178–1189. https://doi.org/10.1161/circresaha.120.315935
31. Rastogi P, Kandasubramanian B, 2019, Review of alginate-based hydrogel bioprinting for application in tissue engineering. Biofabrication, 11: 042001.https://doi.org/10.1088/1758-5090/ab331e
32. Cui X, Li J, Hartanto Y, et al., 2020, Advances in extrusion 3D bioprinting: A focus on multicomponent hydrogel-based bioinks. Adv Healthc Mater, 9: e1901648. https://doi.org/10.1002/adhm.201901648
33. Yi B, 2021, An overview of the Chinese healthcare system. Hepatobiliary Surg Nutr, 10: 93–95. https://doi.org/10.21037/hbsn-2021-3
34. Shao L, Gao Q, Xie C, et al., 2020, Directly coaxial 3D bioprinting of large-scale vascularized tissue constructs. Biofabrication, 12: 035014. https://doi.org/10.1088/1758-5090/ab7e76
35. Gao Q, He Y, Fu JZ, et al., 2015, Coaxial nozzle-assisted 3D bioprinting with built-in microchannels for nutrients delivery. Biomaterials, 61: 203–215. https://doi.org/10.1016/j.biomaterials.2015.05.031
36. Levato R, Jungst T, Scheuring RG, et al., 2020, From shape to function: The next step in bioprinting. Adv Mater, 32: e1906423. https://doi.org/10.1002/adma.201906423
37. Kingsley DM, Roberge CL, Rudkouskaya A, et al., 2019, Laser-based 3D bioprinting for spatial and size control of tumor spheroids and embryoid bodies. Acta Biomater, 95: 357–370. https://doi.org/10.1016/j.actbio.2019.02.014
38. You F, Eames BF, Chen X, 2017, Application of extrusion-based hydrogel bioprinting for cartilage tissue engineering. Int J Mol Sci, 18: 1597. https://doi.org/10.3390/ijms18071597
39. Slimane SN, Marcel V, Fenouil T, et al., 2020, Ribosome biogenesis alterations in colorectal cancer. Cells, 9: 2361. https://doi.org/10.3390/cells9112361
40. Lu Y, Li Y, Liu Q, et al., 2021, MondoA-thioredoxin-interacting protein axis maintains regulatory T-cell identity and function in colorectal cancer microenvironment. Gastroenterology, 161: 575–591.e516. https://doi.org/10.1053/j.gastro.2021.04.041
41. Hon KW, Abidin SA, Othman I, et al., 2020, Insights into the role of microRNAs in colorectal cancer (CRC) metabolism. Cancers (Basel), 12: 2462. https://doi.org/10.3390/cancers12092462]
42. Sheng L, Jena PK, Hu Y, et al., 2021, Age-specific microbiota in altering host inflammatory and metabolic signaling as well as metabolome based on the sex. Hepatobiliary Surg Nutr, 10: 31–48. https://doi.org/10.21037/hbsn-20-671
43. Lin X, Zhuang S, Chen X, et al., 2022, lncRNA ITGB8-AS1 functions as a ceRNA to promote colorectal cancer growth and migration through integrin-mediated focal adhesion signaling. Mol Ther, 30: 688–702. https://doi.org/10.1016/j.ymthe.2021.08.011
44. Gordon SR, Maute RL, Dulken BW, et al., 2017, PD-1 expression by tumour-associated macrophages inhibits phagocytosis and tumour immunity. Nature, 545: 495–499. https://doi.org/10.1038/nature22396
45. Li Y, Qi M, Ding F, et al., 2021, Tumour targetable and microenvironment-responsive nanoparticles simultaneously disrupt the PD-1/PD-L1 pathway and MAPK/ERK/JNK pathway for efficient treatment of colorectal cancer. J Drug Target, 29: 454–465. https://doi.org/10.1080/1061186x.2020.1853750
46. Ponsioen B, Post JB, des Amorie JR, et al., 2021, Quantifying single-cell ERK dynamics in colorectal cancer organoids reveals EGFR as an amplifier of oncogenic MAPK pathway signalling. Nat Cell Biol, 23: 377–390. https://doi.org/10.1038/s41556-021-00654-5
47. Rawat M, Nighot M, Al-Sadi R, et al., 2020, IL1B increases intestinal tight junction permeability by up-regulation of MIR200C-3p, which degrades occludin mRNA. Gastroenterology, 159: 1375–1389. https://doi.org/10.1053/j.gastro.2020.06.038
48. Wang X, Zeldin S, Shi H, et al., 2022, TAZ-induced Cybb contributes to liver tumor formation in non-alcoholic steatohepatitis. J Hepatol, 76: 910–920. https://doi.org/10.1016/j.jhep.2021.11.031
49. Herb M, Gluschko A, Schramm M, 2018, LC3-associated phagocytosis initiated by integrin ITGAM-ITGB2/Mac-1 enhances immunity to Listeria monocytogenes. Autophagy, 14: 1462–1464. https://doi.org/10.1080/15548627.2018.1475816
50. Anaya JM, Kim-Howard X, Prahalad S, et al., 2012, Evaluation of genetic association between an ITGAM non-synonymous SNP (rs1143679) and multiple autoimmune diseases. Autoimmun Rev, 11: 276–280. https://doi.org/10.1016/j.autrev.2011.07.007
51. Sugarman R, Patel R, Sharma S, et al., 2019, Pharmacokinetics and pharmacodynamics of new drugs for pancreatic cancer. Expert Opin Drug Metab Toxicol, 15: 541–552. https://doi.org/10.1080/17425255.2019.1637417
52. Yamasaki M, Kimura R, Mayahara S, et al., 2019, Study on the infusion-site adverse events and vascular distribution of epirubicin in chemotherapy with epirubicin and fosaprepitant. Mol Clin Oncol, 11: 43–49. https://doi.org/10.3892/mco.2019.1849
53. Stinchcombe TE, Morris DE, Lee CB, et al., 2008, Induction chemotherapy with carboplatin, irinotecan, conformal thoracic radiotherapy (74 Gy) with concurrent carboplatin, paclitaxel, and gefitinib in unresectable stage IIIA and stage IIIB non-small cell lung cancer. J Thorac Oncol, 3: 250–257. https://doi.org/10.1097/JTO.0b013e3181653cf4
54. Urien S, Lokiec F, 2004, Population pharmacokinetics of total and unbound plasma cisplatin in adult patients. Br J Clin Pharmacol, 57: 756–763. https://doi.org/10.1111/j.1365-2125.2004.02082.x
55. Elias D, Goere D, Blot F, et al., 2007, Optimization of hyperthermic intraperitoneal chemotherapy with oxaliplatin plus irinotecan at 43 degrees C after compete cytoreductive surgery: Mortality and morbidity in 106 consecutive patients. Ann Surg Oncol, 14: 1818–1824. https://doi.org/10.1245/s10434-007-9348-1