Performance of hybrid gelatin-PVA bioinks integrated with genipin through extrusionbased 3D bioprinting: An in vitro evaluation using human dermal fibroblasts
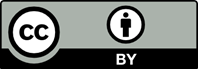
3D bioprinting technology is a well-established and promising advanced fabrication technique that utilizes potential biomaterials as bioinks to replace lost skin and promote new tissue regeneration. Cutaneous regenerative biomaterials are highly commended since they benefit patients with larger wound sizes and irregular wound shapes compared to the painstaking split-skin graft. This study aimed to fabricate biocompatible, biodegradable, and printable bioinks as a cutaneous substitute that leads to newly formed tissue post-transplantation. Briefly, gelatin (GE) and polyvinyl alcohol (PVA) bioinks were prepared in various concentrations (w/v); GE (6% GE: 0% PVA), GPVA3 (6% GE: 3% PVA), and GPVA5 (6% GE: 5% PVA), followed by 0.1% (w/v) genipin (GNP) crosslinking to achieve optimum printability. According to the results, GPVA5_GNP significantly presented at least 590.93 ± 164.7% of swelling ratio capacity and optimal water vapor transmission rate (WVTR), which is <1500 g/m2 /h to maintain the moisture of the wound microenvironment. Besides, GPVA5_GNP is also more durable than other hydrogels with the slowest biodegradation rate of 0.018 ± 0.08 mg/h. The increasing amount of PVA improved the rheological properties of the hydrogels, leading the GPVA5_GNP to have the highest viscosity, around 3.0 ± 0.06 Pa.s. It allows a better performance of bioinks printability via extrusion technique. Moreover, the cross-section of the microstructure hydrogels showed the average pore sizes >100 µm with excellent interconnected porosity. X-ray diffraction (XRD) analysis showed that the hydrogels maintain their amorphous properties and were well-distributed through energy dispersive X-ray after crosslinking. Furthermore, there had no substantial functional group changes, as observed by Fourier transform infrared spectroscopy, after the addition of crosslinker. In addition, GPVA hydrogels were biocompatible to the cells, effectively demonstrating >90% of cell viability. In conclusion, GPVA hydrogels crosslinked with GNP, as prospective bioinks, exhibited the superior properties necessary for wound healing treatment.
1. Wei C, Feng Y, Che D, et al., 2021, Biomaterials in skin tissue engineering. Int J Polym Mater Polym Biomater, 71: 1–19. https://doi.org/10.1080/00914037.2021.1933977
2. Fadilah NI, Jailani MS, Hisham MA, et al., 2022, Cell secretomes for wound healing and tissue regeneration : Next generation acellular based tissue engineered products. J Tissue Eng, 13: 20417314221114273. https://doi.org/10.1177/20417314221114273
3. Weng T, Zhang W, Xia Y, et al., 2021, 3D bioprinting for skin tissue engineering: Current status and perspectives. J Tissue Eng, 12: 20417314211028574. https://doi.org/10.1177/20417314211028574
4. Rodrigues M, Kosaric N, Bonham CA, et al., 2019, Wound healing: A cellular perspective. Physiol Rev, 99: 665–706. https://doi.org/10.1152/physrev.00067.2017
5. Luo R, Dai J, Zhang J, et al., 2021, Accelerated skin wound healing by electrical stimulation. Adv Healthc Mater, 10: 1–15. https://doi.org/10.1002/adhm.202100557
6. Zeng R, Lin C, Lin Z, et al., 2018, Approaches to cutaneous wound healing: Basics and future directions. Cell Tissue Res, 374: 217–232. https://doi.org/10.1007/s00441-018-2830-1
7. Zawani M, Fauzi MB, 2021, Injectable hydrogels for chronic skin wound management: A concise review. Biomedicines, 9: 527. https://doi.org/10.3390/biomedicines9050527
8. Kang JI, Park KM, Kang, 2021, Advances in gelatin-based hydrogels for wound management. J Mater Chem B, 9: 1503– 1520. https://doi.org/10.1039/d0tb02582h
9. Sen CK, 2021, Human wound and its burden: Updated 2020 compendium of estimates. Adv Wound Care (New Rochelle), 10: 281–292. https://doi.org/10.1089/wound.2021.0026
10. Masri S, Zawani M, Zulkiflee I, et al., 2022, Cellular interaction of human skin cells towards natural bioink via 3d-bioprinting technologies for chronic wound: A comprehensive review. Int J Mol Sci, 23: 476. https://doi.org/10.3390/ijms23010476
11. Fuchs C, Pham L, Wang Y, et al., 2021, MagneTEskin-reconstructing skin by magnetically induced assembly of autologous microtissue cores. Sci Adv, 7: 1–11.https://doi.org/10.1126/sciadv.abj0864
12. Boyce ST, Lalley AL, 2018, Tissue engineering of skin and regenerative medicine for wound care. Burns Trauma, 6: 4. https://doi.org/10.1186/s41038-017-0103-y
13. Salleh A, Mustafa N, Teow TH, et al., 2022, Dual-layered approach of ovine collagen-gelatin/cellulose hybrid biomatrix containing graphene oxide-silver nanoparticles for cutaneous wound healing : Fabrication, physicochemical, cytotoxicity and, antibacterial characterisation. Biomedicines, 10: 816.
