Bioprinting of exosomes: Prospects and challenges for clinical applications
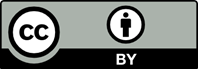
Three-dimensional bioprinting (3DBP) is an additive manufacturing technique that has emerged as a promising strategy for the fabrication of scaffolds, which can successfully recapitulate the architectural, biochemical, and physical cues of target tissues. More importantly, 3DBP offers fine spatiotemporal control and high submicron scale resolution, which can be leveraged for the incorporation and directional gradient release of single or multiple biomimetic cues, including cell-derived exosomes (EXOs). EXOs are extracellular vesicles that originate from the endosomal compartment of various cell types, with sizes ranging from 30 to120 nm. They act as cell mediators and contain discrete cell constituents, including growth factors, cytokines, lipid moieties, nucleic acids, metabolites, and cell surface markers, depending on the cell type. Essentially, owing to their therapeutic potential, EXOs derived from mesenchymal stem cells (MSCs) have been recently investigated in several clinical trials for the treatment of various conditions, including cancer, diabetes, dry eyes, periodontitis, and acute ischemic stroke. The 3DBP strategy of EXOs is especially useful in tissue engineering and regenerative medicine applications, as tissues can be biofabricated to closely mimic the complex microarchitecture and developmental profiles of native heterogeneous tissues for restoring biological functions. Moreover, EXOs can be manipulated to carry exogenous cargo such as genes or proteins of therapeutic interest, confer multifunctional attributes, and further enhance their tissue regenerative potential. However, significant challenges, including the selection of appropriate bioink, pattern resolution, engineering-defined exosomal gradient, spatial presentation and modulation of EXO release kinetics, as well as EXO stability and storage conditions, must be addressed for the successful translation of therapeutic grade EXOs to clinical settings. In this review, we highlight the recent advances and offer future perspectives on the bioprinting of EXOs as regenerative biotherapeutics for the fabrication of complex heterogeneous tissues that are suitable for clinical transplantation.
1. Doyle LM, Wang MZ, 2019, Overview of extracellular vesicles, their origin, composition, purpose, and methods for exosome isolation and analysis. Cells, 8: 727. https://doi.org/10.3390/cells8070727
2. Lin J, Li J, Huang B, et al., 2015, Exosomes: Novel biomarkers for clinical diagnosis. Sci World J, 2015: 657086. https://doi.org/10.1155/2015/657086
3. Bang C, Thum T, 2012, Exosomes: New players in cell-cell communication. Int J Biochem Cell Biol, 44: 2060–2064. https://doi.org/10.1016/j.biocel.2012.08.007
4. Li N, Rochette L, Wu Y, et al., 2019, New insights into the role of exosomes in the heart after myocardial infarction. J Cardiovasc Transl Res, 12: 18–27. https://doi.org/10.1007/s12265-018-9831-z
5. Kim YG, Choi J, Kim K, 2020, Mesenchymal stem cell-derived exosomes for effective cartilage tissue repair and treatment of osteoarthritis. Biotechnol J, 15: e2000082. https://doi.org/10.1002/biot.202000082
6. Hur YH, Feng S, Wilson KF, et al., 2021, Embryonic stem cell-derived extracellular vesicles maintain ESC stemness by activating FAK. Dev Cell, 56: 277–291.e6. https://doi.org/10.1016/j.devcel.2020.11.017
