Robotic-assisted automated in situ bioprinting
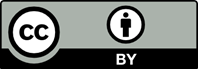
In situ bioprinting has emerged as a promising technology for tissue and organ engineering based on the precise positioning of living cells, growth factors, and biomaterials. Rather than traditional in vitro reconstruction and recapitulation of tissue or organ models, the in situ technology can directly print on specific anatomical positions in living bodies. The requirements for biological activity, function, and mechanical property in an in vivo setting are more complex. By combining progressive innovations of biomaterials, tissue engineering, and digitalization, especially robotics, in situ bioprinting has gained significant interest from the academia and industry, demonstrating its prospect for clinical studies. This article reviews the progress of in situ bioprinting, with an emphasis on robotic-assisted studies. The main modalities for in situ three-dimensional bioprinting, which include extrusion-based printing, inkjet printing, laser-based printing, and their derivatives, are briefly introduced. These modalities have been integrated with various custom-tailored printers (i.e., end effectors) mounted on robotic arms for dexterous and precision biofabrication. The typical prototypes based on various robot configurations, including Cartesian, articulated, and parallel mechanisms, for in situ bioprinting are discussed and compared. The conventional and most recent applications of robotic-assisted methods for in situ fabrication of tissue and organ models, including cartilage, bone, and skin, are also elucidated, followed by a discussion on the existing challenges in this field with their corresponding suggestions.
1. Gao W, Zhang Y, Ramanujan D, et al., 2015, The status, challenges, and future of additive manufacturing in engineering. Comput Aided Des, 69: 65–89. https://doi.org/10.1016/j.cad.2015.04.001
2. Kruth JP, Leu MC, Nakagawa T, 1998, Progress in additive manufacturing and rapid prototyping. Cirp Ann, 47: 25–540. https://doi.org/10.1016/S0007-8506(07)63240-5
3. Dilberoglu UM, Gharehpapagh B, Yaman U, et al., 2017, The role of additive manufacturing in the era of industry 4.0. Proc Manuf, 11: 545–554. https://doi.org/10.1016/j.promfg.2017.07.148
4. Murphy SV, Atala A, 2014, 3D bioprinting of tissues and organs. Nat Biotechnol, 32: 773–785. https://doi.org/10.1038/nbt.2958
5. Ma X, Liu J, Zhu W, et al., 2018, 3D bioprinting of functional tissue models for personalized drug screening and in vitro disease modeling. Adv Drug Deliv Rev, 132: 235–251. https://doi.org/10.1016/j.-addr.2018.06.011
6. Pati F, Gantelius J, Svahn HA, 2016, 3D bioprinting of tissue/ organ models. Angew Chem Int Ed Engl, 55: 4650–4665. https://doi.org/10.1002/anie.201505062
7. Peng W, Datta P, Ayan B, et al., 2017, 3D bioprinting for drug discovery and development in pharmaceutics. Acta Biomater, 57: 26–46. https://doi.org/10.1016/j.actbio.2017.05.025
8. Di Marzio N, Eglin D, Serra T, et al., 2020, Bio-fabrication: convergence of 3D bioprinting and nano-biomaterials in tissue engineering and regenerative medicine. Front Bioeng Biotechnol, 8: 326. https://doi.org/10.3389/fbioe.2020.00326
9. Murphy SV, De Coppi P, Atala A, 2020, Opportunities and challenges of translational 3D bioprinting. Nat Biomed Eng, 4: 370–380. https://doi.org/10.1038/s41551-019-0471-7
10. Sun W, Starly B, Daly AC, et al., 2020, The bioprinting roadmap. Biofabrication, 12: 022002. https://doi.org/10.1088/1758-5090/ab5158
11. Samandari M, Mostafavi A, Quint J, et al., 2022, In situ bioprinting: Intraoperative implementation of regenerative medicine. Trends Biotechnol, 40: 1229–1247. https://doi.org/10.1016/j.tibtech.2022.03.009
12. Campbell PG, Weiss LE, 2007, Tissue engineering with the aid of inkjet printers. Expert Opin Biol Ther, 7: 1123–1127. https://doi.org/10.1517/14712598.7.8.1123
13. Hong N, Yang GH, Lee J H, et al., 2018, 3D bioprinting and its in vivo applications. J Biomed Mater Res B Appl Biomater, 106: 444–459. https://doi.org/10.1002/-jbm.b.33826
14. Prendergast ME, Burdick JA, 2020, Recent advances in enabling technologies in 3D printing for precision medicine. Adv Mater, 32: 1902516. https://doi.org/10.1002/adma.201902516
15. Singh S, Choudhury D, Yu F, et al., 2020, In situ bioprinting-bioprinting from benchside to bedside? Acta Biomater, 101: 14–25. https://doi.org/10.1016/j.actbio.2019.08.045
