Fused Deposition Modeling PEEK Implants for Personalized Surgical Application: From Clinical Need to Biofabrication
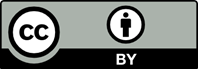
Three-dimensional printing (3DP) technology is suitable for manufacturing personalized orthopedic implants for reconstruction surgery. Compared with traditional titanium, polyether-ether-ketone (PEEK) is the ideal material for 3DP orthopedic implants due to its various advantages, including thermoplasticity, thermal stability, high chemical stability, and radiolucency suitable elastic modulus. However, it is challenging to develop a well-designed method and manufacturing technique to meet the clinical needs because it requires elaborate details and interplays with clinical work. Furthermore, establishing surgical standards for new implants requires many clinical cases and an accumulation of surgical experience. Thus, there are few case reports on using 3DP PEEK implants in clinical practice. Herein, we formed a team with a lot of engineers, scientists, and doctors and conducted a series of studies on the 3DP PEEK implants for chest wall reconstruction. First, the thoracic surgeons sort out the specific types of chest wall defects. Then, the engineers designed the shape of the implant and performed finite element analysis for every implant. To meet the clinical needs and mechanical requirements of implants, we developed a new fused deposition modeling technology to make personalized PEEK implants. Overall, the thoracic surgeons have used 114 personalized 3DP PEEK implants to reconstruct the chest wall defect and further established the surgical standards of the implants as part of the Chinese clinical guidelines. The surface modification technique and composite process are developed to overcome the new clinical problems of implant-related complications after surgery. Finally, the major challenges and possible solutions to translating 3DP PEEK implants into a mature and prevalent clinical product are discussed in the paper
1. Ambrosi A, Pumera M, 2016, 3D-printing technologies for electrochemical applications. Chem Soc Rev, 45:2740–55. https://doi.org/10.1039/c5cs00714c
2. Hu G, Kang J, Ng LW, et al., 2018, Functional inks and printing of two-dimensional materials. Chem Soc Rev, 47:3265–300. https://doi.org/10.1039/c8cs00084k
3. Layani M, Wang X, Magdassi S, 2018, Novel Materials for 3D Printing by Photopolymerization. Adv Mater, 30:e1706344. https://doi.org/10.1002/adma.201706344
4. Hirt L, Reiser A, Spolenak R, et al., 2017, Additive manufacturing of metal structures at the micrometer scale. Adv Mater, 29 :1604211. https://doi.org/10.1002/adma.201604211
5. Liaw CY, Guvendiren M, 2017, Current and emerging applications of 3D printing in medicine. Biofabrication, 9:024102. https://doi.org/10.1088/1758-5090/aa7279
6. Martelli N, Serrano C, van den Brink H, et al., 2016, Advantages and disadvantages of 3-dimensional printing in surgery: A systematic review. Surgery, 159:1485–500. https://doi.org/10.1016/j.surg.2015.12.017
7. Khorsandi D, Fahimipour A, Abasian P, et al., 2021, 3D and 4D printing in dentistry and maxillofacial surgery: Printing techniques, materials, and applications. Acta Biomater, 122:26–49. https://doi.org/10.1016/j.actbio.2020.12.044
8. Burnard JL, Parr WC, Choy WJ, et al., 2020, 3D-printed spine surgery implants: A systematic review of the efficacy and clinical safety profile of patient-specific and off-the-shelf devices. Eur Spine J, 29:1248–60. https://doi.org/10.1007/s00586-019-06236-2