7. Feng Z, Zhou J, Liu Y, et al., 2021, Epithelium- and endothelium-derived exosomes regulate the alveolar macrophages by targeting RGS1 mediated calcium signaling-dependent immune response. Cell Death Differ, 28: 2238–2256. https://doi.org/10.1038/s41418-021-00750-x
8. Zamani P, Fereydouni N, Butler AE, et al., 2019, The therapeutic and diagnostic role of exosomes in cardiovascular diseases. Trends Cardiovasc Med, 29: 313–323. https://doi.org/10.1016/j.tcm.2018.10.010
9. Liu J, Jiang F, Jiang Y, et al., 2020, Roles of exosomes in ocular diseases. Int J Nanomedicine, 15: 10519–10538. https://doi.org/10.2147/ijn.S277190
10. Hill AF, 2019, Extracellular vesicles and neurodegenerative diseases. J Neurosci, 39: 9269–9273. https://doi.org/10.1523/jneurosci.0147-18.2019
11. Castaño C, Novials A, Párrizas M, 2019, Exosomes and diabetes. Diabetes Metab Res Rev, 35: e3107. https://doi.org/10.1002/dmrr.3107
12. Tavasolian F, Moghaddam AS, Rohani F, et al., 2020, Exosomes: Effectual players in rheumatoid arthritis. Autoimmun Rev, 19: 102511. https://doi.org/10.1016/j.autrev.2020.102511
13. Phinney DG, Pittenger MF, 2017, Concise review: MSC-derived exosomes for cell-free therapy. Stem Cells, 35: 851–858. https://doi.org/10.1002/stem.2575
14. Perocheau D, Touramanidou L, Gurung S, et al., 2021, Clinical applications for exosomes: Are we there yet? Br J Pharmacol, 178: 2375–2392. https://doi.org/10.1111/bph.15432
15. Baran J, Baj-Krzyworzeka M, Weglarczyk K, et al., 2010, Circulating tumour-derived microvesicles in plasma of gastric cancer patients. Cancer Immunol Immunother, 59: 841–850. https://doi.org/10.1007/s00262-009-0808-2
16. Bebelman MP, Janssen E, Pegtel DM, et al., 2021, The forces driving cancer extracellular vesicle secretion. Neoplasia, 23: 149–157. https://doi.org/10.1016/j.neo.2020.11.011
17. Whiteside TL, 2017, Exosomes carrying immunoinhibitory proteins and their role in cancer. Clin Exp Immunol, 189: 259–267. https://doi.org/10.1111/cei.12974
18. Mohammadi MR, Rodriguez SM, Luong JC, et al., 2021, Exosome loaded immunomodulatory biomaterials alleviate local immune response in immunocompetent diabetic mice post islet xenotransplantation. Commun Biol, 4: 685. https://doi.org/10.1038/s42003-021-02229-4
19. Fan L, Guan P, Xiao C, et al., 2021, Exosome-functionalized polyetheretherketone-based implant with immunomodulatory property for enhancing osseointegration. Bioact Mater, 6: 2754–2766. https://doi.org/10.1016/j.bioactmat.2021.02.005
20. Liang Y, Duan L, Lu J, et al., 2021, Engineering exosomes for targeted drug delivery. Theranostics, 11: 3183–3195. https://doi.org/10.7150/thno.52570
21. Vonk LA, van Dooremalen SFJ, Liv N, et al., 2018, Mesenchymal stromal/stem cell-derived extracellular vesicles promote human cartilage regeneration in vitro. Theranostics, 8: 906–920. https://doi.org/10.7150/thno.20746
22. Xia B, Gao J, Li S, et al., 2020, Mechanical stimulation of Schwann cells promote peripheral nerve regeneration via extracellular vesicle-mediated transfer of microRNA 23b- 3p. Theranostics, 10: 8974–8995. https://doi.org/10.7150/thno.44912
23. Elsharkasy OM, Nordin JZ, Hagey DW, et al., 2020, Extracellular vesicles as drug delivery systems: Why and how? Adv Drug Deliv Rev, 159: 332–343. https://doi.org/10.1016/j.addr.2020.04.004
24. Arrighetti N, Corbo C, Evangelopoulos M, et al., 2019, Exosome-like nanovectors for drug delivery in cancer. Curr Med Chem, 26: 6132–6148. https://doi.org/10.2174/0929867325666180831150259
