3D-Printed scaffolds based on poly(Trimethylene carbonate), poly(ε-Caprolactone), and β-Tricalcium phosphate
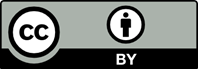
Three-dimensional (3D)-printed scaffolds of biodegradable polymers have been increasingly applied in bone repair and regeneration, which helps avoid the second surgery. PTMC/PCL/TCP composites were made using poly(trimethylene carbonate), poly(ε-caprolactone), and β-tricalcium phosphate. PTMC/PCL/TCP scaffolds were manufactured using a biological 3D printing technique. Furthermore, the properties of PTMC/PCL/TCP scaffolds, such as biodegradation, mechanic properties, drug release, cell cytotoxicity, cell proliferation, and bone repairing capacity, were evaluated. We showed that PTMC/PCL/TCP scaffolds had low cytotoxicity and good biocompatibility, and they also enhanced the proliferation of osteoblast MC3T3-E1 and rBMSC cell lines, which demonstrated improved adhesion, penetration, and proliferation. Moreover, PTMC/PCL/TCP scaffolds can enhance bone induction and regeneration, indicating that they can be used to repair bone defects in vivo.
1. Ligon SC, Liska R, Stampfl J, et al., 2017, Polymers for 3D printing and customized additive manufacturing. Chem Rev, 117: 10212–10290. https://doi.org/10.1021/acs.chemrev.7b00074
2. Matai I, Kaur G, Seyedsalehi A, et al., 2020, Progress in 3D bioprinting technology for tissue/organ regenerative engineering. Biomaterials, 226: 119536. https://doi.org/10.1016/j.biomaterials.2019.119536
3. Yan YF, Chen H, Zhang HB, et al., 2019, Vascularized 3D printed scaffolds for promoting bone regeneration. Biomaterials, 190–1: 97–110. https://doi.org/10.1016/j.biomaterials.2018.10.033
4. Liang Q, Ma Y, Yao X, et al., 2022, Advanced 3D-printing bioinks for articular cartilage repair. Int J Bioprint, 8: 511.
5. Bunpetch V, Zhang XA, Li T, et al., 2019, Silicate-based bioceramic scaffolds for dual-lineage regeneration of osteochondral defect. Biomaterials, 192: 323–333. https://doi.org/10.1016/j.biomaterials.2018.11.025
6. Zhuang P, Sun AX, An J, et al., 2018, 3D Neural tissue models: from spheroids to bioprinting. Biomaterials, 154: 113–133. https://doi.org/10.1016/j.biomaterials.2017.10.002
7. Daly AC, Pitacco P, Nulty J, et al., 2018, 3D printed microchannel networks to direct vascularisation during endochondral bone repair. Biomaterials, 162: 34–46. https://doi.org/10.1016/j.biomaterials.2018.01.057
8. Liu DH, Nie W, Li DJ, et al., 2019, 3D printed PCL/SrHA scaffold for enhanced bone regeneration. Chem Eng J, 362: 269–279.
9. Agudelo RR, Scheuermann K, García AG, et al., 2018, Hybrid nanofibers based on poly-caprolactone/gelatin/hydroxyapatite nanoparticles-loaded doxycycline: effective anti-tumoral and antibacterial activity. Mat Sci Eng C Mater Biol Appl, 83: 25–34. https://doi.org/10.1016/j.msec.2017.08.012
10. Kang HL, Jiang XD, Liu ZW, et al., 2021, Biodegradable 3D printed scaffolds of modified poly (Trimethylene Carbonate) composite materials with poly (L-Lactic Acid) and hydroxyapatite for bone regeneration. Nanomaterials (Basel), 11: 3215. https://doi.org/10.3390/nano11123215
11. Hu B, Du HJ, Yan GP, et al., 2014, Magnetic polycarbonate microspheres for tumor-targeted delivery of tumor necrosis factor. Drug Deliv, 21: 204–212. https://doi.org/10.3109/10717544.2013.843609
12. Wang X, Jiang M, Zhou ZW, et al., 2017, 3D printing of polymer matrix composites: A review and prospective. Composites B, 110: 442–458. https://doi.org/10.1016/j.compositesb.2016.11.034
13. Han SH, Cha MS, Jin YZ, et al., 2021, BMP-2 and hMSC dual delivery onto 3D printed PLA-biogel scaffold for critical-size bone defect regeneration in rabbit tibia. Biomed Mater, 16: 015019. https://doi.org/10.1088/1748-605X/aba879
14. Aihemaiti P, Jiang H, Aiyiti W, et al., 2022, Optimization of 3D printing parameters of biodegradable polylactic acid/hydroxyapatite composite bone plates. Int J Bioprint, 8: 490. https://doi.org/10.18063/ijb.v8i1.490
15. Lu K, Yan GP, Chen H, et al., 2009, Microwave-assisted ring-opening copolymerization of e>-caprolactone and 2-phenyl-5,5-bis(oxymethyl) trimethylene carbonate. Sci Bull, 54: 3237–3243.
