Perfusable Vessel-on-a-Chip for Antiangiogenic Drug Screening with Coaxial Bioprinting
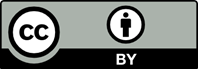
Vessel-on-a-chips, which can be used to study microscale fluid dynamics, tissue-level biological molecules delivery and intercellular communication under favorable three-dimensional (3D) extracellular matrix microenvironment, are increasingly gaining traction. However, not many of them can allow for long-term perfusion and easy observation of angiogenesis process. Since angiogenesis is necessary for the expansion of tumor, antiangiogenic drugs play a significant role in cancer treatment. In this study, we established an innovative and reliable antiangiogenic drug screening chip that was highly modularly integrated for long-term perfusion (up to 10 days depending on the hydrogel formula) and real-time monitoring. To maintain an unobstructed flow of cell-laden tubes for subsequent perfusion culture on the premise of excellent bioactivities, a polycaprolactone stent inspired by coronary artery stents was introduced to hold up the tubular lumen from the inside, while the perfusion chip was also elaborately designed to allow for convenient observation. After 3 days of perfusion screening, distinct differences in human umbilical vein endothelial cell sprouting were observed for a gradient of concentrations of bevacizumab, which pointed to the effectiveness and reliability of the drug screening perfusion system. Overall, a perfusion system for antiangiogenic drug screening was developed, which can not only conduct drug evaluation, but also be potentially useful in other vessel-mimicking scenarios in the area of tissue engineering, drug screening, pharmacokinetics, and regenerative medicine.
1. Cherrington JM, Strawn LM, Shawver LK, 2000, New Paradigms for the Treatment of Cancer: The Role of Anti-Angiogenesis Agents. Adv Cancer Res, 79:1–38. https://doi.org/10.1016/s0065-230x(00)79001-4
2. Zou L, Lai H, Zhou Q, et al., 2011, Lasting Controversy on Ranibizumab and Bevacizumab. Theranostics, 1:395–402. https://doi.org/10.7150/thno/v01p0395
3. Hurwitz H, Fehrenbacher L, Novotny W, et al., 2004 Bevacizumab Plus Irinotecan, Fluorouracil, and Leucovorin for Metastatic Colorectal Cancer. N Engl J Med, 350:2335–42. https://doi.org/10.1056/NEJMoa032691
4. Santoro MM, 2014, Antiangiogenic Cancer Drug using the Zebrafish Model. Atertisclero Thromb Vasc Biol, 34:1846–53. https://doi.org/10.1161/ATVBAHA.114.303221
5. Beedie SL, Diamond AJ, Fraga LR, et al., 2017, Vertebrate Embryos as Tools for Anti-angiogenic Drug Screening and Function. Reprod Toxicol, 70:49–59. https://doi.org/10.1016/j.reprotox.2016.11.013
6. Kim S, Ko J, Lee SR, et al., 2021, Anchor-IMPACT: A Standardized Microfluidic Platform for High-throughput Antiangiogenic Drug Screening. Biotechnol Bioeng, 118:2524–35.
7. Van Duinen V, Stam W, Mulder E, et al., 2020, Robust and Scalable Angiogenesis Assay of Perfused 3D Human iPSCderived Endothelium for Anti-angiogenic Drug Screening. Int J Mol Sci, 21:4804. https://doi.org/10.3390/ijms21134804
8. Nie J, Gao Q, Wang Y, et al., 2018, Vessel-on-a-chip with Hydrogel-based Microfluidics. Small, 14:e1802368. https://doi.org/10.1002/smll.201802368
9. Huh D, Matthews BD, Mammoto A, et al., 2010, Reconstituting Organ-level Lung Functions on a Chip. Science, 328:1662–8. https://doi.org/10.1126/science.1188302
