A biocompatible double-crosslinked gelatin/ sodium alginate/dopamine/quaterniazed chitosan hydrogel for wound dressings based on 3D bioprinting technology
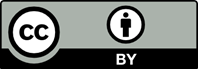
Severe skin injuries can cause serious problems, which could affect the patient’s normal life, if not dealt properly in a timely and effective manner. It is an urgent requirement to develop personalized wound dressings with excellent antibacterial activity and biocompatibility to match the shape of the wound to facilitate clinical application. In this study, a bioink (GAQ) based on gelatin (Gel)/sodium alginate (SA)/ quaternized chitosan (QCS) was prepared, and GAQ hydrogel dressing grafting with dopamine (GADQ) was fabricated by an extrusion three-dimensional (3D) printing technology. QCS was synthesized by modifying quaternary ammonium group on chitosan, and its structure was successfully characterized by nuclear magnetic resonance (1H NMR) and Fourier-transform infrared spectroscopy (FT-IR). Our results showed that the GADQ hydrogel dressing that was double-crosslinked by EDC/ NHS and Ca2+ had good tensile strength, considerable swelling ratio, and effective antioxidation properties. It also showed that GADQ1.5% had 93.17% and 91.06% antibacterial activity against Staphylococcus aureus and Escherichia coli, respectively. Furthermore, the relative survival ratios of fibroblast cells seeded on these hydrogels exceeded 350% after cultured for 7 days, which proved the biocompatibility of these hydrogels. Overall, this advanced 3D-printed GADQ1.5% hydrogels with effective antioxidation, excellent antibacterial activity and good biocompatibility had a considerable application potential for wound healing.
1. Zhao X, Wu H, Guo B, et al., 2017, Antibacterial anti-oxidant electroactive injectable hydrogel as self-healing wound dressing with hemostasis and adhesiveness for cutaneous wound healing. Biomaterials, 122:34–47. https://doi.org/10.1016/j.biomaterials.2017.01.011
2. Wang X, Qi J, Zhang W, et al., 2021, 3D-printed antioxidant antibacterial carboxymethyl cellulose/epsilon-polylysine hydrogel promoted skin wound repair. Int J Biol Macromol, 187:91–104. https://doi.org/10.1016/j.ijbiomac.2021.07.115
3. Feng Z, Su Q, Zhang C, et al., 2020, Bioinspired nanofibrous glycopeptide hydrogel dressing for accelerating wound healing: A cytokine-free, M2-type macrophage polarization approach. Adv Funct Mater, 30(52):2006454. https://doi.org/10.1002/adfm.202006454
4. Liu G, Zhou Y, Xu Z, et al., 2022, Janus hydrogel with dual antibacterial and angiogenesis functions for enhanced diabetic wound healing. Chin Chem Lett. https://doi.org/10.1016/j.cclet.2022.07.048
5. Xian C, Zhang Z, You X, et al., 2022, Nanosized fat emulsion injection modulating local microenvironment promotes angiogenesis in chronic wound healing. Adv Funct Mater, 32(32):2202410. https://doi.org/10.1002/adfm.202202410
6. Zakerikhoob M, Abbasi S, Yousefi G, et al., 2021, Curcumin-incorporated crosslinked sodium alginate-g-poly (N-isopropyl acrylamide) thermo-responsive hydrogel as an in-situ forming injectable dressing for wound healing: In vitro characterization and in vivo evaluation. Carbohydr Polym, 271:118434. https://doi.org/10.1016/j.carbpol.2021.118434
7. Li M, Liang Y, He J, et al., 2020, Two-pronged strategy of biomechanically active and biochemically multifunctional hydrogel wound dressing to accelerate wound closure and wound healing. Chem Mater, 32(23):9937–9953. https://doi.org/10.1021/acs.chemmater.0c02823
8. Tran PL, Hamood AN, de Souza A, et al., 2015, A study on the ability of quaternary ammonium groups attached to a polyurethane foam wound dressing to inhibit bacterial attachment and biofilm formation. Wound Repair Regen, 23(1):74–81. https://doi.org/10.1111/wrr.12244