25. Zhang B, Wang M, Gong A, et al., 2015, HucMSC-exosome mediated-Wnt4 signaling is required for cutaneous wound healing. Stem Cells, 33: 2158–2168. https://doi.org/10.1002/stem.1771
26. Shabbir A, Cox A, Rodriguez-Menocal L, et al., 2015, Mesenchymal stem cell exosomes induce proliferation and migration of normal and chronic wound fibroblasts, and enhance angiogenesis in vitro. Stem Cells Dev, 24: 1635–1647. https://doi.org/10.1089/scd.2014.0316
27. Geiger A, Walker A, Nissen E, 2015, Human fibrocyte-derived exosomes accelerate wound healing in genetically diabetic mice. Biochem Biophys Res Commun, 467: 303–309. https://doi.org/10.1016/j.bbrc.2015.09.166
28. Moghaddam AS, Afshari JT, Esmaeili S-A, et al., 2019, Cardioprotective microRNAs: Lessons from stem cell-derived exosomal microRNAs to treat cardiovascular disease. Atherosclerosis, 285: 1–9. https://doi.org/10.1016/j.atherosclerosis.2019.03.016
29. Wang Y, Zhang Q, Yang G, et al., 2021, RPE-derived exosomes rescue the photoreceptors during retina degeneration: An intraocular approach to deliver exosomes into the subretinal space. Drug Deliv, 28: 218–228. https://doi.org/10.1080/10717544.2020.1870584
30. Yu B, Shao H, Su C, et al., 2016, Exosomes derived from MSCs ameliorate retinal laser injury partially by inhibition of MCP-1. Sci Rep, 6: 34562. https://doi.org/10.1038/srep34562
31. Doeppner TR, Herz J, Görgens A, et al., 2015, Extracellular vesicles improve post-stroke neuroregeneration and prevent postischemic immunosuppression. Stem Cells Transl Med, 4: 1131–1143. https://doi.org/10.5966/sctm.2015-0078
32. Kang M, Huang CC, Lu Y, et al., 2020, Bone regeneration is mediated by macrophage extracellular vesicles. Bone, 141: 115627. https://doi.org/10.1016/j.bone.2020.115627
33. Zhou M, Li B, Liu C, et al., 2021, M2 Macrophage-derived exosomal miR-501 contributes to pubococcygeal muscle regeneration. Int Immunopharmacol, 101: 108223. https://doi.org/10.1016/j.intimp.2021.108223
34. Deng F, Yan J, Lu J, et al., 2021, M2 macrophage-derived exosomal miR-590-3p attenuates DSS-induced mucosal damage and promotes epithelial repair via the LATS1/YAP/ β-Catenin signalling axis. J Crohns Colitis, 15: 665–677. https://doi.org/10.1093/ecco-jcc/jjaa214
35. Zhang H, Wang R, Wang G, et al., 2021, Single-cell RNA sequencing reveals B cells are important regulators in fracture healing. Front Endocrinol (Lausanne), 12: 666140. https://doi.org/10.3389/fendo.2021.666140
36. Liu H, Gao W, Yuan J, et al., 2016, Exosomes derived from dendritic cells improve cardiac function via activation of CD4(+) T lymphocytes after myocardial infarction. J Mol Cell Cardiol, 91: 123–133. https://doi.org/10.1016/j.yjmcc.2015.12.028
37. Okoye IS, Coomes SM, Pelly VS, et al., 2014, MicroRNA-containing T-regulatory-cell-derived exosomes suppress pathogenic T helper 1 cells. Immunity, 41: 89–103. https://doi.org/10.1016/j.immuni.2014.05.019
38. Tung SL, Boardman DA, Sen M, et al., 2018, Regulatory T cell-derived extracellular vesicles modify dendritic cell function. Sci Rep, 8: 6065. https://doi.org/10.1038/s41598-018-24531-8
39. Hu H, Wu J, Cao C, et al., 2020, Exosomes derived from regulatory T cells ameliorate acute myocardial infarction by promoting macrophage M2 polarization. IUBMB Life, 72: 2409–2419. https://doi.org/10.1002/iub.2364
40. Liu M, Liu Z, Chen Y, et al., 2022, Dendritic epidermal T cells secreting exosomes promote the proliferation of epidermal stem cells to enhance wound re-epithelialization. Stem Cell Res Ther, 13: 121. https://doi.org/10.1186/s13287-022-02783-6