16. Feng TJ, Mei LL, Liu F, et al., 2021, Microwave-assisted ring opening copolymerization and property of polycarbonates. Polym Adv Technol, 32: 3412–3420.
17. Liu H, Wu F, Chen R, et al., 2022, Electrohydrodynamic jet-printed ultrathin polycaprolactone scaffolds mimicking bruch’s membrane for retinal pigment epithelial tissue engineering. Int J Bioprint, 8: 550.
18. Xiong X, Xia J, Shaojie Y, et al., 2021, Solvent evaporation induced fabrication of porous polycaprolactone scaffold via low-temperature 3D printing for regeneration medicine researches. Polymer, 217: 123436. https://doi.org/10.1016/j.polymer.2021.123436
19. Guney A, Maldo J, Dhert WJA, et al., 2017, Triblock copolymers based on caprolactone and 1,3-trimethylene carbonate for the 3D printing of tissue engineering scaffolds. Int J Artif Ogran, 40: 176–184. https://doi.org/10.5301/ijao.5000543
20. UIIah I, Cao L, Cui W, et al., 2021, Stereolithography printing of bone scaffolds using biofunctional calcium phosphate nanoparticles. J Mater Sci Technol, 88: 99–108.
21. Chen L, Deng CJ, Li JY, et al., 2019, 3D printing of a lithiumcalcium-silicate crystal bioscaffold with dual bioactivities for osteochondral interface reconstruction. Biomaterials, 196: 138–150. https://doi.org/10.1016/j.biomaterials.2018.04.005
22. Ma HS, Feng C, Chang J, et al., 2018, 3D-printed bioceramic scaffolds: from bone tissue engineering to tumor therapy. Acta Biomater, 79: 37–59. https://doi.org/10.1016/j.actbio.2018.08.026
23. Li DW, Zhang K, Shi C, et al., 2018, Small molecules modified biomimetic gelatin/hydroxyapatite nanofibers constructing an ideal osteogenic microenvironment with significantly enhanced cranial bone formation. Int J Nanomedicine, 13: 7167–7181. https://doi.org/10.2147/IJN.S174553
24. Marc B, Le Gars SB, Nicola D, 2020, β-Tricalcium phosphate for bone substitution: Synthesis and properties. Acta Biomater, 113: 23–41. https://doi.org/10.1016/j.actbio.2020.06.022
25. Jang J, Park JY, Gao G, et al., 2018, Biomaterials-based 3D cell printing for next-generation therapeutics and diagnostics. Biomaterials, 156: 88–106. https://doi.org/10.1016/j.biomaterials.2017.11.030
26. Corcione CE, Gervaso F, Scalera F, et al., 2019, Highly loaded hydroxyapatite microsphere/pla porous scaffolds obtained by fused deposition modeling. Ceram Int, 45: 2803–2810.
27. Chen H, Yan GP, Li L, et al., 2009, Synthesis, characterization and properties of ε-caprolactone and carbonate copolymers. J Appl Polym Sci, 114: 3087–3096.
28. Liu F, Mei LL, Tan ZL, et al., 2016, Studies on microwave assisted ring-opening polymerization and property of poly (9-phenyl-2,4,8,10-tetraoxaspiro-[5,5]undcane-3-one). Chin. J Polym Sci, 34: 1330–1338.
29. Shi FQ, 1990, How to copy the disease model of animals. In: medical animal experiment method. Ch. 4. Beijing, china: people’s medical publishing house, p226–232.
30. Liu F, Kang HL, Liu ZW, et al., 2021, 3D printed multifunctional scaffolds based on poly(ε-caprolactone) and hydroxyapatite composites. Nanomaterials (Basel), 11: 2456. https://doi.org/10.3390/nano11092456
31. Liu YQ, Li T, Ma HS, et al., 2018, 3D-printed scaffolds with bioactive elements-induced photothermal effect for bone tumor therapy. Acta Biomater, 73: 531–46. https://doi.org/10.1016/j.actbio.2018.04.014
32. Morgan EF, Unnikrisnan GU, Hussein AI, 2018, Bone mechanical properties in healthy and diseased states. Annu Rev Biomed Eng, 20: 119–143. https://doi.org/10.1146/annurev-bioeng-062117-121139
33. Roberts CR, Rains JK, Paré PD, et al., 1997, Ultrastructure and tensile properties of human tracheal cartilage. J Biomech, 31: 81–6. https://doi.org/10.1016/s0021-9290(97)00112-7