Effect of ionic crosslinking on morphology and thermostability of biomimetic supercritical fluids-decellularized dermal-based composite bioscaffolds for bioprinting applications
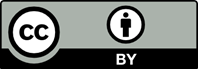
In the present study, supercritical fluid was employed to prepare a kind of supercritical fluids-decellularized dermal-based scaffold (SFDDS) from porcine dermal tissue. Further, new composite bioscaffolds containing SFDDS were designed for bioprinting applications. Then, the effect of crosslinking functionality on microstructures and thermal properties of the composite bioscaffolds containing decellularized extracellular matrix were studied. The results of thermal stability from thermogravimetric analysis and difference thermogravimetry demonstrated the structural stability of the composite bioscaffolds. A method was designed to prepare bioinspired decellularized dermal-based composite bioscaffolds, which were further characterized by infrared spectroscopy, scanning electron microscopy, and thermogravimetry analysis.
1. Huang CC, Chen YJ, Liu HW, 2021, Characterization of composite nano-bioscaffolds based on collagen and supercritical fluids-assisted decellularized fibrous extracellular matrix. Polymers, 13: 4326. https://doi.org/10.3390/polym13244326
2. Tappa K, Jammalamadaka U, 2018, Novel biomaterials used in medical 3d printing techniques. J Funct Biomater, 9: 17. https://doi.org/10.3390/jfb9010017
3. Rhee S, Puetzer JL, Mason BN, et al., 2016, 3D bioprinting of spatially heterogeneous collagen constructs for cartilage tissue engineering. ACS Biomater Sci Eng, 2: 1800–1805. https://doi.org/10.1021/acsbiomaterials.6b00288
4. Laronda MM, Rutz AL, Xiao S, et al., 2017, A bioprosthetic ovary created using 3D printed microporous scaffolds restores ovarian function in sterilized mice. Nat Commun, 8: 15261. https://doi.org/10.1038/ncomms15261
5. Markstedt K, Mantas A, Tournier I, et al., 2015, 3D bioprinting human chondrocytes with nanocellulose alginate bioink for cartilage tissue engineering applications. Biomacromolecules, 16: 1489–1496. https://doi.org/10.1021/acs.biomac.5b00188
6. Nguyen D, Hägg DA, Forsman A, et al., 2017, Cartilage tissue engineering by the 3d bioprinting of ips cells in a nanocellulose/alginate bioink. Sci Rep, 7: 658.
7. Tan Z, Parisi C, Di Silvio L, et al., 2017, Cryogenic 3D printing of super soft hydrogels. Sci Rep, 7: 16293. https://doi.org/10.1038/s41598-017-16668-9
8. Gao Q, Kim BS, Gao G, 2021, Advanced strategies for 3D bioprinting of tissue and organ analogs using alginate hydrogel bioinks. Mar Drugs, 19: 708. https://doi.org/10.3390/md19120708
9. Chen YW, Hsieh DJ, Periasamy S, 2021, Development of a decellularized porcine bone graft by supercritical carbon dioxide extraction technology for bone regeneration. J Tissue Eng Regen Med, 15: 401–404. https://doi.org/10.1002/term.3181
10. Seo Y, Jung Y, Kim SH, 2018, Decellularized heart ecm hydrogel using supercritical carbon dioxide for improved angiogenesis. Acta Biomater, 67: 270–281. https://doi.org/10.1016/j.actbio.2017.11.046
11. Ma JZ, Hou XY, Gao DG, 2014, Greener approach to efficient leather soaking process: role of enzymes and their synergistic effect. J Clean Prod, 1: 226–232.
