In vitro Evaluation of a 20% Bioglass-Containing 3D printable PLA Composite for Bone Tissue Engineering
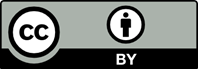
Three-dimensional (3D) printing is considered a key technology in the production of customized scaffolds for bone tissue engineering. In a previous work, we developed a 3D printable, osteoconductive, hierarchical organized scaffold system. The scaffold material should be osteoinductive. Polylactic acid (PLA) (polymer)/Bioglass (BG) (mineral/ion source) composite materials are promising. Previous studies of PLA/BG composites never exceed BG fractions of 10%, as increase of bioactive BG component negatively affects the printability of the composite material. Here, we test a novel, 3D printable PLA/ BG composite with BG fractions up to 20% for its biological activity in vitro. PLA/BG filaments suitable for microstructure 3D printing were spun and the effect of different BG contents (5%, 10%, and 20%) in this material on mesenchymal stem cell (MSC) activity was tested in vitro. Our results showed that all tested composites are biocompatible. MSC cell adherence and metabolic activity increase with increasing BG content. The presence of BG component in scaffold has only slight effect on osteogenic gene expression, but it has significant suppressive effect on the expression of inflammatory genes in MSC. In addition, the material did not provoke any significant inflammatory response in whole-blood stimulation assay. The results show that by increasing the BG content, the bioactivity can be further enhanced.
1. Söhling N, Neijhoft J, Nienhaus V, et al., 2020, 3D-Printing of Hierarchically Designed and Osteoconductive Bone Tissue Engineering Scaffolds. Materials (Basel), 13:1836. https://doi.org/10.3390/ma13081836
2. Grémare A, Guduric V, Bareille R, et al., 2018, Characterization of Printed PLA Scaffolds for Bone Tissue Engineering. J Biomed Mater Res Part A, 106:887–94. https://doi.org/10.1002/jbm.a.36289
3. Lam CX, Hutmacher DW, Schantz JT, et al., 2009, Evaluation of Polycaprolactone Scaffold Degradation for 6 Months In Vitro and In Vivo. J Biomed Mater Res A, 90:906–19. https://doi.org/10.1002/jbm.a.32052
4. Shrivats AR, McDermott MC, Hollinger JO, 2014, Bone Tissue Engineering: State of the Union. Drug Discov Today, 19:781–6. https://doi.org/10.1016/j.drudis.2014.04.010
5. Mehrpouya M, Vahabi H, Barletta M, et al., 2021, Additive Manufacturing of Polyhydroxyalkanoates (PHAs) Biopolymers: Materials, Printing Techniques, and Applications. Mater Sci Eng C, 127:112216. https://doi.org/10.1016/j.msec.2021.112216
6. Kalia VC, Singh Patel SK, Shanmugam R, et al., 2021, Polyhydroxyalkanoates: Trends and Advances Toward Biotechnological Applications. Bioresour Technol, 326:124737. https://doi.org/10.1016/j.biortech.2021.124737
7. Eldesoqi K, Seebach C, Ngoc CN, et al., 2013, High Calcium Bioglass Enhances Differentiation and Survival of Endothelial Progenitor Cells, Inducing Early Vascularization in Critical Size Bone Defects. PLoS One, 8:e79058. https://doi.org/10.1371/journal.pone.0079058
8. Zhang J, Liu W, Schnitzler V, et al., 2014, Calcium Phosphate Cements for Bone Substitution: Chemistry, Handling and Mechanical Properties. Acta Biomater, 10:1035–49. https://doi.org/10.1016/j.actbio.2013.11.001
9. Henriksen SS, Ding M, Juhl MV, et al., 2011, Mechanical Strength of Ceramic Scaffolds Reinforced with Biopolymers is Comparable to that of Human Bone. J Mater Sci Mater Med, 22:1111–8. https://doi.org/10.1007/s10856-011-4290-y
10. Wu C, Fan W, Zhou Y, et al., 2012, 3D-Printing of Highly Uniform CaSiO3 Ceramic Scaffolds: Preparation, Characterization and In Vivo Osteogenesis. J Mater Chem, 22:12288–95. https://doi.org/10.1039/c2jm30566f