9. Li J, Zhai D, Lv F, et al., 2016, Preparation of copper-containing bioactive glass/eggshell membrane nanocomposites for improving angiogenesis, antibacterial activity and wound healing. Acta Biomater, 36:254–266. https://doi.org/10.1016/j.actbio.2016.03.011
10. Fan Z, Liu B, Wang J, et al., 2014, A novel wound dressing based on Ag/graphene polymer hydrogel: Effectively kill bacteria and accelerate wound healing. Adv Funct Mater, 24(25):3933–3943. https://doi.org/10.1002/adfm.201304202
11. Rieger KA, Birch NP, Schiffman JD, 2013, Designing electrospun nanofiber mats to promote wound healing—A review. J Mater Chem B, 1(36):4531–4541. http://doi.org/10.1039/c3tb20795a
12. Shu W, Wang Y, Zhang X, et al., 2021, Functional hydrogel dressings for treatment of burn wounds. Front Bioeng Biotech, 9:788461. https://doi.org/10.3389/fbioe.2021.788461
13. Wang L, Zhou M, Xu T, et al., 2022, Multifunctional hydrogel as wound dressing for intelligent wound monitoring. Chem Eng J, 433:134625. https://doi.org/10.1016/j.cej.2022.134625
14. Liu SQ, Zheng RM, Chen S, et al., 2018, A compliant, self-adhesive and self-healing wearable hydrogel as epidermal strain sensor. J Mater Chem C, 6(15):4183–4190. https://doi.org/10.1039/c8tc00157j
15. Sun M, Sun X, Wang Z, et al., 2018, Synthesis and properties of gelatin methacryloyl (GelMA) hydrogels and their recent applications in load-bearing tissue. Polymers, 10(11):1290. https://doi.org/10.3390/polym10111290
16. Dou C, Li Z, Luo Y, et al., 2022, Bio-based poly (gamma-glutamic acid)-gelatin double-network hydrogel with high strength for wound healing. Int J Biol Macromol, 202:438–452. https://doi.org/10.1016/j.ijbiomac.2022.01.057
17. Gomez CG, Rinaudo M, Villar MA, 2007, Oxidation of sodium alginate and characterization of the oxidized derivatives. Carbohydr Polym, 67(3):296–304. https://doi.org/10.1016/j.carbpol.2006.05.025
18. Abasalizadeh F, Moghaddam SV, Alizadeh E, et al., 2020, Alginate-based hydrogels as drug delivery vehicles in cancer treatment and their applications in wound dressing and 3D bioprinting. J Biol Eng, 14(1):17. https://doi.org/10.1186/s13036-020-0227-7
19. Shao Y, Wu C, Wu T, et al., 2018, Green synthesis of sodium alginate-silver nanoparticles and their antibacterial activity. Int J Biol Macromol, 111:1281–1292. https://doi.org/10.1016/j.ijbiomac.2018.01.012
20. Chen K, Wang F, Liu S, et al., 2020, In situ reduction of silver nanoparticles by sodium alginate to obtain silver-loaded composite wound dressing with enhanced mechanical and antimicrobial property. Int J Biol Macromol, 148:501–509. https://doi.org/10.1016/j.ijbiomac.2020.01.156
21. Lu B, Han X, Zou D, et al., 2022, Catechol-chitosan/ polyacrylamide hydrogel wound dressing for regulating local inflammation. Mater Today Biol, 16:100392. https://doi.org/10.1016/j.mtbio.2022.100392
22. Zou F, Wang Y, Tang T, et al., 2023, Synergistic strategy constructed hydrogel-aerogel biphase gel (HAB-gel) with self-negative-pressure exudate absorption, M2 macrophage-polarized and antibacterial for chronic wound treatment. Chem Eng J, 451:138952. https://doi.org/10.1016/j.cej.2022.138952
23. Zhu L,Chen L, 2022, Facile design and development of nano-clustery graphene-based macromolecular protein hydrogel loaded with ciprofloxacin to antibacterial improvement for the treatment of burn wound injury. Polym Bull, 79(9): 7953–7968. https://doi.org/10.1007/s00289-021-03875-8
24. Allen R, Waclaw B, 2016, Antibiotic resistance: A physicist’s view. Phys Biol, 13(4):045001. https://doi.org/10.1088/1478-3975/13/4/045001