41. Al Halawani A, Mithieux SM, Yeo GC, et al., 2022, Extracellular vesicles: Interplay with the extracellular matrix and modulated cell responses. Int J Mol Sci, 23: 3389. https://doi.org/10.3390/ijms23063389
42. Rackov G, Garcia-Romero N, Esteban-Rubio S, et al., 2018, Vesicle-mediated control of cell function: The role of extracellular matrix and microenvironment. Front Physiol, 9: 651. https://doi.org/10.3389/fphys.2018.00651
43. Buzás EI, Tóth E, Sódar BW, et al., 2018, Molecular interactions at the surface of extracellular vesicles. Semin Immunopathol, 40: 453–464. https://doi.org/10.1007/s00281-018-0682-0
44. Sung BH, Ketova T, Hoshino D, et al., 2015, Directional cell movement through tissues is controlled by exosome secretion. Nat Commun, 6: 7164. https://doi.org/10.1038/ncomms8164
45. Purushothaman A, Bandari SK, Liu J, et al., 2016, Fibronectin on the surface of myeloma cell-derived exosomes mediates exosome-cell interactions. J Biol Chem, 291: 1652–1663. https://doi.org/10.1074/jbc.M115.686295
46. Shen AR, Zhong X, Tang TT, et al., 2020, Integrin, exosome and kidney disease. Front Physiol, 11: 627800. https://doi.org/10.3389/fphys.2020.627800
47. Hoshino A, Costa-Silva B, Shen T-L, et al., 2015, Tumour exosome integrins determine organotropic metastasis. Nature, 527: 329–335. https://doi.org/10.1038/nature15756
48. Christianson HC, Svensson KJ, van Kuppevelt TH, et al., 2013, Cancer cell exosomes depend on cell-surface heparan sulfate proteoglycans for their internalization and functional activity. Proc Natl Acad Sci U S A, 110: 17380–17385. https://doi.org/10.1073/pnas.1304266110
49. Arasu UT, Kärnä R, Härkönen K, et al., 2017, Human mesenchymal stem cells secrete hyaluronan-coated extracellular vesicles. Matrix Biol, 64: 54–68. https://doi.org/10.1016/j.matbio.2017.05.001
50. Shimoda M, Khokha R, 2017, Metalloproteinases in extracellular vesicles. Biochim Biophys Acta Mol Cell Res, 1864: 1989–2000. https://doi.org/10.1016/j.bbamcr.2017.05.027
51. Sajeesh S, Broekelman T, Mecham RP, et al., 2020, Stem cell derived extracellular vesicles for vascular elastic matrix regenerative repair. Acta Biomater, 113: 267–278. https://doi.org/10.1016/j.actbio.2020.07.002
52. Woo CH, Kim HK, Jung GY, et al., 2020, Small extracellular vesicles from human adipose-derived stem cells attenuate cartilage degeneration. J Extracell Vesicles, 9: 1735249. https://doi.org/10.1080/20013078.2020.1735249
53. Li JJ, Hosseini-Beheshti E, Grau GE, et al., 2019, Stem cell-derived extracellular vesicles for treating joint injury and osteoarthritis. Nanomaterials (Basel), 9: 261. https://doi.org/10.3390/nano9020261
54. Zhang S, Chu WC, Lai RC, et al., 2016, Exosomes derived from human embryonic mesenchymal stem cells promote osteochondral regeneration. Osteoarthr Cartil, 24: 2135–2140. https://doi.org/10.1016/j.joca.2016.06.022
55. Huang J, Xiong J, Yang L, et al., 2021, Cell-free exosome-laden scaffolds for tissue repair. Nanoscale, 13: 8740–8750. https://doi.org/10.1039/D1NR01314A
56. Shafei S, Khanmohammadi M, Heidari R, et al., 2020, Exosome loaded alginate hydrogel promotes tissue regeneration in full-thickness skin wounds: An in vivo study. J Biomed Mater Res A, 108: 545–556. https://doi.org/10.1002/jbm.a.36835
57. Yang S, Zhu B, Yin P, et al., 2020, Integration of human umbilical cord mesenchymal stem cells-derived exosomes with hydroxyapatite-embedded hyaluronic acid-alginate hydrogel for bone regeneration. ACS Biomater Sci Eng, 6: 1590–1602. https://doi.org/10.1021/acsbiomaterials.9b01363