10. Zhang B, Korolj A, Lai BF, et al., 2018, Advances in Organon-a-chip Engineering. Nat Rev Mater, 3:257–78.
11. Pampaloni F, Reynaud EG, Stelzer EH, 2007, The Third Dimension Bridges the Gap between Cell Culture and Live Tissue. Nat Rev Mol Cell Biol, 8:839–45. https://doi.org/10.1038/nrm2236
12. Sun W, Luo Z, Lee J, et al., 2019, Organ-on-a-chip for Cancer and Immune Organs Modeling. Adv Healthc Mater, 8:1801363. https://doi.org/10.1002/adhm.201801363
13. Huang D, Liu T, Liao J, et al., 2021, Reversed-Engineered Human Alveolar Lung-on-a-chip Model. Proc Nat Acad Sci U S A, 118:e2016146118. https://doi.org/10.1073/pnas.2016146118
14. Yang Q, Xiao Z, Lv X, et al., 2021, Fabrication and Biomedical Applications of Heart-on-a-chip. Int J Bioprint, 7:370. https://doi.org/10.18063/ijb.v7i3.370
15. Knowlton S, Tasoglu S, 2016, A Bioprinted Liver-on-a-chip for Drug Screening Applications. Trends Biotechnol, 34:681–2. https://doi.org/10.1016/j.tibtech.2016.05.014
16. Phan DT, Wang X, Craver BM, et al., 2017, A Vascularized and Perfused Organ-on-a-chip Platform for Large-scale Drug Screening Applications. Lab Chip, 17:511–20.
17. Chramiec A, Teles D, Yeager K, et al., 2020, Integrated Human Organ-on-a-chip Model for Predictive Studies of Anti-tumor Drug Efficacy and Cardiac Safety. Lab Chip, 20:4357–72. https://doi.org/10.1039/d0lc00424c
18. Yang Q, Lian Q, Xu F, 2017, Perspective: Fabrication of Integrated Organ-on-a-chip Via Bioprinting. Biomicrofluidics, 11:031301. https://doi.org/10.1063/1.4982945
19. Lee GY, Kenny PA, Lee EH, et al., 2007, Three-dimensional Culture Models of Normal and Malignant Breast Epithelial Cells. Nat Methods, 4:359–65. https://doi.org/10.1038/nmeth1015
20. Wang L, Tao T, Su W, et al., 2017, A Disease Model of Diabetic Nephropathy in a Glomerulus-on-a-chip Microdevice. Lab Chip, 17:1749–60. https://doi.org/10.1039/c7lc00134g
21. Sharifi F, Yesil-Celiktas O, Kazan A, et al., 2020, A Hepatocellular Carcinoma Bone Metastasis-on-a-chip Model for Studying Thymoquinone-loaded Anticancer Nanoparticles. Biodes Manuf, 3:189–202.
22. Liu Y, Yang Q, Zhang H, et al., 2021, Construction of Canceron-a-chip for Drug Screening. Drug Discov Today, 26:1875–90. https://doi.org/10.1016/j.drudis.2021.03.006
23. Tavakol DN, Fleischer S, Vunjak-Novakovic G, 2021, Harnessing Organs-on-a-chip to Model Tissue Regeneration. Cell Stem Cell, 28:993–1015. https://doi.org/10.1016/j.stem.2021.05.008
24. Zhao Y, Rafatian N, Feric NT, et al., 2019, A Platform for Generation of Chamber-specific Cardiac Tissues and Disease Modeling. Cell, 176:913–27.e18. https://doi.org/10.1016/j.cell.2018.11.042
25. Zubizarreta ME, Xiao S, 2020, Bioengineering Models of Female Reproduction. Bi Des Manuf, 3:237–51. https://doi.org/10.1007/s42242-020-00082-8
26. Caballero D, Blackburn SM, De Pablo M, et al., 2017, Tumour-vessel-on-a-chip Models for Drug Delivery. Lab Chip, 17:3760–71.
27. Gu Z, Fu J, Lin H, et al., 2020, Development of 3D Bioprinting: From Printing Methods to Biomedical Applications. Asian J Pharm Sci, 15:529–57.
28. He Y, Xie M, Gao Q, et al., 2019, Why Choose 3D Bioprinting? Part I: A Brief Introduction of 3D Bioprinting for the Beginners. Biodes Manuf, 3:1–4.
29. Yang Q, Lv X, Gao B, et al, 2021, Mechanics of Hydrogel-based Bioprinting: From 3D to 4D. Adv Appl Mech, 54:285–318.
30. Zhang Y, Duchamp M, Oklu R, et al., 2016, Bioprinting the Cancer Microenvironment. ACS Biomater Sci Eng, 2:1710–21.
31. Kinstlinger IS, Miller JS, 2016, 3D-printed Fluidic Networks as Vasculature for Engineered Tissue. Lab Chip, 16:2025–43.