14. Zulkiflee I, Fauzi MB, 2021, Gelatin-polyvinyl alcohol film for tissue engineering: A concise review. Biomedicines, 9: 979. https://doi.org/10.3390/biomedicines9080979
15. Roy R, Tiwari M, Donelli G, et al., 2018, Strategies for combating bacterial biofilms: A focus on anti-biofilm agents and their mechanisms of action. Virulence, 9: 522–554. https://doi.org/10.1080/21505594.2017.1313372
16. Masri S, Fauzi MB, 2021, Current insight of printability quality improvement strategies in natural-based bioinks for skin regeneration and wound healing. Polymers (Basel), 13: 1011. https://doi.org/10.3390/polym13071011
17. Koch F, Wehrle M, Tröndle K, et al., 2019, Rapid Assessment of Combined Drop on Demand and Extrusion-based Bioprinting with Controlled Shear Stress and High Shape Fidelity. In: 2019 20th International Conference Solid-State Sensors, Actuators Microsystems Eurosensors XXXIII, Transducers 2019 Eurosensors XXXIII. Germany, IEEE. p1048–1051. https://doi.org/10.1109/TRANSDUCERS.2019.8808595
18. He P, Zhao J, Zhang J, et al., 2018, Bioprinting of skin constructs for wound healing. Burns Trauma, 6: 1–10. https://doi.org/10.1186/s41038-017-0104-x
19. Ghosh S, Kaushik G, Roy P, et al., 2021, Application of 3D bioprinting in wound healing: A review. Trends Biomater Artif Organs, 35: 495–509.
20. Wang Y, Yuan X, Yao B, et al., 2022, Tailoring bioinks of extrusion-based bioprinting for cutaneous wound healing. Bioact Mater, 17: 178–194. https://doi.org/10.1016/j.bioactmat.2022.01.024
21. Wang X, Ao Q, Tian X, et al., 2017, Gelatin-based hydrogels for organ 3D bioprinting. Polymers (Basel), 9: 401. https://doi.org/10.3390/polym9090401
22. Young S, Wong ME, Tabata Y, et al., 2005, Gelatin as a delivery vehicle for the controlled release of bioactive molecules. J Control Release, 109: 256–274. https://doi.org/10.1016/j.jconrel.2005.09.023
23. Badylak SF, Freytes DO, Gilbert TW, 2009, Extracellular matrix as a biological scaffold material: Structure and function. Acta Biomater, 5: 1–13. https://doi.org/10.1016/j.actbio.2008.09.013
24. Huang CY, Hu KH, Wei ZH, 2016, Comparison of cell behavior on pva/pva-gelatin electrospun nanofibers with random and aligned configuration. Sci Rep, 6: 37960. https://doi.org/10.1038/srep37960
25. Nguyen TH, Ventura R, Min YK, et al., 2016, Genipin cross-linked polyvinyl alcohol-gelatin hydrogel for bone regeneration. J Biomed Sci Eng, 9: 419–429. https://doi.org/10.4236/jbise.2016.99037
26. Bedran-Russo AK, Pereira PN, Duarte WR, et al., 2007, Application of crosslinkers to dentin collagen enhances the ultimate tensile strength. J Biomed Mater Res Part B Appl Biomater, 80: 268–272. https://doi.org/10.1002/jbm.b.30593
27. Fernandes DM, Barbosaa WS, Rangel WS, et al., 2021, Polymeric membrane based on polyactic acid and babassu oil for wound healing. Mater Today Commun, 26: 102173. https://doi.org/10.1016/j.mtcomm.2021.102173
28. Ghaffari R, Salimi-Kenari H, Fahimipour F, et al., 2020, Fabrication and characterization of dextran/nanocrystalline β-tricalcium phosphate nanocomposite hydrogel scaffolds. Int J Biol Macromol, 148: 434–448. https://doi.org/10.1016/j.ijbiomac.2020.01.112
29. Fauzi MB, Lokanathan Y, Nadzir MM, et al., 2017, Attachment, proliferation, and morphological properties of human dermal fibroblasts on ovine tendon collagen scaffolds: A comparative study. Malaysian J Med Sci, 24: 33–43. https://doi.org/10.21315/mjms2017.24.2.5