9. Melville JC, Manis CS, Shum JW, et al., 2019, Single-Unit 3D-Printed Titanium Reconstruction Plate for Maxillary Reconstruction: The Evolution of Surgical Reconstruction for Maxillary Defects-A Case Report and Review of Current Techniques. J Oral Maxillofac Surg, 77:874.e1–13. https://doi.org/10.1016/j.joms.2018.11.030
10. Kamel MK, Cheng A, Vaughan B, et al., 2020, Sternal reconstruction using customized 3D-printed titanium implants. Ann Thorac Surg, 109:e411–4. https://doi.org/10.1016/j.athoracsur.2019.09.087
11. Wang L, Cao T, Li X, et al., 2016, Three-dimensional printing titanium ribs for complex reconstruction after extensive posterolateral chest wall resection in lung cancer. J Thorac Cardiovasc Surg, 152:e5–7. https://doi.org/10.1016/j.jtcvs.2016.02.064
12. Anderson LA, Christie M, Blackburn BE, et al., 2021, 3D-printed titanium metaphyseal cones in revision total knee arthroplasty with cemented and cementless stems. Bone Joint J, 103-b 6 Suppl A:150–7. https://doi.org/10.1302/0301-620x.103b6.Bjj-2020-2504.R1
13. Moradiellos J, Amor S, Córdoba M, et al., 2017, Functional chest wall reconstruction with a biomechanical three-dimensionally printed implant. Ann Thorac Surg, 103:e389–91. https://doi.org/10.1016/j.athoracsur.2016.11.048
14. Wang L, Huang L, Li X, et al., 2019, Three-dimensional printing PEEK implant: A novel choice for the reconstruction of chest wall defect. Ann Thorac Surg, 107:921–8. https://doi.org/10.1016/j.athoracsur.2018.09.044
15. Aragon J, Perez Mendez I, 2016, Dynamic 3D printed titanium copy prosthesis: A novel design for large chest wall resection and reconstruction. J Thorac Dis, 8:E385–9. https://doi.org/10.21037/jtd.2016.03.94
16. Kurtz SM, editor. 2019, An overview of PEEK biomaterials. In: PEEK Biomaterials Handbook. 2nd ed., Ch. 1. Norwich, NY: William Andrew Publishing. p3–9.
17. Bessard E, De Almeida O, Bernhart G, 2014, Unified isothermal and non-isothermal modelling of neat PEEK crystallization. J Therm Anal Calorim, 115:1669–78. https://doi.org/10.1007/s10973-013-3308-8
18. Katti KS, 2004, Biomaterials in total joint replacement. Colloids Surf B Biointerfaces, 39:133–42. https://doi.org/10.1016/j.colsurfb.2003.12.002
19. Stober EJ, Seferis JC, Keenan JD, 1984, Characterization and exposure of polyetheretherketone (PEEK) to fluid environments. Polymer, 25:1845–52. https://doi.org/10.1016/0032-3861(84)90260-X
20. Boinard E, Pethrick RA, MacFarlane CJ, 2000, The influence of thermal history on the dynamic mechanical and dielectric studies of polyetheretherketone exposed to water and brine. Polymer, 41:1063–76. https://doi.org/10.1016/S0032-3861(99)00259-1
21. Bishop MT, Karasz FE, Russo PS, et al., 1985, Solubility and properties of a poly(aryl ether ketone) in strong acids. Macromolecules, 18:86–93. https://doi.org/10.1021/ma00143a014
22. Li HM, Fouracre RA, Given MJ, et al., 1999, The effects on polyetheretherketone and polyethersulfone of electron and/spl gamma/irradiation. IEEE Trans Dielectr Electr Insul, 6:295–303. https://doi.org/10.1109/94.775614
23. Kurtz SM, Devine JN, 2007, PEEK biomaterials in trauma, orthopedic, and spinal implants. Biomaterials, 28:4845–69. https://doi.org/10.1016/j.biomaterials.2007.07.013
24. Ouyang L, Chen M, Wang D, et al., 2019, Nano Textured PEEK Surface for Enhanced Osseointegration. ACS Biomater Sci Eng, 5:1279–89. https://doi.org/10.1021/acsbiomaterials.8b01425
25. Han X, Yang D, Yang C, et al., 2019, Carbon fiber reinforced PEEK composites based on 3D-printing technology for orthopedic and dental applications. J Clin Med, 8:240. https://doi.org/10.3390/jcm8020240
26. Katzer A, Marquardt H, Westendorf J, et al., 2002, Polyetheretherketone—cytotoxicity and mutagenicity in vitro. Biomaterials, 23:1749–59. https://doi.org/10.1016/S0142-9612(01)00300-3
