Printing channels with millimeter-scale curvature and deciphering their effect on the proliferation, morphology, orientation, and migration of M-22 cells
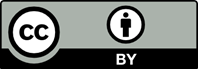
Complex curved structures of tissues have been recognized to influence the behavior and function of cells. Tissue curvatures sensed by cells are approximately on the millimeter scale. However, previous research mainly focused on the effect of micro- and nano-scale spatial curved structures, underestimating the significance of milli-scale curvature. Here, we employed fused deposition modeling (FDM) with two-stage temperature control, superfine cone-shaped needle, stable air pressure, and precise motion platform for the customized production of homogeneous, precise, and curved fibers; the responses of M-22 cells to FDM-printed curved channels with radii of 1.5 to 3 mm were systematically investigated. The cells aligned with these curved channels and exhibited various aspect ratios in the channels with different curvatures. Cell proliferation, migration speed of single cells, and front-end speed of collective cells were tightly regulated by these curved structures. Also, a computational model based on force equilibrium was proposed to explore the essential factors and mechanisms of curvature affecting cell behavior. Our simulation results demonstrated that the curvature and width of channels, along with the relative size of cells, can significantly impact the cell–boundary interaction force and the number of valid pseudopodia generated by cells in the process of cell migration. These results provide a comprehensive understanding of the effect of milli-scale curvature on the cells and underpin the design of scaffolds that can be produced easily with sophisticated micro- and nano-scale curved features to regulate cell behavior in tissue engineering.
1. Huang C-K, Paylaga GJ, Bupphathong S, et al., 2020, Spherical microwell arrays for studying single cells and microtissues in 3D confinement. Biofabrication, 12(2):025016. https://doi.org/10.1088/1758-5090/ab6eda
2. Callens SJP, Uyttendaele RJC, Fratila-Apachitei LE, et al., 2020, Substrate curvature as a cue to guide spatiotemporal cell and tissue organization. Biomaterials, 232:119739. https://doi.org/10.1016/j.biomaterials.2019.119739
3. Altay G, Tosi S, García-Díaz M, et al., 2020, Imaging the cell morphological response to 3D topography and curvature in engineered intestinal tissues. Front Bioeng Biotechnol, 8:294. https://doi.org/10.3389/fbioe.2020.00294
4. An SS, Bai TR, Bates JHT, et al., 2007, Airway smooth muscle dynamics: A common pathway of airway obstruction in asthma. Eur Respir J, 29(5):834–860. https://doi.org/10.1183/09031936.00112606
5. Baptista D, Teixeira L, van Blitterswijk C, et al., 2019, Overlooked? Underestimated? Effects of substrate curvature on cell behavior. Trends Biotechnol, 37(8):838–854. https://doi.org/10.1016/j.tibtech.2019.01.006
6. Vutan A-M, Ciupe V, Gruescu CM, et al., 2018, Experimental method for dynamic evaluation of spinal column deformation exercises. in New Advances in Mechanism and Machine Science, Springer, 137–146. https://doi.org/10.1007/978-3-319-79111-1_13
7. Yang C-Y, Huang W-Y, Chen L-H, et al., 2021, Neural tissue engineering: The influence of scaffold surface topography and extracellular matrix microenvironment. J Mater Chem B, 9(3):567–584. https://doi.org/10.1039/d0tb01605e
8. Lai H, Gong B, Yin J, et al., 2022, 3D printing topographic cues for cell contact guidance: A review. Mater Des, 218:110663. https://doi.org/10.1016/j.matdes.2022.110663