25. Jiang S, Deng J, Jin Y, et al., 2023, Breathable, antifreezing, mechanically skin-like hydrogel textile wound dressings with dual antibacterial mechanisms. Bioact Mater, 21: 313–323. https://doi.org/10.1016/j.bioactmat.2022.08.014
26. Pang Q, Lou D, Li S, et al., 2020, Smart flexible electronics-integrated wound dressing for real-time monitoring and on-demand treatment of infected wounds. Adv Sci, 7(6):1902673. https://doi.org/10.1002/advs.201902673
27. Jayakumar R, Prabaharan M, Nair SV, et al., 2010, Novel chitin and chitosan nanofibers in biomedical applications. Biotechnol Adv, 28(1):142–150. https://doi.org/10.1016/j.biotechadv.2009.11.001
28. Wang X, Xu P, Yao Z, et al., 2019, Preparation of antimicrobial hyaluronic acid/quaternized chitosan hydrogels for the promotion of seawater-immersion wound healing. Front Bioeng Biotech, 7:360. https://doi.org/10.3389/fbioe.2019.00360
29. Matica MA, Aachmann FL, Tondervik A, et al., 2019, Chitosan as a wound dressing starting material: Antimicrobial properties and mode of action. Int J Mol Sci, 20(23):5889. https://doi.org/10.3390/ijms20235889
30. Liang Y, Zhao X, Hu T, et al., 2019, Mussel-inspired, antibacterial, conductive, antioxidant, injectable composite hydrogel wound dressing to promote the regeneration of infected skin. J Colloid Interface Sci, 556:514–528. https://doi.org/10.1016/j.jcis.2019.08.083
31. Loo AEK, Wong YT, Ho R, et al., 2012, Effects of hydrogen peroxide on wound healing in mice in relation to oxidative damage. PLoS One, 7(11):e49215. https://doi.org/10.1371/journal.pone.0049215
32. Tang P, Han L, Li P, et al., 2019, Mussel-inspired electroactive and antioxidative scaffolds with incorporation of polydopamine-reduced graphene oxide for enhancing skin wound healing. ACS Appl Mater Interfaces, 11(8):7703–7714. https://doi.org/10.1021/acsami.8b18931
33. Ou Q, Zhang S, Fu C, et al., 2021, More natural more better: Triple natural anti-oxidant puerarin/ferulic acid/ polydopamine incorporated hydrogel for wound healing. J Nanobiotechnol, 19(1):237. https://doi.org/10.1186/s12951-021-00973-7
34. Neto AI, Cibrao AC, Correia CR, et al., 2014, Nanostructured polymeric coatings based on chitosan and dopamine-modified hyaluronic acid for biomedical applications. Small, 10(12):2459–2469. https://doi.org/10.1002/smll.201303568
35. Hong S, Yang K, Kang B, et al., 2013, Hyaluronic acid catechol: A biopolymer exhibiting a pH-dependent adhesive or cohesive property for human neural stem cell engineering. Adv Funct Mater, 23(14):1774–1780. https://doi.org/10.1002/adfm.201202365
36. Leppiniemi J, Lahtinen P, Paajanen A, et al., 2017, 3D-printable bioactivated nanocellulose-alginate hydrogels. ACS Appl Mater Interfaces, 9(26):21959–21970. https://doi.org/10.1021/acsami.7b02756
37. Teoh JH, Mozhi A, Sunil V, et al., 2021, 3D printing personalized, photocrosslinkable hydrogel wound dressings for the treatment of thermal burns. Adv Funct Mater, 31(48):2105932. https://doi.org/10.1002/adfm.202105932
38. Guo Y, Huang J, Fang Y, et al., 2022, 1D, 2D, and 3D scaffolds promoting angiogenesis for enhanced wound healing. Chem Eng J, 437:134690. https://doi.org/10.1016/j.cej.2022.134690
39. Chen X, Han S, Wu W, et al., 2022, Harnessing 4D printing bioscaffolds for advanced orthopedics. Small, 18(36):e2106824. https://doi.org/10.1002/smll.202106824
40. Fayyazbakhsh F, Khayat MJ, Leu MC, 2022, 3D-printed gelatin-alginate hydrogel dressings for burn wound healing: A comprehensive study. Int J Bioprint, 8(4):618. https://doi.org/10.18063/ijb.v8i4.618