58. Liu B, Lee BW, Nakanishi K, et al., 2018, Cardiac recovery via extended cell-free delivery of extracellular vesicles secreted by cardiomyocytes derived from induced pluripotent stem cells. Nat Biomed Eng, 2: 293–303. https://doi.org/10.1038/s41551-018-0229-7
59. Chen CW, Wang LL, Zaman S, et al., 2018, Sustained release of endothelial progenitor cell-derived extracellular vesicles from shear-thinning hydrogels improves angiogenesis and promotes function after myocardial infarction. Cardiovasc Res, 114: 1029–1040. https://doi.org/10.1093/cvr/cvy067
60. Shi Q, Qian Z, Liu D, et al., 2017, GMSC-derived exosomes combined with a chitosan/silk hydrogel sponge accelerates wound healing in a diabetic rat skin defect model. Front Physiol, https://doi.org/8:10.3389/fphys.2017.00904
61. Gu Z, Fu J, Lin H, et al., 2020, Development of 3D bioprinting: From printing methods to biomedical applications. Asian J Pharm Sci, 15: 529–557. https://doi.org/10.1016/j.ajps.2019.11.003
62. Gao B, Yang Q, Zhao X, et al., 2016, 4D bioprinting for biomedical applications. Trends Biotechnol, 34: 746–756. https://doi.org/10.1016/j.tibtech.2016.03.004
63. Kolesky DB, Homan KA, Skylar-Scott MA, et al., 2016, Three-dimensional bioprinting of thick vascularized tissues. Proc Natl Acad Sci U S A, 113: 3179–3184. https://doi.org/10.1073/pnas.1521342113
64. Liu X, Wang X, Zhang L, et al., 2021, 3D liver tissue model with branched vascular networks by multimaterial bioprinting. Adv Healthc Mater, 10: 2101405. https://doi.org/10.1002/adhm.202101405
65. Bittner SM, Guo JL, Mikos AG, 2018, Spatiotemporal control of growth factors in three-dimensional printed scaffolds. Bioprinting, 12. https://doi.org/10.1016/j.bprint.2018.e00032
66. Castro NJ, O’Brien J, Zhang LG, 2015, Integrating biologically inspired nanomaterials and table-top stereolithography for 3D printed biomimetic osteochondral scaffolds. Nanoscale, 7: 14010–14022. https://doi.org/10.1039/c5nr03425f
67. Freeman FE, Pitacco P, van Dommelen LHA, et al., 2020, 3D bioprinting spatiotemporally defined patterns of growth factors to tightly control tissue regeneration. Sci Adv, 6: eabb5093. https://doi.org/10.1126/sciadv.abb5093
68. Johnson BN, Lancaster KZ, Zhen G, et al., 2015, 3D printed anatomical nerve regeneration pathways. Adv Funct Mater, 25: 6205–6217. https://doi.org/10.1002/adfm.201501760
69. Epstein NE, 2013, Complications due to the use of BMP/ INFUSE in spine surgery: The evidence continues to mount. Surg Neurol Int, 4: S343–S352. https://doi.org/10.4103/2152-7806.114813
70. Shields LB, Raque GH, Glassman SD, et al., 2006, Adverse effects associated with high-dose recombinant human bone morphogenetic protein-2 use in anterior cervical spine fusion. Spine (Phila Pa 1976), 31: 542–547. https://doi.org/10.1097/01.brs.0000201424.27509.72
71. Shenoda BB, Ajit SK, 2016, Modulation of immune responses by exosomes derived from antigen-presenting cells. Clin Med Insights Pathol, 9: 1–8. https://doi.org/10.4137/CPath.S39925
72. Gupta D, Zickler AM, El Andaloussi S, 2021, Dosing extracellular vesicles. Adv Drug Deliv Rev, 178: 113961. https://doi.org/10.1016/j.addr.2021.113961
73. Zheng D, Huo M, Li B, et al., 2020, The role of exosomes and exosomal microRNA in cardiovascular disease. Front Cell Dev Biol, 8: 616161. https://doi.org/10.3389/fcell.2020.616161