9. Li H, Xu Y, Xu H, et al., 2014, Electrospun membranes: Control of the structure and structure related applications in tissue regeneration and drug delivery. J Mater Chem B, 2(34):5492–5510. https://doi.org/10.1039/c4tb00913d
10. Assoian RK, Bade ND, Cameron CV, et al., 2019, Cellular sensing of micron-scale curvature: A frontier in understanding the microenvironment. Open Biol, 9(10):190155. https://doi.org/10.1098/rsob.190155
11. Wan LQ, Ronaldson K, Park M, et al., 2011, Micropatterned mammalian cells exhibit phenotype-specific left-right asymmetry. Proc Natl Acad Sci, 108(30):12295–12300. https://doi.org/10.1073/pnas.1103834108
12. Mao M, He J, Li Z, et al., 2020, Multi-directional cellular alignment in 3D guided by electrohydrodynamically-printed microlattices. Acta Biomater, 101:141–151. https://doi.org/10.1016/j.actbio.2019.10.028
13. Ray A, Lee O, Win Z, et al., 2017, Anisotropic forces from spatially constrained focal adhesions mediate contact guidance directed cell migration. Nat Commun, 8(1):1–17. https://doi.org/10.1038/ncomms14923
14. Bade ND, Xu T, Kamien RD, et al., 2018, Gaussian curvature directs stress fiber orientation and cell migration. Biophys J, 114(6):1467–1476. https://doi.org/10.1016/j.bpj.2018.01.039
15. Chao PG, Hsu H-Y, Tseng H-Y, 2014, Electrospun microcrimped fibers with nonlinear mechanical properties enhance ligament fibroblast phenotype. Biofabrication, 6(3):035008. https://doi.org/10.1088/1758-5082/6/3/035008
16. Connon CJ, Gouveia RM, 2021, Milliscale substrate curvature promotes myoblast self‐organization and differentiation. Adv Biol, 5(4):2000280. https://doi.org/10.1002/adbi.202000280
17. Son J, Bang MS, Park JK, 2019, Hand-maneuverable collagen sheet with micropatterns for 3D modular tissue engineering. ACS Biomater Sci Eng, 5(1):339–345. http://doi.org/10.1021/acsbiomaterials.8b01066
18. Carthew J, Taylor JBJ, Garcia-Cruz MR, et al., 2021, The bumpy road to stem cell therapies: Rational design of surface topographies to dictate stem cell mechanotransduction and fate. ACS Appl Mater Interfaces, 14(20):23066–23101. https://doi.org/10.1021/acsami.1c22109
19. Mei Y, He C, Gao C, et al., 2021, 3D-printed degradable anti-tumor scaffolds for controllable drug delivery. Int J Bioprinting, 7(4):418. https://doi.org/10.18063/ijb.v7i4.418
20. Saha SK, Wang D, Nguyen VH, et al., 2019, Scalable submicrometer additive manufacturing. Science (80-), 366(6461):105–109. https://doi.org/10.1126/science.aax8760
21. Zhang Z-Z, Wang S-J, Zhang J-Y, et al., 2017, 3D-printed poly (ε-caprolactone) scaffold augmented with mesenchymal stem cells for total meniscal substitution: A 12- and 24-week animal study in a rabbit model. Am J Sports Med, 45(7):1497–1511. https://doi.org/10.1177/0363546517691513
22. Ji S, Guvendiren M, 2019, 3D printed wavy scaffolds enhance mesenchymal stem cell osteogenesis. Micromachines, 11(1):31. https://doi.org/10.3390/mi11010031
23. Hou Y, Xie W, Yu L, et al., 2020, Surface roughness gradients reveal topography‐specific mechanosensitive responses in human mesenchymal stem cells. Small, 16(10):1905422. https://doi.org/10.1002/smll.201905422
24. Nguyen AT, Sathe SR, Yim EKF, 2016, From nano to micro: Topographical scale and its impact on cell adhesion, morphology and contact guidance. J Phys Condens Matter, 28(18):183001. https://doi.org/10.1088/0953-8984/28/18/183001
25. Buyuksungur S, Tanir TE, Buyuksungur A, et al., 2017, 3D printed poly (ε-caprolactone) scaffolds modified with hydroxyapatite and poly (propylene fumarate) and their effects on the healing of rabbit femur defects. Biomater Sci, 5(10):2144–2158. https://doi.org/10.1039/c7bm00514h
