Research Progress of Robot Technology in In situ 3D Bioprinting
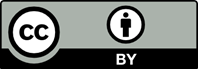
Three-dimensional (3D) bioprinting is an emerging research direction in bio-manufacturing, a landmark in the shift from traditional manufacturing to high-end manufacturing. It integrates manufacturing science, biomedicine, information technology, and material science. In situ bioprinting is a type of 3D bioprinting which aims to print tissues or organs directly on defective sites in the human body. Printed materials can grow and proliferate in the human body; therefore, the graft is similar to the target tissues or organs and could accurately match the defective site. This article mainly summarizes the current status of robotic applications in the medical field and reviews its research progress in in situ 3D bioprinting.
1. Singh S, Choudhury D, Yu F, et al., 2020, In Situ Bioprinting-Bioprinting from Benchside to Bedside? Acta Biomater, 2020,101:14–25. https://doi.org/10.1016/j.actbio.2019.08.045
2. Vijayavenkataraman S, Yan WC, Lu WF, et al., 2018, 3D Bioprinting of Tissues and Organs for Regenerative Medicine. Adv Drug Deliv Rev, 132:296–332. https://doi.org/10.1016/j.addr.2018.07.004
3. Keriquel V, Guillemot F, Arnault I, et al., 2020, In Vivo Bioprinting for Computer and Robotic-assisted Medical Intervention: Preliminary Study in Mice. Biofabrication, 2:014101. https://doi.org/10.1088/1758-5082/2/1/014101
4. Ding H, Chang RC, 2018, Simulating Image-guided In Situ Bioprinting of a Skin Graft onto a Phantom Burn Wound Bed. Addit Manuf, 22:708–19. https://doi.org/10.1016/j.addma.2018.06.022
5. Wang M, He J, Liu Y, et al., 2015, The Trend Towards In Vivo Bioprinting. Int J Bioprint, 1:15-26. http://dx.doi.org/10.18063/IJB.2015.01.001
6. Ma K, Zhao T, Yang L, et al., 2020, Application of Robotic assisted In Situ 3D Printing in Cartilage Regeneration with HAMA Hydrogel: An In Vivo Study. J Adv Res, 23:123–32. https://doi.org/10.1016/j.jare.2020.01.010
7. Ashammakhi N, Ahadian S, Pountos I, et al., 2019, In Situ Three-dimensional Printing for Reparative and Regenerative Therapy. Biomed Microdevices, 21:42. https://doi.org/10.1007/s10544-019-0372-2
8. Chong C, Wang Y, Fathi A, et al., 2019, Skin Wound Repair: Results of a Pre-Clinical Study to Evaluate Electropsun Collagen-Elastin-PCL Scaffolds as Dermal Substitutes. Burns, 45:1639–48. https://doi.org/10.1016/j.burns.2019.04.014
9. Cheng RY, Gertraud E, Jean-Michel G, et al., 2020, Handheld Instrument for Wound-Conformal Delivery of Skin Precursor Sheets Improves Healing in Full-Thickness Burns. Biofabrication, 12:025002. https://doi.org/10.1088/1758-5090/ab6413
10. Navid H, Richard C, Lian L, et al., 2018, Handheld Skin Printer: In Situ Formation of Planar Biomaterials and Tissues. Lab Chip, 18:1440–51. https://doi.org/10.1039/c7lc01236e
11. Albanna M, Binder KW, Murphy SV, et al., 2019, In Situ Bioprinting of Autologous Skin Cells Accelerates Wound Healing of Extensive Excisional Full-Thickness Wounds. Sci Rep, 9:1856. https://doi.org/10.1038/s41598-018-38366-w
12. Wang H, Lian Q, Li D, et al., 2021, Multi-Tissue Layering and Path Planning of In Situ Bioprinting for Complex Skin and Soft Tissue Defects. Rapid Prototyp J, 27:321–32. https://doi.org/10.1108/rpj-08-2020-0201
13. Peng L, Zhou Y, Lu W, et al., 2019, Characterization of a Novel Polyvinyl Alcohol/Chitosan Porous Hydrogel Combined with Bone Marrow Mesenchymal Stem Cells and its Application in Articular Cartilage Repair. BioMed Central, 20:257. https://doi.org/10.1186/s12891-019-2644-7
14. Pengcheng T, Yang G, Suyang Z, et al., 2019, Research Progress on Signaling Molecules Involved in Articular Cartilage Repair. Sheng Wu Yi Xue Gong Cheng Xue Za Zhi., 36:343–8. https://doi.org/10.7507/1001-5515.201806021
15. Yifan D, 2018, 3D Printed Osteochondral Integrated Repair Gradient Hybrid Scaffold and its Performance. Guangzhou, China: South China University of Technology.