16. Wang M, He J, Liu Y, et al., 2015, The trend towards in vivo bioprinting. Int J Bioprint, 1: 15–26. https://doi.org/10.18063/IJB.2015.01.001
17. Ozbolat IT, 2015, Bioprinting scale-up tissue and organ constructs for transplantation. Trends Biotechnol, 33: 395–400. https://doi.org/10.1016/j.tibtech.2015.04.005
18. Ding H, Chang RC, 2018, Simulating image-guided in situ bioprinting of a skin graft onto a phantom burn wound bed. Addit Manuf, 22: 708–719. https://doi.org/10.1016/j.addma.2018.06.022
19. Samandari M, Quint J, Rodríguez‐delaRosa A, et al., 2022, Bioinks and bioprinting strategies for skeletal muscle tissue engineering. Adv Mater, 34: 2105883. https://doi.org/10.1002/adma.202105883
20. Wu Y, Ravnic DJ, Ozbolat IT, 2020, Intraoperative bioprinting: Repairing tissues and organs in a surgical setting. Trends Biotechnol, 38: 594–605. https://doi.org/10.1016/j.tibtech.2020.01.004
21. Ozbolat IT, Hospodiuk M, 2016, Current advances and future perspectives in extrusion-based bioprinting. Biomaterials, 76: 321–343. https://doi.org/10.1016/j.biomaterials.2015.10.076
22. Sun H, Jia Y, Dong H, et al., 2020, Combining additive manufacturing with microfluidics: An emerging method for developing novel organs-on-chips. Curr Opin Chem Eng, 28: 1–9. https://doi.org/10.1016/j.coche.2019.10.006
23. Dou C, Perez V, Qu J, et al., 2021, A state‐of‐the‐art review of laser‐assisted bioprinting and its future research trends. Chem Bio Eng Rev, 8: 517–534. https://doi.org/10.1002/cben.202000037
24. Jentsch S, Nasehi R, Kuckelkorn C, et al., 2021, Multiscale 3D bioprinting by nozzle‐free acoustic droplet ejection. Small Methods, 5: 2000971. https://doi.org/10.1002/smtd.202000971
25. Masaeli E, Marquette C, 2020, Direct-write bioprinting approach to construct multilayer cellular tissues. Front Bioeng Biotechnol, 7: 478. https://doi.org/10.3389/fbioe.2019.00478
26. Darwish LR, El-Wakad MT, Farag MM, 2021, Towards an ultra-affordable three-dimensional bioprinter: A heated inductive-enabled syringe pump extrusion multifunction module for open-source fused deposition modeling three- dimensional printers. J Manuf Sci Eng, 143: 125001. https://doi.org/10.1115/1.4050824
27. Salehi MM, Ataeefard M, 2019, Micro powder poly lactic acid/carbon black composite as a bio printing ink. J Composite Mater, 53: 2407–2414. https://doi.org/10.1177/0021998319828154
28. Kalan S, Chauhan S, Coelho RF, et al., 2010, History of robotic surgery. J Robot Surg, 4: 141–147. https://doi.org/10.1007/s11701-010-0202-2
29. Simeunović A, Wolf K, Tierling K, et al., 2022, A surgical robot for intracorporeal additive manufacturing of tissue engineering constructs. IEEE Robot Automation Lett, 7: 7495–7502. https://doi.org/10.1109/LRA.2022.3183752
30. Tan B, Kuang S, Li X, et al., 2021, Stereotactic technology for 3D bioprinting: From the perspective of robot mechanism. Biofabrication, 13: 043001. https://doi.org/10.1088/1758-5090/ac1846
31. Shi E, Lou L, Warburton L, et al., 2022, 3D printing in combined Cartesian and curvilinear coordinates. J Med Devices, 16: 044502. https://doi.org/10.1115/1.4055064
32. Urhal P, Weightman A, Diver C, et al., 2019, Robot assisted additive manufacturing: A review. Robot Comput Integr Manuf, 59: 335–345. https://doi.org/10.1016/j.rcim.2019.05.005
33. O’Neill JJ, Johnson RA, Dockter RL, et al., 2017, 3D bioprinting directly onto moving human anatomy. In: 2017 IEEE/RSJ International Conference on Intelligent Robots and Systems (IROS). New Jersey: IEEE. p934–940. https://doi.org/10.1109/IROS.2017.8202257
34. Fortunato GM, Rossi G, Bonatti AF, et al., 2021, Robotic platform and path planning algorithm for in situ bioprinting. Bioprinting, 22: e00139. https://doi.org/10.1016/j.bprint.2021.e00139
35. Dong H, Du Z, Chirikjian GS, 2013, Workspace density and inverse kinematics for planar serial revolute manipulators. Mech Machine Theory, 70: 508–522. https://doi.org/10.1016/j.mechmachtheory.2013.08.008
36. Hanly EJ, Talamini MA, 2004, Robotic abdominal surgery. Am J Surg, 188: 19–26. https://doi.org/10.1016/j.amjsurg.2004.08.020
37. D’Souza M, Gendreau J, Feng A, et al., 2019, Robotic-assisted spine surgery: History, efficacy, cost, and future trends. Robot Surg Res Rev, 6: 9–23. https://doi.org/10.2147/RSRR.S190720