26. Hedayati SK, Behravesh AH, Hasannia S, et al., 2020, 3D printed PCL scaffold reinforced with continuous biodegradable fiber yarn: A study on mechanical and cell viability properties. Polym Test, 83106347. https://doi.org/10.1016/j.polymertesting.2020.106347
27. Buttenschön A, Edelstein-Keshet L, 2020, Bridging from single to collective cell migration: A review of models and links to experiments. PLoS Comput Biol, 16(12):e1008411. https://doi.org/10.1371/journal.pcbi.1008411
28. Du W, Chen J, Li H, et al., 2016, Direct cellular organization with ring-shaped composite polymers and glass substrates for urethral sphincter tissue engineering. J Mater Chem B, 4(22):3998–4008. https://doi.org/10.1039/c6tb00437g
29. Zhao J, Manuchehrfar F, Liang J, 2020, Cell–substrate mechanics guide collective cell migration through intercellular adhesion: A dynamic finite element cellular model. Biomech Model Mechanobiol, 19(5):1781–1796. https://doi.org/10.1007/s10237-020-01308-5
30. Albert PJ, Schwarz US, 2014, Dynamics of cell shape and forces on micropatterned substrates predicted by a cellular Potts model. Biophys J, 106(11):2340–2352. https://doi.org/10.1016/j.bpj.2014.04.036
31. McEvoy E, Deshpande VS, McGarry P, 2017, Free energy analysis of cell spreading. J Mech Behav Biomed Mater, 74:283–295. https://doi.org/10.1016/j.jmbbm.2017.06.006
32. Kim M-C, Kim C, Wood L, et al., 2012, Integrating focal adhesion dynamics, cytoskeleton remodeling, and actin motor activity for predicting cell migration on 3D curved surfaces of the extracellular matrix. Integr Biol, 4(11):1386–1397. https://doi.org/10.1039/c2ib20159c
33. Kim M-C, Neal DM, Kamm RD, et al., 2013, Dynamic modeling of cell migration and spreading behaviors on fibronectin coated planar substrates and micropatterned geometries. PLoS Comput Biol, 9(2):e1002926. https://doi.org/10.1371/journal.pcbi.1002926
34. Bangasser BL, Shamsan GA, Chan CE, et al., 2017, Shifting the optimal stiffness for cell migration. Nat Commun, 8(1):1–10. https://doi.org/10.1038/ncomms15313
35. Chang C, Dai Z, Shih P, 2022, Modeling and simulation of cell migration on the basis of force equilibrium. Int J Numer Method Biomed Eng, 38(2):e3550. https://doi.org/10.1002/cnm.3550
36. Zhang J, Gao Z, Zhang Y, et al., 2020, Study on chitosan-based nanocomposite hydrogel in soft tissue defect of hand. Nanosci Nanotechnol Lett, 12(9):1120–1126. https://doi.org/10.1166/nnl.2020.3217
37. Ge L, Yang L, Bron R, et al., 2020, Topography-mediated fibroblast cell migration is influenced by direction, wavelength, and amplitude. ACS Appl Bio Mater, 3(4):2104–2116. https://doi.org/10.1021/acsabm.0c00001
38. Vedula SRK, Leong MC, Lai TL, et al., 2012, Emerging modes of collective cell migration induced by geometrical constraints. Proc Natl Acad Sci, 109(32):12974–12979. https://doi.org/10.1073/pnas.1119313109
39. Seetharaman S, Etienne-Manneville S, 2020, Cytoskeletal crosstalk in cell migration. Trends Cell Biol, 30(9):720–735. https://doi.org/10.1016/j.tcb.2020.06.004
40. Innocenti M, 2018, New insights into the formation and the function of lamellipodia and ruffles in mesenchymal cell migration. Cell Adh Migr, 12(5):401–416. https://doi.org/10.1080/19336918.2018.1448352
41. Moussa HI, Chan WY, Logan M, et al., 2020, Limitation in controlling the morphology of mammalian vero cells induced by cell division on asymmetric tungsten-silicon oxide nanocomposite. Materials (Basel), 13(2):335. https://doi.org/10.3390/ma13020335