74. Yerneni SS, Whiteside TL, Weiss LE, et al., 2019, Bioprinting exosome-like extracellular vesicle microenvironments. Bioprinting, 13: e00041. https://doi.org/10.1016/j.bprint.2019.e00041
75. Chen P, Zheng L, Wang Y, et al., 2019, Desktop-stereolithography 3D printing of a radially oriented extracellular matrix/mesenchymal stem cell exosome bioink for osteochondral defect regeneration. Theranostics, 9: 2439–2459. https://doi.org/10.7150/thno.31017
76. Zha Y, Li Y, Lin T, et al., 2021, Progenitor cell-derived exosomes endowed with VEGF plasmids enhance osteogenic induction and vascular remodeling in large segmental bone defects. Theranostics, 11: 397–409. https://doi.org/10.7150/thno.50741
77. Bari E, Scocozza F, Perteghella S, et al., 2021, 3D bioprinted scaffolds containing mesenchymal stem/stromal lyosecretome: Next generation controlled release device for bone regenerative medicine. Pharmaceutics, 13: 515. https://doi.org/10.3390/pharmaceutics13040515
78. Wang C, Wang M, Xu T, et al., 2019, Engineering bioactive self-healing antibacterial exosomes hydrogel for promoting chronic diabetic wound healing and complete skin regeneration. Theranostics, 9: 65–76. 10.7150/thno.29766
79. Tao SC, Guo SC, Li M, et al., 2017, Chitosan wound dressings incorporating exosomes derived from microRNA-126- overexpressing synovium mesenchymal stem cells provide sustained release of exosomes and heal full-thickness skin defects in a diabetic rat model. Stem Cells Transl Med, 6: 736–747. https://doi.org/10.5966/sctm.2016-0275
80. Guo SC, Tao SC, Yin WJ, et al., 2017, Exosomes derived from platelet-rich plasma promote the re-epithelization of chronic cutaneous wounds via activation of YAP in a diabetic rat model. Theranostics, 7: 81–96. https://doi.org/10.7150/thno.16803
81. Han C, Zhou J, Liang C, et al., 2019, Human umbilical cord mesenchymal stem cell derived exosomes encapsulated in functional peptide hydrogels promote cardiac repair. Biomater Sci, 7: 2920–2933. https://doi.org/10.1039/c9bm00101h
82. Murphy SV, Atala A, 2014, 3D bioprinting of tissues and organs. Nat Biotechnol, 32: 773–785. https://doi.org/10.1038/nbt.2958
83. Maumus M, Rozier P, Boulestreau J, et al., 2020, Mesenchymal stem cell-derived extracellular vesicles: Opportunities and challenges for clinical translation. Front Bioeng Biotechnol, 8. https://doi.org/10.3389/fbioe.2020.00997
84. Eslani M, Putra I, Shen X, et al., 2017, Corneal mesenchymal stromal cells are directly antiangiogenic via PEDF and sFLT- 1. Invest Ophthalmol Vis Sci, 58: 5507–5517. https://doi.org/10.1167/iovs.17-22680
85. Hoang DH, Nguyen TD, Nguyen H-P, et al., 2020, Differential wound healing capacity of mesenchymal stem cell-derived exosomes originated from bone marrow, adipose tissue and umbilical cord under serum- and xeno-free condition. Front Mol Biosci, 7: 119. https://doi.org/10.3389/fmolb.2020.00119
86. Lopez-Verrilli MA, Caviedes A, Cabrera A, et al., 2016, Mesenchymal stem cell-derived exosomes from different sources selectively promote neuritic outgrowth. Neuroscience, 320: 129–139. https://doi.org/10.1016/j.neuroscience.2016.01.061
87. Piffoux M, Nicolás-Boluda A, Mulens-Arias V, et al., 2019, Extracellular vesicles for personalized medicine: The input of physically triggered production, loading and theranostic properties. Adv Drug Deliv Rev, 138: 247–258. https://doi.org/10.1016/j.addr.2018.12.009