38. Torabi A, Khadem M, Zareinia K, et al., 2020, Using a redundant user interface in teleoperated surgical systems for task performance enhancement. Robotica, 38: 1880–1894. https://doi.org/10.1017/-S0263574720000326
39. Zhao Q, Guo J, Hong J, et al., 2021, An enhanced moment-based approach to time-dependent positional reliability analysis for robotic manipulators. Mech Machine Theory, 156: 104167. https://doi.org/10.1016/j.mechmachtheory.2020.104167
40. Li L, Shi J, Ma K, et al., 2021, Robotic in situ 3D bio-printing technology for repairing large segmental bone defects. J Adv Res, 30: 75–84. https://doi.org/10.1016/j.jare.2020.11.011
41. Zhao W, Chen H, Zhang Y, et al., 2022, Adaptive multi‐degree‐of‐freedom in situ bioprinting robot for hair‐follicle‐inclusive skin repair: A preliminary study conducted in mice. Bioeng Transl Med, 2022: e10303. https://doi.org/10.1002/btm2.10303
42. Zhang Z, Wu C, Dai C, et al., 2022, A multi-axis robot-based bioprinting system supporting natural cell function preservation and cardiac tissue fabrication. Bioactive Mater, 18: 138–150. https://doi.org/10.1016/j.bioactmat.2022.02.009
43. Ding J, Lyu S, Da T, et al., 2019, Error space estimation of three degrees of freedom planar parallel mechanisms. J Mech Robot, 11: 031013. https://doi.org/10.1115/1.4042633
44. Yang Y, Peng Y, Pu H, et al., 2019, Deployable parallel lower-mobility manipulators with scissor-like elements. Mech Machine Theory, 135: 226–250. https://doi.org/10.1016/j.mechmachtheory.2019.01.013
45. Zhu Z, Guo S Z, Hirdler T, et al., 2018, 3D printed functional and biological materials on moving freeform surfaces. Adv Mater, 30: 1707495. https://doi.org/10.1002/adma.201707495
46. Zhao W, Xu T, 2020, Preliminary engineering for in situ in vivo bioprinting: A novel micro bioprinting platform for in situ in vivo bioprinting at a gastric wound site. Biofabrication, 12: 045020. https://doi.org/10.1088/1758-5090/aba4ff
47. Cui X, Breitenkamp K, Finn MG, et al., 2012, Direct human cartilage repair using three-dimensional bioprinting technology. Tissue Eng Part A, 18: 1304–1312. https://doi.org/10.1089/ten.tea.-2011.0543
48. Sun Y, You Y, Jiang W, et al., 2020, 3D bioprinting dual-factor releasing and gradient-structured constructs ready to implant for anisotropic cartilage regeneration. Sci Adv, 6: eaay1422. https://doi.org/-10.1126/sciadv.aay1422
49. Ma K, Zhao T, Yang L, et al., 2020, Application of robotic-assisted in situ 3D printing in cartilage regeneration with HAMA hydrogel: An in vivo study. J Adv Res, 23: 123–132. https://doi.org/10.1016/j.jare.2020.01.010
50. Keriquel V, Oliveira H, Rémy M, et al., 2017, In situ printing of mesenchymal stromal cells, by laser-assisted bioprinting, for in vivo bone regeneration applications. Sci Rep, 7: 1–10. https://doi.org/10.1038/s41598-017-01914-x
51. Lipskas J, Deep K, Yao W, 2019, Robotic-assisted 3D bio-printing for repairing bone and cartilage defects through a minimally invasive approach. Sci Rep, 9: 1–9. https://doi.org/10.1038/s41598-019-38972-2
52. Lee V, Singh G, Trasatti JP, et al., 2014, Design and fabrication of human skin by three-dimensional bioprinting. Tissue Eng Part C Methods, 20: 473–484. https://doi.org/10.1089/ten.tec.2013.0335
53. Cubo N, Garcia M, Del Canizo JF, et al., 2016, 3D bioprinting of functional human skin: Production and in vivo analysis. Biofabrication, 9: 015006. https://doi.org/10.1088/1758-5090/9/1/015006
54. Albanna M, Binder KW, Murphy SV, et al., 2019, In situ bioprinting of autologous skin cells accelerates wound healing of extensive excisional full-thickness wounds. Sci Rep, 9: 1586. https://doi.org/10.1038/s41598-018-38366-w
55. Chen Y, Zhang J, Liu X, et al., 2020, Noninvasive in vivo 3D bioprinting. Sci Adv, 6: eaba7406. https://doi.org/10.1126/sciadv.aba7406
56. Lee VK, Lanzi AM, Ngo H, et al., 2014, Generation of multi-scale vascular network system within 3D hydrogel using 3D bio-printing technology. Cell Mol Bioeng, 7: 460–472. https://doi.org/10.1007/s12195-014-0340-0
57. Owens CM, Marga F, Forgacs G, et al., 2013, Biofabrication and testing of a fully cellular nerve graft. Biofabrication, 5: 045007. https://doi.org/10.1088/1758-5082/5/4/045007
58. Zhou C, Yang Y, Wang J, et al., 2021, Ferromagnetic soft catheter robots for minimally invasive bioprinting. Nat Commun, 12: 5072. https://doi.org/10.1038/s41467-021-25386-w