30. Maver T, Hribernik S, Mohan T, et al., 2015, Functional wound dressing materials with highly tunable drug release properties. RSC Adv, 5: 77873–77884. https://doi.org/10.1039/C5RA11972C
31. Rodríguez-Rodríguez R, García-Carvajal ZY, Jiménez- Palomar I, et al., 2019, Development of gelatin/chitosan/ PVA hydrogels: Thermal stability, water state, viscoelasticity, and cytotoxicity assays. J Appl Polym Sci, 136: 1–9. https://doi.org/10.1002/app.47149
32. Ghanbari M, Salavati-Niasari M, Mohandes F, 2021, Injectable hydrogels based on oxidized alginate-gelatin reinforced by carbon nitride quantum dots for tissue engineering. Int J Pharm, 602: 120660. https://doi.org/10.1016/j.ijpharm.2021.120660
33. Tan CT, Liang K, Ngo ZH, et al., 2020, Application of 3D bioprinting technologies to the management and treatment of diabetic foot ulcers. Biomedicines, 8: 441. https://doi.org/10.3390/biomedicines8100441
34. Huang S, Yao B, Xie J, et al., 2016, 3D bioprinted extracellular matrix mimics facilitate directed differentiation of epithelial progenitors for sweat gland regeneration. Acta Biomater, 32: 170–177. https://doi.org/10.1016/j.actbio.2015.12.039
35. He B, Wang J, Xie M, et al., 2022, 3D printed biomimetic epithelium/stroma bilayer hydrogel implant for corneal regeneration. Bioact Mater, 17: 234–247.
https://doi.org/10.1016/j.bioactmat.2022.01.034
36. Rodríguez-rodríguez R, Espinosa-andrews H, Velasquillo- Martínez C, et al., 2020, Composite hydrogels based on gelatin, chitosan and polyvinyl alcohol to biomedical applications : A review. Int J Polym Mater Polym Biomater, 69: 1–20. https://doi.org/10.1080/00914037.2019.1581780
37. De Stefano P, Briatico-Vangosa F, Bianchi E, et al., 2021, Bioprinting of matrigel scaffolds for cancer research. Polymers (Basel), 13: 2026. https://doi.org/10.3390/polym13122026
38. Chimene D, Lennox KK, Kaunas RR, et al., 2016, Advanced bioinks for 3D printing: A materials science perspective. Ann Biomed Eng, 44: 2090–2102. https://doi.org/10.1007/s10439-016-1638-y
39. Shie MY, Lee JJ, Ho CC, et al., 2020, Effects of gelatin methacrylate bio-ink concentration on mechano-physical properties and human dermal. Polymers (Basel), 2: 1930. https://doi.org/10.3390/polym12091930
40. Ding H, Chang RC, 2018, Printability study of bioprinted tubular structures using liquid hydrogel precursors in a support bath. Appl Sci, 8: 403. https://doi.org/10.3390/app8030403
41. Yang D, Li Y, Nie J, 2007, Preparation of gelatin/PVA nanofibers and their potential application in controlled release of drugs. Carbohydr Polym, 69: 538–543. https://doi.org/10.1016/j.carbpol.2007.01.008
42. Sgonc R, Gruber J, 2013, Age-related aspects of cutaneous wound healing: A mini-review. Gerontology, 59: 159–164. https://doi.org/10.1159/000342344
43. Zandi N, Dolatyar B, Lotfi R, et al., 2021, Biomimetic nanoengineered scaffold for enhanced full-thickness cutaneous wound healing. Acta Biomater, 124: 191–204. https://doi.org/10.1016/j.actbio.2021.01.029
44. Hezaveh H, Muhamad II, 2013, Controlled drug release via minimization of burst release in pH-response kappa-carrageenan/polyvinyl alcohol hydrogels. Chem Eng Res Des, 91: 508–519.