12. Kim BS, Kim JU, So KH, et al., 2021, Supercritical fluid-based decellularization technologies for regenerative medicine applications, Macromol Biosc, 21: e2100160. https://doi.org/10.1002/mabi.202100160
13. Huang CC, Liu CY, Huang CY, et al., 2014, Carbodiimide cross-linked and biodegradation-controllable small intestinal submucosa sheets. Biomed Mater Eng, 24: 1959– 1967. https://doi.org/10.3233/BME-141005
14. Liu YW, Huang CC, Wang YY, et al., 2021, Biological evaluations of decellularized extracellular matrix collagen microparticles prepared based on plant enzymes and aqueous two-phase method. Regen Biomater, 8: rbab002. https://doi.org/10.1093/rb/rbab002
15. Huang CC, 2022, Newly designed decellularized scaffolds for scaffold-based gene therapy from elastic cartilages via supercritical carbon dioxide fluid and alkaline/protease treatments. Curr Gene Ther, 22: 162–167. https://doi.org/10.2174/1566523219666210618151843
16. Chou PR, Lin YN, Wu SH, et al., 2020, Supercritical carbon dioxide-decellularized porcine acellular dermal matrix combined with autologous adipose-derived stem cells: its role in accelerated diabetic wound healing. Int J Med Sci, 17: 354–367. https://doi.org/10.7150/ijms.41155
17. Li Y, Xu Y, Liu Y, et al., 2019, Decellularized cartilage matrix scaffolds with laser-machined micropores for cartilage regeneration and articular cartilage repair. Mater Sci Eng C Mater Biol Appl, 105: 110139. https://doi.org/10.1016/j.msec.2019.110139
18. Wang D, Zhu Y, Huang Y, et al., 2021, Pancreatic extracellular matrix/alginate hydrogels provide a supportive microenvironment for insulin-producing cells. ACS Biomater Sci Eng, 7: 3793–3805. https://doi.org/10.1021/acsbiomaterials.1c00269
19. Hwang TI, Moon JY, Kim JI, et al., 2020, Fabrication of three-dimensional alginate porous scaffold incorporated with decellularized cornu cervi pantotrichum particle for bone tissue engineering. J Nanosci Nanotechnol, 20: 5356– 5359. https://doi.org/10.1166/jnn.2020.17676
20. Luo B, Loh QL, Chong MT, et al., 2014, Bioactivated protein-based porous microcarriers for tissue engineering applications. J Mater Chem B, 2: 7795–7803. https://doi.org/10.1039/C4TB00846D
21. Zhang YS, Haghiashtiani G, Hübscher T, et al., 2021, 3D extrusion bioprinting. Nat Rev Methods Primers, 1: 75.
22. Lee JM, Yeong WY, 2020, Engineering macroscale cell alignment through coordinated toolpath design using support-assisted 3D bioprinting. J R Soc Interface, 17: 20200294. https://doi.org/10.1098/rsif.2020.0294
23. Wang JK, Luo B, Guneta V, et al., 2017, Supercritical carbon dioxide extracted extracellular matrix material from adipose tissue. Mat Sci Eng C Mater Biol Appl, 75: 349–358. https://doi.org/10.1016/j.msec.2017.02.002
24. Sun D, Liu Y, Wang H, 2018, Novel decellularized liver matrix-alginate hybrid gel beads for the 3D culture of hepatocellular carcinoma cells. Int J Biol Macromol, 109: 1154–1163. https://doi.org/10.1016/j.ijbiomac.2017.11.103
25. Adochitei A, Drochioiu G, 2011, Rapid characterization of peptide secondary structure by ftir spectroscopy. Rev Roum Chim, 56: 783–791.
26. Chen K, Li J, Zhao W, et al., 2022, Physicochemical properties of collagen from the bone of harpadon nehereus and its protective effects against angiotensin ii-induced injury in human umbilical vein endothelial cells. ACS Omega, 7: 23412–23420. https://doi.org/10.1021/acsomega.2c01739
27. Zhao WH, Chi CF, Zhao YQ, et al., 2018, Preparation, physicochemical and antioxidant properties of acid- and pepsin-soluble collagens from the swim bladders of miiuy croaker (Miichthys miiuy). Mar Drugs, 16: 161. https://doi.org/10.3390/md16050161
28. Nagai T, Izumi M, Ishii M, 2004, Fish scale collagen. Preparation and partial characterization. Int J Food Sci Technol, 39: 239–244. https://doi.org/10.1111/j.1365-2621.2004.00777.x
29. Naghieh S, Karamooz-Ravari MR, Sarker MD, et al., 2018, Influence of crosslinking on the mechanical behavior of 3D printed alginate scaffolds: experimental and numerical approaches. J Mech Behav Biomed Mater, 80: 111–118. https://doi.org/10.1016/j.jmbbm.2018.01.034