27. Toth JM, Wang M, Estes BT, et al., 2006, Polyetheretherketone as a biomaterial for spinal applications. Biomaterials, 27:324–34. https://doi.org/10.1016/j.biomaterials.2005.07.011
28. Stratton-Powell AA, Pasko KM, Brockett CL, et al., 2016, The biologic response to polyetheretherketone (PEEK) wear particles in total joint replacement: A systematic review. Clin Orthop Relat Res, 474:2394–404. https://doi.org/10.1007/s11999-016-4976-z
29. Wang L, Liu X, Jiang T, et al., 2020, Three-dimensional printed polyether-ether-ketone implant for extensive chest wall reconstruction: A case report. Thorac Cancer, 11:2709–12. https://doi.org/10.1111/1759-7714.13560
30. Lethaus B, Ter Laak MP, Laeven P, et al., 2011, A treatment algorithm for patients with large skull bone defects and first results. J Craniomaxillofac Surg, 39:435–40. https://doi.org/10.1016/j.jcms.2010.10.003
31. Kim MM, Boahene KD, Byrne PJ, 2009, Use of customized polyetheretherketone (PEEK) implants in the reconstruction of complex maxillofacial defects. Arch Facial Plast Surg, 11:53–7. https://doi.org/10.1001/archfaci.11.1.53
32. Najeeb S, Zafar MS, Khurshid Z, et al., 2016, Applications of polyetheretherketone (PEEK) in oral implantology and prosthodontics. J Prosthodont Res, 60:12–9. https://doi.org/10.1016/j.jpor.2015.10.001
33. Panayotov IV, Orti V, Cuisinier F, et al., 2016, Polyetheretherketone (PEEK) for medical applications. J Mater Sci Mater Med, 27:118. https://doi.org/10.1007/s10856-016-5731-4
34. Seaman S, Kerezoudis P, Bydon M, et al., 2017, Titanium vs. polyetheretherketone (PEEK) interbody fusion: Meta-analysis and review of the literature. J Clin Neurosci, 44:23–9. https://doi.org/10.1016/j.jocn.2017.06.062
35. Zanjanijam AR, Major I, Lyons JG, et al., 2020, Fused filament fabrication of PEEK: A review of process-structure property relationships. Polymers (Basel), 12:1665. https://doi.org/10.3390/polym12081665
36. Sun C, Jin Z, Wang L, et al., 2017, In 8th WACBE World Congress on Bioengineering.
37. Kang J, Wang L, Yang C, et al., 2018, Custom design and biomechanical analysis of 3D-printed PEEK rib prostheses. Biomech Model Mechanobiol, 17:1083–92. https://doi.org/10.1007/s10237-018-1015-x
38. Zhang C, Wang L, Kang J, et al., 2020, Bionic design and verification of 3D printed PEEK costal cartilage prosthesis. J Mech Behav Biomed Mater, 103:103561. https://doi.org/10.1016/j.jmbbm.2019.103561
39. Yang C, Wang B, Li D, et al., 2017, Modelling and characterisation for the responsive performance of CF/PLA and CF/PEEK smart materials fabricated by 4D printing. Virtual Phys Prototyp, 12:69–76. https://doi.org/10.1080/17452759.2016.1265992
40. Yang C, Tian X, Li D, et al., 2017, Influence of thermal processing conditions in 3D printing on the crystallinity and mechanical properties of PEEK material. J Mater Process Technol, 248:1–7. https://doi.org/10.1016/j.jmatprotec.2017.04.027
41. Yang C, Tian X, Liu T, et al., 2017, 3D printing for continuous fiber reinforced thermoplastic composites: Mechanism and performance. Rapid Prototyp J, 23:209–15. https://doi.org/10.1108/rpj-08-2015-0098
42. Li D, Yang C, Kang J, et al., 2018, Precision design and control-performance manufacturing research of large-size individualized PEEK implants. J Mech, 54:121–5. https://doi.org/10.3901/JME.2018.23.121
43. Wang L, Li J, Zhong D, 2019, Chinese expert consensus on chest wall tumor resection and chest wall reconstruction (version 2018). Chin J Clin Thorac Cardiovasc Surg, 26:1–7. https://doi.org/10.7507/1007-4848.201809058