32. Gao Q, He Y, Fu JZ, et al., 2015, Coaxial Nozzle-assisted 3D Bioprinting with Built-in Microchannels for Nutrients Delivery. Biomaterials, 61:203–15. https://doi.org/10.1016/j.biomaterials.2015.05.031
33. Gao Q, Liu Z, Lin Z, et al., 2017, 3D Bioprinting of Vessel like Structures with Multilevel Fluidic Channels. ACS Biomater Sci Eng, 3:399–408. https://doi.org/10.1021/acsbiomaterials.6b00643
34. Ouyang L, Highley CB, Sun W, et al., 2017, A Generalizable Strategy for the 3D Bioprinting of Hydrogels from Nonviscous Photo-crosslinkable Inks. Adv Mater, 29: 1604983. https://doi.org/10.1002/adma.201604983
35. Jia W, Gungor-Ozkerim PS, Zhang YS, et al., 2016, Direct 3D Bioprinting of Perfusable Vascular Constructs Using a Blend Bioink. Biomaterials, 106:58–68. https://doi.org/10.1016/j.biomaterials.2016.07.038
36. Gao G, Lee JH, Jang J, et al., 2017, Tissue Engineered Bioblood-vessels Constructed Using a Tissue-specific Bioink and 3D Coaxial Cell Printing Technique: A Novel Therapy for Ischemic Disease. Adv Funct Mater, 27:1700798. https://doi.org/10.1002/adfm.201700798
37. Shao L, Gao Q, Zhao H, et al., 2018, Fiber-based Mini Tissue with Morphology-controllable GelMA Microfibers. Small, 14:e1802187. https://doi.org/10.1002/smll.201802187
38. Shao L, Gao Q, Xie C, et al., 2020, Directly Coaxial 3D Bioprinting of Large-scale Vascularized Tissue Constructs. Biofabrication, 12:035014. https://doi.org/10.1088/1758-5090/ab7e76
39. Lee VK, Kim DY, Ngo H, et al., 2014, Creating Perfused Functional Vascular Channels Using 3D Bio-printing Technology. Biomaterials, 35:8092–102. https://doi.org/10.1016/j.biomaterials.2014.05.083
40. Gao G, Park JY, Kim BS, et al., 2018, Coaxial Cell Printing of Freestanding, Perfusable, and Functional in vitro Vascular Models for Recapitulation of Native Vascular Endothelium Pathophysiology. Adv Healthc Mater, 7:e1801102. https://doi.org/10.1002/adhm.201801102
41. Cui H, Zhu W, Huang Y, et al., 2019, In vitro and in vivo Evaluation of 3D Bioprinted Small-diameter Vasculature with Smooth Muscle and Endothelium Biofabrication, 12:015004. https://doi.org/10.1088/1758-5090/ab402c
42. Pennings I, Van Haaften EE, Jungst T, et al., 2019, Layerspecific Cell Differentiation in Bi-layered Vascular Grafts under Flow Perfusion. Biofabrication, 12:015009. https://doi.org/10.1088/1758-5090/ab47f0
43. Available from: https://www.cancer.gov/about-cancer/treatment/types/immunotherapy/angiogenesis-inhibitorsfact-sheet [Last accessed on 2022 Aug 22].
44. McCormack PL, Keam SJ, 2008, Bevacizumab. Drugs, 68:487–506.
45. Blick SK, Keating GM, Wagstaff AJ, 2007, Ranibizumab. Drugs, 67:1199–206. https://doi.org/10.2165/00003495-200767080-00007
46. Javle M, Smyth EC, Chau I, 2014, Ramucirumab: Successfully Targeting Angiogenesis in Gastric Cancer. Clin Cancer Res, 20:5875–81. https://doi.org/10.1158/1078-0432.CCR-14-1071
47. Holash J, Davis S, Papadopoulos N, et al., 2002, VEGF-Trap: A VEGF Blocker with Potent Antitumor Effects. Proc Nat Acad Sci U S A, 99:11393–8. https://doi.org/10.1073/pnas.172398299
48. Ciombor KK, Berlin J, Chan E, 2013, Aflibercept. Clin Cancer Res, 19:1920–5. https://doi.org/10.1158/1078-0432.CCR-12-2911
49. Wilhelm SM, Dumas J, Adnane L, et al., 2011, Regorafenib (BAY 73-4506): A New Oral Multikinase Inhibitor of Angiogenic, Stromal and Oncogenic Receptor Tyrosine Kinases with Potent Preclinical Antitumor Activity. Int J Cancer, 129:245–55. https://doi.org/10.1002/ijc.25864
50. Strumberg D, Schultheis B, 2012, Regorafenib for Cancer. Expert Opin Investig Drugs, 21:879–89. https://doi.org/10.1517/13543784.2012.684752