88. Zhang X-F, Wang T, Wang Z-X, et al., 2021, Hypoxic ucMSC-secreted exosomal miR-125b promotes endothelial cell survival and migration during wound healing by targeting TP53INP1. Mol Ther Nucleic Acids, 26: 347–359. https://doi.org/10.1016/j.omtn.2021.07.014
89. Lener T, Gimona M, Aigner L, et al., 2015, Applying extracellular vesicles based therapeutics in clinical trials – an ISEV position paper. J Extracell Vesicles, 4: 30087. https://doi.org/10.3402/jev.v4.30087
90. Ahn SH, Ryu SW, Choi H, et al., 2022, Manufacturing therapeutic exosomes: From bench to industry. Mol Cells, 45: 284–290. https://doi.org/10.14348/molcells.2022.2033
91. Andreu Z, Rivas E, Sanguino-Pascual A, et al., 2016, Comparative analysis of EV isolation procedures for miRNAs detection in serum samples. J Extracell Vesicles, 5: 31655. https://doi.org/10.3402/jev.v5.31655
92. Busatto S, Vilanilam G, Ticer T, et al., 2018, Tangential flow filtration for highly efficient concentration of extracellular vesicles from large volumes of fluid. Cells, 7. https://doi.org/10.3390/cells7120273
93. Lobb RJ, Becker M, Wen SW, et al., 2015, Optimized exosome isolation protocol for cell culture supernatant and human plasma. J Extracell Vesicles, 4: 27031. https://doi.org/10.3402/jev.v4.27031
94. Kosanović M, Milutinović B, Goč S, et al., 2017, Ion-exchange chromatography purification of extracellular vesicles. Biotechniques, 63: 65–71. https://doi.org/10.2144/000114575
95. Greening DW, Xu R, Ji H, et al., 2015, A protocol for exosome isolation and characterization: Evaluation of ultracentrifugation, density-gradient separation, and immunoaffinity capture methods. Methods Mol Biol, 1295: 179–209. https://doi.org/10.1007/978-1-4939-2550-6_15
96. Corso G, Mäger I, Lee Y, et al., 2017, Reproducible and scalable purification of extracellular vesicles using combined bind-elute and size exclusion chromatography. Sci Rep, 7: 11561. https://doi.org/10.1038/s41598-017-10646-x
97. Oeyen E, Van Mol K, Baggerman G, et al., 2018, Ultrafiltration and size exclusion chromatography combined with asymmetrical-flow field-flow fractionation for the isolation and characterisation of extracellular vesicles from urine. J Extracell Vesicles, 7: 1490143. https://doi.org/10.1080/20013078.2018.1490143
98. Maroto R, Zhao Y, Jamaluddin M, et al., 2017, Effects of storage temperature on airway exosome integrity for diagnostic and functional analyses. J Extracell Vesicles, 6: 1359478. https://doi.org/10.1080/20013078.2017.1359478
99. Yuan F, Li YM, Wang Z, 2021, Preserving extracellular vesicles for biomedical applications: Consideration of storage stability before and after isolation. Drug Deliv, 28: 1501–1509. https://doi.org/10.1080/10717544.2021.1951896
100. Chen YS, Lin EY, Chiou TW, et al., 2020, Exosomes in clinical trial and their production in compliance with good manufacturing practice. Ci Ji Yi Xue Za Zhi, 32: 113–120. https://doi.org/10.4103/tcmj.tcmj_182_19
101. Budgude P, Kale V, Vaidya A, 2021, Cryopreservation of mesenchymal stromal cell-derived extracellular vesicles using trehalose maintains their ability to expand hematopoietic stem cells in vitro. Cryobiology, 98: 152–163. https://doi.org/10.1016/j.cryobiol.2020.11.009
102. Bosch S, de Beaurepaire L, Allard M, et al., 2016, Trehalose prevents aggregation of exosomes and cryodamage. Sci Rep, 6: 36162. https://doi.org/10.1038/srep36162
103. Charoenviriyakul C, Takahashi Y, Nishikawa M, et al., 2018, Preservation of exosomes at room temperature using lyophilization. Int J Pharm, 553: 1–7. https://doi.org/10.1016/j.ijpharm.2018.10.032