41. Liu Y, Wong CW, Chang SW, et al., 2021, An injectable, self-healing phenol-functionalized chitosan hydrogel with fast gelling property and visible light-crosslinking capability for 3D printing. Acta Biomater, 122:211–219. https://doi.org/10.1016/j.actbio.2020.12.051
42. Zhong H, Huang J, Wu J, et al., 2021, Electrospinning nanofibers to 1D, 2D, and 3D scaffolds and their biomedical applications. Nano Res, 15(2):787–804. https://doi.org/10.1007/s12274-021-3593-7
43. Wu Z, Hong Y, 2019, Combination of the silver-ethylene interaction and 3D printing to develop antibacterial superporous hydrogels for wound management. ACS Appl Mater Interfaces, 11(37):33734–33747. https://doi.org/10.1021/acsami.9b14090
44. Streifel BC, Lundin JG, Sanders AM, et al., 2018, Hemostatic and absorbent polyHIPE-kaolin composites for 3D printable wound dressing materials. Macromol Biosci, 18(5): 1700414. https://doi.org/10.1002/mabi.201700414
45. Long J, Etxeberria AE, Nand AV, et al., 2019, A 3D printed chitosan-pectin hydrogel wound dressing for lidocaine hydrochloride delivery. Mater Sci Eng C Mater Biol Appl, 104:109873. https://doi.org/10.1016/j.msec.2019.109873
46. Zhao X, Li P, Guo B, et al., 2015, Antibacterial and conductive injectable hydrogels based on quaternized chitosan-graft-polyaniline/oxidized dextran for tissue engineering. Acta Biomater, 26:236–248. https://doi.org/10.1016/j.actbio.2015.08.006
47. Qu J, Zhao X, Liang Y, et al., 2019, Degradable conductive injectable hydrogels as novel antibacterial, anti-oxidant wound dressings for wound healing. Chem Eng J, 362: 548–560. https://doi.org/10.1016/j.cej.2019.01.028
48. Chung Y-C, Chen C-Y, 2008, Antibacterial characteristics and activity of acid-soluble chitosan. Bioresour Technol, 99(8):2806–2814. https://doi.org/10.1016/j.biortech.2007.06.044
49. Wu L, Wu Y, Che X, et al., 2021, Characterization, antioxidant activity, and biocompatibility of selenium nanoparticle-loaded thermosensitive chitosan hydrogels. J Biomater Sci Polym Ed, 32(10):1370–1385. https://doi.org/10.1080/09205063.2021.1917813
50. Xu J, Fang H, Su Y, et al., 2022, A 3D bioprinted decellularized extracellular matrix/gelatin/quaternized chitosan scaffold assembling with poly(ionic liquid) s for skin tissue engineering. Int J Biol Macromol, 220: 1253–1266. https://doi.org/10.1016/j.ijbiomac.2022.08.149
51. Lim SH, Hudson SM, 2004, Synthesis and antimicrobial activity of a water-soluble chitosan derivative with a fiber-reactive group. Carbohydr Res, 339(2):313–319. https://doi.org/10.1016/j.carres.2003.10.024
52. Podstawczyk D, Niziol M, Szymczyk-Ziolkowska P, et al., 2021, Development of thermoinks for 4D direct printing of temperature-induced self-rolling hydrogel actuators. Adv Funct Mater, 31(15):2009664. https://doi.org/10.1002/adfm.202009664
53. Qu J, Zhao X, Liang Y, et al., 2018, Antibacterial adhesive injectable hydrogels with rapid self-healing, extensibility and compressibility as wound dressing for joints skin wound healing. Biomaterials, 183:185–199. https://doi.org/10.1016/j.biomaterials.2018.08.044
54. Zhao X, Guo B, Wu H, et al., 2018, Injectable antibacterial conductive nanocomposite cryogels with rapid shape recovery for noncompressible hemorrhage and wound healing. Nat Commun, 9:2784. https://doi.org/10.1038/s41467-018-04998-9
55. Liu H, Du YM, Wang XH, et al., 2004, Chitosan kills bacteria through cell membrane damage. Int J Food Microbiol, 95(2):147–155. https://doi.org/10.1016/j.ijfoodmicro.2004.01.022
56. Peng Z-X, Wang L, Du L, et al., 2010, Adjustment of the antibacterial activity and biocompatibility of hydroxypropyltrimethyl ammonium chloride chitosan by varying the degree of substitution of quaternary ammonium. Carbohydr Polym, 81(2):275–283. https://doi.org/10.1016/j.carbpol.2010.02.008