42. He S, Liu C, Li X, et al., 2015, Dissecting collective cell behavior in polarization and alignment on micropatterned substrates. Biophys J, 109(3):489–500. https://doi.org/10.1016/j.bpj.2015.06.058
43. Buskermolen ABC, Ristori T, Mostert D, et al., 2020, Cellular contact guidance emerges from gap avoidance. Cell Rep Phys Sci, 1(5):100055. https://doi.org/10.1016/j.xcrp.2020.100055
44. Liu C, Xu J, He S, et al., 2018, Collective cell polarization and alignment on curved surfaces. J Mech Behav Biomed Mater, 88:330–339. https://doi.org/10.1016/j.jmbbm.2018.08.014
45. Iwawaki Y, Mizusawa N, Iwata T, et al., 2015, MiR-494-3p induced by compressive force inhibits cell proliferation in MC3T3-E1 cells. J Biosci Bioeng, 120(4):456–462. https://doi.org/10.1016/j.jbiosc.2015.02.006
46. Pietramaggiori G, Liu P, Scherer SS, et al., 2007, Tensile forces stimulate vascular remodeling and epidermal cell proliferation in living skin. Ann Surg, 246(5):896–902. https://doi.org/10.1097/SLA.0b013e3180caa47f
47. SenGupta S, Parent CA, Bear JE, 2021, The principles of directed cell migration. Nat Rev Mol Cell Biol, 22(8): 529–547. https://doi.org/10.1038/s41580-021-00366-6
48. Yamada KM, Sixt M, 2019, Mechanisms of 3D cell migration. Nat Rev Mol Cell Biol, 20(12):738–752. https://doi.org/10.1038/s41580-019-0172-9
49. D’alessandro J, Cellerin V, Benichou O, et al., 2021, Cell migration guided by long-lived spatial memory. Nat Commun, 12(1):1–10. https://doi.org/10.1038/s41467-021-24249-8
50. Haeger A, Wolf K, Zegers MM, et al., 2015, Collective cell migration: Guidance principles and hierarchies. Trends Cell Biol, 25(9):556–566. https://doi.org/10.1016/j.tcb.2015.06.003
51. Xi W, Sonam S, Beng Saw T, et al., 2017, Emergent patterns of collective cell migration under tubular confinement. Nat Commun, 8(1):1–15. https://doi.org/10.1038/s41467-017-01390-x
52. van Der Putten C, Buskermolen ABC, Werner M, et al., 2021, Protein micropatterning in 2.5 D: An approach to investigate cellular responses in multi-cue environments. ACS Appl Mater Interfaces, 13(22):25589–25598. https://doi.org/10.1021/acsami.1c01984
53. Werner M, Petersen A, Kurniawan NA, et al., 2019, Cell‐perceived substrate curvature dynamically coordinates the direction, speed, and persistence of stromal cell migration. Adv Biosyst, 3(10):1900080. https://doi.org/10.1002/adbi.201900080
54. Werner M, Blanquer SBG, Haimi SP, et al., 2017, Surface curvature differentially regulates stem cell migration and differentiation via altered attachment morphology and nuclear deformation. Adv Sci, 4(2):1600347. https://doi.org/10.1002/advs.201600347
55. Pieuchot L, Marteau J, Guignandon A, et al., 2018, Curvotaxis directs cell migration through cell-scale curvature landscapes. Nat Commun, 9(1):1–13. https://doi.org/10.1038/s41467-018-06494-6
56. Rougerie P, Pieuchot L, Dos Santos RS, et al., 2020, Topographical curvature is sufficient to control epithelium elongation. Sci Rep, 10(1):1–14. https://doi.org/10.1038/s41598-020-70907-0
57. Mathur A, Moore SW, Sheetz MP, et al., 2012, The role of feature curvature in contact guidance. Acta Biomater, 8(7):2595–2601. https://doi.org/10.1016/j.actbio.2012.03.025
58. Bowers DT, Brown JL, 2021, Nanofiber curvature with Rho GTPase activity increases mouse embryonic fibroblast random migration velocity. Integr Biol, 13(12):295–308. https://doi.org/10.1093/intbio/zyab022
59. Werner M, Kurniawan NA, Korus G, et al., 2018, Mesoscale substrate curvature overrules nanoscale contact guidance to direct bone marrow stromal cell migration. J R Soc Interface, 15(145):20180162. https://doi.org/10.1098/rsif.2018.0162