https://doi.org/10.1016/j.cherd.2012.08.014
45. Mahnama H, Dadbin S, Frounchi M, et al., 2017, Preparation of biodegradable gelatin/PVA porous scaffolds for skin regeneration. Artif Cells Nanomed Biotechnol, 45: 928–935. https://doi.org/10.1080/21691401.2016.1193025
46. Cheng L, Zou Q, Zou L, et al., 2013, Properties of vanillin modified poly(vinyl alcohol)/gelatin composite. Appl Mech Mater, 327: 48–52. https://doi.org/10.4028/www.scientific.net/AMM.327.48
47. Zidaric T, Milojevic M, Gradisnik L, et al., 2020, Polysaccharide-based bioink formulation for 3D bioprinting of an in vitro model of the human dermis. Nanomaterials, 10: 733. https://doi.org/10.3390/nano10040733
48. Naghieh S, Chen X, 2021, Printability-A key issue in extrusion-based bioprinting. J Pharm Anal, 11: 564–579. https://doi.org/10.1016/j.jpha.2021.02.001
49. Agubata CO, Mbah MA, Akpa PA, et al., 2021, Application of self-healing, swellable and biodegradable polymers for wound treatment. J Wound Care, 30: IVI–IVX. https://doi.org/10.12968/jowc.2021.30.Sup9a.IV
50. Jeong H, Lee DY, Yang DH, et al., 2022, Mechanical and cell-adhesive properties of gelatin/polyvinyl alcohol hydrogels and their application in wound dressing. Macromol Res, 30: 223–229. https://doi.org/10.1007/s13233-022-0027-7
51. Xu R, Xia H, He W, et al., 2016, Controlled water vapor transmission rate promotes wound-healing via wound re-epithelialization and contraction enhancement. Sci Rep, 6: 1–12. https://doi.org/10.1038/srep24596
52. Sutar T, Bangde P, Dandekar P, et al., 2021, Fabrication of herbal hemostat films loaded with medicinal tridax procumbenns extracts. Fibers Polym, 22: 2135–2144. https://doi.org/10.1007/s12221-021-0808-1
53. Lou CW, 2008, Process technology and properties evaluation of a chitosan-coated tencel/cotton nonwoven fabric as a wound dressing. Fibers Polym, 9: 286–292. https://doi.org/10.1007/s12221-008-0046-9
54. Gu Z, Xie H, Huang C, et al., 2013, Preparation of chitosan/ silk fibroin blending membrane fixed with alginate dialdehyde for wound dressing. Int J Biol Macromol, 58: 121–126. https://doi.org/10.1016/j.ijbiomac.2013.03.059
55. Butler MF, Ng YF, Pudney PD, 2003, Mechanism and kinetics of the crosslinking reaction between biopolymers containing primary amine groups and genipin. J Polym Sci Part A Polym Chem, 41: 3941–3953. https://doi.org/10.1002/pola.10960
56. Arif MM, Fauzi MB, Nordin A, et al., 2020, Fabrication of bio-based gelatin sponge for potential use as a functional acellular skin substitute. Polymers (Basel), 12: 1–19. https://doi.org/10.3390/polym12112678
57. Wang QS, Wang GF, Zhang HY, et al., 2021, Development of genipin crosslinked gelatin matrices on surface interaction: Enhancing the biocompatibility by attenuating sterile inflammation. Chin J Chem Eng, 38: 205–215. https://doi.org/10.1016/j.cjche.2021.03.022
58. Nike DU, Fadilah NI, Sallehuddin N, et al., 2022, Genipin-crosslinking effects on biomatrix development for cutaneous wound healing: A concise review. Front Bioeng Biotechnol, 10: 1–16. https://doi.org/10.3389/fbioe.2022.865014
59. Kim JM, Ko H, Kim SJ, et al., 2016, Chemopreventive properties of genipin on AGS cell line via induction of JNK/Nrf2/ARE signaling pathway. J Biochem Mol Toxicol, 30: 45–54. https://doi.org/10.1002/jbt.21741
60. Fan X, Lin L, Cui B, et al., 2020, Therapeutic potential of genipin in various acute liver injury, fulminant hepatitis, NAFLD and other non-cancer liver diseases: More friend than foe. Pharmacol Res, 159: 104945. https://doi.org/10.1016/j.phrs.2020.104945
61. Naseriyeh T, Noori T, Zhaleh H, et al., 2022, Enhanced in vitro cytotoxicity and intracellular uptake of genipin via loaded on nano-liposomes made from soybean lecithin in MCF-7 cells. Nanomed J, 9: 67–76. https://doi.org/10.22038/NMJ.2022.60524.1626