44. Wang L, Yan X, Zhao J, et al., 2021, Expert consensus on resection of chest wall tumors and chest wall reconstruction. Transl Lung Cancer Res, 10:4057–83. https://doi.org/10.21037/tlcr-21-935
45. Su Y, He J, Jiang N, et al., 2020, Additively-manufactured poly-ether-ether-ketone (PEEK) lattice scaffolds with uniform microporous architectures for enhanced cellular response and soft tissue adhesion. Mater Des, 191:108671. https://doi.org/10.1016/j.matdes.2020.108671
46. Liu X, Huang L, Zhang H, et al., 2021, Facile Amidogen Bio‐ Activation Method Can Boost the Soft Tissue Integration on 3D Printed Poly–Ether–Ether–Ketone Interface. Adv Mater Interfaces, 8 :2100547. https://doi.org/10.1002/admi.202100547
47. Zheng J, Kang J, Sun C, et al., 2021, Effects of printing path and material components on mechanical properties of 3D-printed polyether-ether-ketone/hydroxyapatite composites. J Mech Behav Biomed Mater, 118:104475. https://doi.org/10.1016/j.jmbbm.2021.104475
48. Zheng J, Zhao H, Dong E, et al., 2021, Additively-manufactured PEEK/HA porous scaffolds with highly-controllable mechanical properties and excellent biocompatibility. Mater Sci Eng C Mater Biol Appl, 128:112333. https://doi.org/10.1016/j.msec.2021.112333
49. Zheng J, Dong E, Kang J, et al., 2021, Effects of Raster Angle and Material Components on Mechanical Properties of Polyether-Ether-Ketone/Calcium Silicate Scaffolds. Polymers (Basel), 13:2547. https://doi.org/10.3390/polym13152547
50. Smith JA, Ho VP, Towe CW, 2018, Using 3-Dimensional Modeling to Customize Titanium Plates for Repair of Chest Wall Trauma. Surg Innov, 25:115–20. https://doi.org/10.1177/1553350617753225
51. Cano JR, Escobar FH, Alonso DP, et al., 2018, Reconstruction of the anterior chest wall with a 3-dimensionally printed biodynamic prosthesis. J Thorac Cardiovasc Surg, 155:e59–60. https://doi.org/10.1016/j.jtcvs.2017.08.118
52. Goriainov V, Cook R, Latham JM, et al., 2014, Bone and metal: An orthopaedic perspective on osseointegration of metals. Acta Biomater, 10:4043–57. https://doi.org/10.1016/j.actbio.2014.06.004
53. Prakash M, Ong Q, Lo C, et al., 2020, Rib Cage Stabilisation with 3D-Printed Polyethylene Sternal Prosthesis Post-Sternotomy Mediastinitis. Heart Lung Circ, 29:1561–5. https://doi.org/10.1016/j.hlc.2020.01.005
54. Mohamed OA, Masood SH, Bhowmik JL, 2015, Optimization of fused deposition modeling process parameters: A review of current research and future prospects. Adv Manuf, 3:42–53. https://doi.org/10.1007/s40436-014-0097-7
55. Wickramasinghe S, Do T, Tran P, 2020, FDM-based 3D printing of polymer and associated composite: A review on mechanical properties, defects and treatments. Polymers (Basel), 12:1529. https://doi.org/10.3390/polym12071529
56. Turner BN, Gold SA, 2015, A review of melt extrusion additive manufacturing processes: II. Materials, dimensional accuracy, and surface roughness. Rapid Prototyp J, 21:250–61. https://doi.org/10.1108/rpj-02-2013-0017
57. Li Z, Kindig MW, Kerrigan JR, et al., 2010, Rib fractures under anterior-posterior dynamic loads: Experimental and finite-element study. J Biomech, 43:228–34. https://doi.org/10.1016/j.jbiomech.2009.08.040
58. Li Z, Kindig MW, Subit D, et al., 2010, Influence of mesh density, cortical thickness and material properties on human rib fracture prediction. Med Eng Phys, 32:998–1008. https://doi.org/10.1016/j.medengphy.2010.06.015
59. Griffin MF, O’Toole G, Sabbagh W, et al., 2020, Comparison of the compressive mechanical properties of auricular and costal cartilage from patients with microtia. J Biomech, 103:109688. https://doi.org/10.1016/j.jbiomech.2020.109688