16. Yang W, Patrick K, Nicholas B, et al., 2021, Three- Dimensional Bioprinting of Articular Cartilage: A Systematic Review. Cartilage, 12:76–92. https://doi.org/10.1177/1947603518809410
17. O’Connell CD, Di Bella C, Fletcher T, et al., 2016, Development of the Biopen: A Handheld Device for Surgical Printing of Adipose Stem Cells at a Chondral Wound Site. Biofabrication, 8:015019. https://doi.org/10.1088/1758-5090/8/1/015019
18. Bella CD, Duchi S, O’Connell CD, et al., 2018, In-Situ Handheld 3D Bioprinting for Cartilage Regeneration. J Tissue Eng Regen Med, 12:611–21. https://doi.org/10.1002/term.2476
19. Duchi S, Onofrillo C, Connell CO, et al., 2017, Handheld Co Axial Bioprinting: Application to In Situ Surgical Cartilage Repair. Sci Rep, 7:5837. https://doi.org/10.1038/s41598-017-05699-x
20. Chen Y, Zhang J, Liu X, et al., 2020, Noninvasive In Vivo 3D Bioprinting. Sci Adv, 6:eaba7406.
21. Wenying W, Honglian D, 2021, Articular Cartilage and Osteochondral Tissue Engineering Techniques: Recent Advances and Challenges. Bioact Mater, 6:4830–55. https://doi.org/10.1016/j.bioactmat.2021.05.011
22. Meiling W, Shuifeng L, Da H, et al., 2020, Biocompatible Heterogeneous Bone Incorporated with Polymeric Biocomposites for Human Bone Repair by 3D Printing Technology. J Appl Polym Sci, 138:50114. https://doi.org/10.1002/app.50114
23. Keriquel V, Oliveira H, Rémy M, et al., 2017, In Situ Printing of Mesenchymal Stromal Cells, by Laser-Assisted Bioprinting, for In Vivo Bone Regeneration Applications. Sci Rep, 7:1778. https://doi.org/10.1038/s41598-017-01914-x
24. Malyshev I, Runova G, Poduraev Y, et al., 2018, Natural Amelogenesis and Rationale for Enamel Regeneration by Means of Robotic Bioprinting of Tissues In Situ. Stomatologiia (Mosk), 97:58–64. https://doi.org/10.17116/stomat201897258-64
25. Campos D, Zhang S, Kreimendahl F, et al., 2020, Hand-Held Bioprinting for De Novo Vascular Formation Applicable to Dental Pulp Regeneration. Connect Tissue Res, 61:205–15. https://doi.org/10.1080/03008207.2019.1640217
26. Lopes HJ, Regina C, Janaína D, et al., 2020, Piezoelectric 3D Bioprinting for Ophthalmological Applications: Process Development and Viability Analysis of the Technology. Biomed Phys Eng Express, 6:035021. https://doi.org/10.1088/2057-1976/ab7bf9
27. Bergeles C, 2014, From Passive Tool Holders to Microsurgeons: Safer, Smaller, Smarter Surgical Robots. IEEE Trans Biomed Eng, 61:1565–76. https://doi.org/10.1109/TBME.2013.2293815
28. Gifari MW, Naghibi H, Stramigioli S, et al., 2019, A Review on Recent Advances in Soft Surgical Robots for Endoscopic Applications. Int J Med Robot, 15:e2010. https://doi.org/10.1002/rcs.2010
29. Kinross JM, Mason SE, Mylonas G, et al., 2020, Next-Generation Robotics in Gastrointestinal Surgery. Nat Rev Gastroenterol Hepatol, 17:430–40. https://doi.org/10.1038/s41575-020-0290-z
30. Chen Y, Zhang S, Wu Z, et al., 2020, Review of Surgical Robotic Systems for Keyhole and Endoscopic Procedures: State of the Art and Perspectives. Front Med, 14:382–403. https://doi.org/10.1007/s11684-020-0781-x
31. Zhang W, Li H, Cui L, et al., 2021, Research Progress and Development Trend of Surgical Robot and Surgical Instrument Arm. Int J Med Robot, 17:e2309. https://doi.org/10.1002/rcs.2309
32. D’Souza M, Gendreau J, Feng A, et al., 2019, Robotic-Assisted Spine Surgery: History, Efficacy, Cost, and Future Trends. Robot Surg, 6:9–23. https://doi.org/10.2147/rsrr.s190720
33. Kaushal M, Kurpad S, Choi H, 2019, Robotic-Assisted Systems for Spinal Surgery. In: Neurosurgical Procedures Innovative Approaches. London: IntechOpen. https://doi.org/10.5772/intechopen.88730
34. Ming H, Chin PL, Tay K, et al., 2014, Early Experiences with Robot-Assisted Total Knee Arthroplasty using the DigiMatch™igiMatche Surgical System. Singapore Med J, 55:529–34. https://doi.org/10.11622/smedj.2014136