62. El-Habashy SE, El-Kamel AH, Essawy MM, et al., 2021, Engineering 3D-printed core-shell hydrogel scaffolds reinforced with hybrid hydroxyapatite/polycaprolactone nanoparticles for in vivo bone regeneration. Biomater Sci, 9: 4019–4039. https://doi.org/10.1039/d1bm00062d
63. Tung NT, Vu VD, Nguyen PL, 2019, DoE-based development, physicochemical characterization, and pharmacological evaluation of a topical hydrogel containing betamethasone dipropionate microemulsion. Colloids Surf B Biointerfaces, 181: 480–488. https://doi.org/10.1016/j.colsurfb.2019.06.002
64. Masri S, Maarof M, Mohd NF, et al., 2022, Injectable crosslinked genipin hybrid gelatin-PVA hydrogels for future use as bioinks in expediting cutaneous healing capacity : Physicochemical characterisation and cytotoxicity evaluation. Biomedicine, 10: 2651. https://doi.org/10.3390/biomedicines10102651
65. Moraes IC, Carvalho RA, Bittante AM, et al., 2009, Film forming solutions based on gelatin and poly(vinyl alcohol) blends: Thermal and rheological characterizations. J Food Eng, 95: 588–596. https://doi.org/10.1016/j.jfoodeng.2009.06.023
66. Gelli R, Del Buffa S, Tempesti P, et al., 2017, Multi-scale investigation of gelatin/poly(vinyl alcohol) interactions in water. Colloids Surf A Physicochem Eng Asp, 532: 18–25. https://doi.org/10.1016/j.colsurfa.2017.07.049
67. Zandraa O, Ngwabebhoh FA, Patwa R, et al., 2021, Development of dual crosslinked mumio-based hydrogel dressing for wound healing application: Physico-chemistry and antimicrobial activity. Int J Pharm, 607: 120952. https://doi.org/10.1016/j.ijpharm.2021.120952
68. Swaroop K, Gaana MJ, Shruthi SS, et al., 2019, Studies on swelling behaviour of radiolytically synthesised PVA/gelatin hydrogels. AIP Conf Proc, 2115: 030050. https://doi.org/10.1063/1.5112889
69. Zawani M, Maarof M, Tabata Y, et al., 2022, Quercetin-embedded gelastin injectable hydrogel as provisional biotemplate for future cutaneous application: Optimization and in vitro evaluation. Gels, 8: 623. https://doi.org/10.3390/gels8100623
70. Thangprasert A, Tansakul C, Thuaksubun N, et al., 2019, Mimicked hybrid hydrogel based on gelatin/PVA for tissue engineering in subchondral bone interface for osteoarthritis surgery. Mater Des, 183: 108113. https://doi.org/10.1016/j.matdes.2019.108113
71. Yannas IV, Lee E, Orgill DP, et al., 1989, Synthesis and characterization of a model extracellular matrix that induces partial regeneration of adult mammalian skin. Proc Natl Acad Sci USA, 86: 933–937.
72. Labus K, Radosinski L, Kotowski P, 2021, Functional properties of two-component hydrogel systems based on gelatin and polyvinyl alcohol-experimental studies supported by computational analysis. Int J Mol Sci, 22: 9909. https://doi.org/10.3390/ijms22189909
73. Erdag D, Koc SN, Oksuzomer MF, et al., 2021, Synergistic effect of selenium and genipin triggers viability of 3T3 cells on PVA/gelatin scaffolds. Acta Bioeng Biomech, 24: 179–190. https://doi.org/10.37190/ABB-01944-2021-04
74. Barba BJ, Oyama TG, Taguchi M, 2021, Simple fabrication of gelatin-polyvinyl alcohol bilayer hydrogel with wound dressing and nonadhesive duality. Polym Adv Technol, 32: 4406–4414. https://doi.org/10.1002/pat.5442
75. George B, Bhatia N, Kumar A, et al., 2022, Bioinspired gelatin based sticky hydrogel for diverse surfaces in burn wound care. Sci Rep, 12: 13735. https://doi.org/10.1038/s41598-022-17054-w
76. Kakarla AB, Kong I, Turek I, et al., 2022, Printable gelatin, alginate and boron nitride nanotubes hydrogel-based ink for 3D bioprinting and tissue engineering applications. Mater Des, 213: 110362. https://doi.org/10.1016/j.matdes.2021.110362
77. Crosby CO, Stern B, Kalkunte N, et al., 2022, Interpenetrating polymer network hydrogels as bioactive scaffolds for tissue engineering. Rev Chem Eng, 38: 347–361. https://doi.org/10.1515/revce-2020-0039