60. Guo BY, Liao DH, Li XY, et al., 2007, Age and gender related changes in biomechanical properties of healthy human costal cartilage. Clin Biomech (Bristol, Avon), 22:292–7. https://doi.org/10.1016/j.clinbiomech.2006.10.004
61. Melchels FP, Domingos MA, Klein TJ, et al., 2012, Additive manufacturing of tissues and organs. Prog Polym Sci, 37:1079–104. https://doi.org/10.1016/j.progpolymsci.2011.11.007
62. Gross BC, Erkal JL, Lockwood SY, et al., 2014, Evaluation of 3D printing and its potential impact on biotechnology and the chemical sciences. Anal Chem, 86:3240–53. https://doi.org/10.1021/ac403397r
63. Rengier F, Mehndiratta A, von Tengg-Kobligk H, et al., 2010, 3D printing based on imaging data: Review of medical applications. Int J Comput Assist Radiol Surg, 5:335–41. https://doi.org/10.1007/s11548-010-0476-x
64. Leukers B, Gülkan H, Irsen SH, et al., 2005, Hydroxyapatite scaffolds for bone tissue engineering made by 3D printing. J Mater Sci Mater Med, 16:1121–4. https://doi.org/10.1007/s10856-005-4716-5
65. Deng L, Deng Y, Xie K, 2017, AgNPs-decorated 3D printed PEEK implant for infection control and bone repair. Colloids Surf B Biointerfaces, 160:483–92. https://doi.org/10.1016/j.colsurfb.2017.09.061
66. El Magri A, El Mabrouk K, Vaudreuil S, et al., 2020, Optimization of printing parameters for improvement of mechanical and thermal performances of 3D printed poly(ether ether ketone) parts. J Appl Polym Sci, 137:49087. https://doi.org/10.1002/app.49087
67. Berman B, 2012, 3-D printing: The new industrial revolution. Bus Horiz, 55:155–62. https://doi.org/10.1016/j.bushor.2011.11.003
68. Hu B, Duan X, Xing Z, et al., 2019, Improved design of fused deposition modeling equipment for 3D printing of highperformance PEEK parts. Mech Mater, 137:3139. https://doi.org/10.1016/j.mechmat.2019.103139
69. Wang R, Cheng KJ, Advincula RC, et al., 2019, On the thermal processing and mechanical properties of 3D-printed polyether ether ketone. MRS Commun, 9:1046–52. https://doi.org/10.1557/mrc.2019.86
70. Wu W, Geng P, Li G, et al., 2015, Influence of Layer Thickness and Raster Angle on the Mechanical Properties of 3D-Printed PEEK and a Comparative Mechanical Study between PEEK and ABS. Materials, 8:5834–46. https://doi.org/10.3390/ma8095271
71. Deng X, Zeng Z, Peng B, et al., 2018, Mechanical properties optimization of poly-ether-ether-ketone via fused deposition modeling. Materials, 11:20216. https://doi.org/10.3390/ma11020216
72. Sandri A, Donati G, Blanc CD, et al., 2020, Anterior chest wall resection and sternal body wedge for primary chest wall tumour: Reconstruction technique with biological meshes and titanium plates. J Thorac Dis, 12:17–21. https://doi.org/10.21037/jtd.2019.06.45
73. Lardinois D, Müller M, Furrer M, et al., 2000, Functional assessment of chest wall integrity after methylmethacrylate reconstruction. Ann Thorac Surg, 69:919–23. https://doi.org/10.1016/S0003-4975(99)01422-8
74. Nishida Y, Tsukushi S, Urakawa H, et al., 2015, Postoperative pulmonary and shoulder function after sternal reconstruction for patients with chest wall sarcomas. Int J Clin Oncol, 20:1218–25. https://doi.org/10.1007/s10147-015-0844-1
75. Daigeler A, Druecke D, Hakimi M, et al., 2009, Reconstruction of the thoracic wall-long-term follow-up including pulmonary function tests. Langenbecks Arch Surg, 394:705–15. https://doi.org/10.1007/s00423-008-0400-9
76. Leuzzi G, Nachira D, Cesario A, et al., 2015, Chest wall tumors and prosthetic reconstruction: A comparative analysis on functional outcome. Thorac Cancer, 6:247–54. https://doi.org/10.1111/1759-7714.12172