35. Banerjee S, Cherian JJ, Elmallah RK, et al., 2015, Robotic-Assisted Knee Arthroplasty. Expert Rev Med Devices, 12:727–35. https://doi.org/10.1586/17434440.2015.1086264
36. Liow MH, Chin PL, Pang HN, et al., 2017, THINK surgical TSolution-One® (Robodoc) total knee arthroplasty. SICOT J, 3:63. https://doi.org/10.1051/sicotj/2017052
37. Reddy VY, Neuzil P, Malchano ZJ, et al., 2007, View-Synchronized Robotic Image-Guided Therapy for Atrial Fibrillation Ablation: Experimental Validation and Clinical Feasibility. Circulation, 115:2705–14. https://doi.org/10.1161/circulationaha.106.677369
38. Jayender J, Patel RV, Nikumb S, 2006, Robot-Assisted Catheter Insertion Using Hybrid Impedance Control: Robotics and Automation, 2006. ICRA 2006. Proceedings 2006 IEEE International Conference on, 2006. https://doi.org/10.1109/robot.2006.1641777
39. Beyar R, 2010, Navigation within the Heart and Vessels in Clinical Practice. Ann N Y Acad Sci, 1188:207–213. https://doi.org/10.1111/j.1749-6632.2009.05102.x
40. Zhang X, Ma X, Zhou J, et al., 2018, Summary of Medical Robot Technology Development: 2018 IEEE International Conference on Mechatronics and Automation (ICMA), 2018. https://doi.org/10.1109/icma.2018.8484458
41. Sutherland GR, Wolfsberger S, Lama S, et al., 2013, The Evolution of neuroArm. Neurosurgery, 72:27–32. https://doi.org/10.1227/NEU.0b013e318270da19
42. Minchev G, Kronreif G, Martínez-Moreno M, et al., 2017, A Novel Miniature Robotic Guidance Device for Stereotactic Neurosurgical Interventions: Preliminary Experience with the iSYS1 Robot. J Neurosurg, 126:985–96. https://doi.org/10.3171/2016.1.JNS152005
43. Wang T, Zhao Q, Gu J, et al., 2019, Neurosurgery Medical Robot Remebot for the Treatment of 17 Patients with Hypertensive Intracerebral Hemorrhage. Int J Med Robot, 15:e2024. https://doi.org/10.1002/rcs.2024
44. Umebayashi D, Mandai A, Osaka Y, et al., 2010, Effects and Complications of Stereotactic Aspiration for Spontaneous Intracerebral Hemorrhage. Neurol Med Chir (Tokyo), 50:538–44. https://doi.org/10.2176/nmc.50.538
45. Tran DK, Paff M, Mnatsakanyan L, et al., 2020, A Novel Robotic-Assisted Technique to Implant the Responsive Neurostimulation System. Oper Neurosurg (Hagerstown), 18:728–35. https://doi.org/10.1093/ons/opz226
46. Liu L, Mariani SG, Schlichting ED, et al., 2020, Frameless ROSA® Robot-Assisted Lead Implantation for Deep Brain Stimulation: Technique and Accuracy. Oper Neurosurg (Hagerstown), 19:57–64. https://doi.org/10.1093/ons/opz320
47. Lipskas J, Deep K, Yao W, 2019, Robotic-Assisted 3D Bioprinting Repairing Bone and Cartilage Defects through a Minimally Invasive Approach. Sci Rep, 9:3746. https://doi.org/10.1038/s41598-019-38972-2
48. Fortunato GM, Rossi G, Bonatti AF, et al., 2021, Robotic Platform and Path Planning Algorithm for In Situ Bioprinting. Bioprinting, 22:e00139. https://doi.org/10.1016/j.bprint.2021.e00139
49. Fortunato GM, Batoni E, Bonatti AF, et al., 2022, Surface Reconstruction and Tissue Recognition for Robotic-Based In Situ Bioprinting. Bioprinting, 26:e00195. https://doi.org/10.1016/j.bprint.2022.e00195
50. Zhao W, Wenxiang Z, Tao X, 2020, Preliminary Engineering for In Situ In Vivo Bioprinting: A Novel Micro Bioprinting Platform For In Situ In Vivo Bioprinting at a Gastric Wound Site. Biofabrication, 12:045020. https://doi.org/10.1088/1758-5090/aba4ff
51. Wenxiang Z, Haiyan C, Yi Z, et al., 2022, Adaptive Multidegree-of-Freedom In Situ Bioprinting Robot for Hair-Follicle-Inclusive Skin Repair: A Preliminary Study Conducted in Mice. Bioeng Transl Med, 2022:e10303. https://doi.org/10.1002/btm2.10303/v3/response1
52. Zhang Z, Wu C, Dai C, et al., 2022, A Multi-axis Robot based Bioprinting System Supporting Natural Cell Function Preservation and Cardiac Tissue Fabrication. Bioactive Mater, 18:138–50.
https://doi.org/10.1016/j.bioactmat.2022.02.009