11. Trombetta R, Inzana JA, Schwarz EM, et al., 2017, 3D Printing of Calcium Phosphate Ceramics for Bone Tissue Engineering and Drug Delivery. Ann Biomed Eng, 45:23–44. https://doi.org/10.1007/s10439-016-1678-3
12. Hwang KS, Choi JW, Kim JH, et al., 2017, Comparative Efficacies of Collagen-Based 3D Printed PCL/PLGA/β-TCP Composite Block Bone Grafts and Biphasic Calcium Phosphate Bone Substitute for Bone Regeneration. Materials (Basel), 10:421. https://doi.org/10.3390/ma10040421
13. Pei F, Ping W, Chengde G, et al., 2018, A Multimaterial Scaffold with Tunable Properties: Toward Bone Tissue Repair. Adv Sci, 5:1700817. https://doi.org/10.1002/advs.201700817
14. Nyberg E, Rindone A, Dorafshar A, et al., 2017, Comparison of 3D-Printed Poly-ϵ-Caprolactone Scaffolds Functionalized with Tricalcium Phosphate, Hydroxyapatite, Bio-Oss, or Decellularized Bone Matrix. Tissue Eng Part A, 23:503–14. https://doi.org/10.1089/ten.tea.2016.0418
15. Scaffaro R, Lopresti F, Botta L, et al., 2016, Integration of PCL and PLA in a Monolithic Porous Scaffold for Interface Tissue Engineering. J Mech Behav Biomed Mater, 63:303–13. https://doi.org/10.1016/j.jmbbm.2016.06.021
16. Poh PS, Chhaya MP, Wunner FM, et al., 2016, Polylactides in Additive Biomanufacturing. Adv Drug Deliv Rev, 107:228–46. https://doi.org/10.1016/j.addr.2016.07.006
17. Ojansivu M, Wang X, Hyväri L, et al., 2018, Bioactive Glass Induced Osteogenic Differentiation of Human Adipose Stem Cells is Dependent on Cell Attachment Mechanism and Mitogen-Activated Protein Kinases. Eur Cells Mater, 35:54–72. https://doi.org/10.22203/eCM.v035a05
18. El-Rashidy AA, Roether JA, Harhaus L, et al., 2017, Regenerating Bone with Bioactive Glass Scaffolds: A Review of In Vivo Studies in Bone Defect Models. Acta Biomater, 62:1–28. https://doi.org/10.1016/j.actbio.2017.08.030
19. Popa AC, Stan GE, Husanu MA, et al., 2017, Bioglass Implant-Coating Interactions in Synthetic Physiological Fluids with Varying Degrees of Biomimicry. Int J Nanomedicine, 12:683–707. https://doi.org/10.2147/IJN.S123236
20. Saboori A, Rabiee M, Moztarzadeh F, et al., 2009, Synthesis, Characterization and In Vitro Bioactivity of Sol-Gel-Derived SiO2-CaO-P2O5-MgO Bioglass. Mater Sci Eng C, 29:335–40. https://doi.org/10.1016/j.msec.2008.07.004
21. Westhauser F, Karadjian M, Essers C, et al., 2019, Osteogenic Differentiation of Mesenchymal Stem Cells is Enhanced in a 45S5-Supplemented β-TCP Composite Scaffold: An In-Vitro Comparison of Vitoss and Vitoss BA. PLoS One, 14:1–18. https://doi.org/10.1371/journal.pone.0212799
22. Al Malat T, Glombitza M, Dahmen J, et al., 2018, The Use of Bioactive Glass S53P4 as Bone Graft Substitute in the Treatment of Chronic Osteomyelitis and Infected Non-Unions a Retrospective Study of 50 Patients Anwendung von Bioglas S53P4 als Knochenersatzmaterial Bei Chronischer Osteomyelitis Und Infe. Z Orthop Unfall, 156:152–159. https://doi.org/10.1055/s-0043-124377
23. Drago L, Toscano M, Bottagisio M, 2018, Recent Evidence on Bioactive Glass Antimicrobial and Antibiofilm Activity: A Mini-Review. Materials (Basel), 11:1–11. https://doi.org/10.3390/ma11020326
24. Lyyra I, Leino K, Hukka T, et al., 2021, Impact of Glass Composition on Hydrolytic Degradation of Polylactide/Bioactive Glass Composites. Materials (Basel), 14:1–20. https://doi.org/10.3390/ma14030667
25. Ng WL, Chua CK, Shen YF, 2019, Print Me An Organ! Why We Are Not There Yet. Prog Polym Sci, 97:101145. https://doi.org/10.1016/j.progpolymsci.2019.101145
26. Yang Y, Wang G, Liang H, et al., 2019, Additive Manufacturing of Bone Scaffolds. Int J Bioprint, 5:1–25. https://doi.org/10.18063/IJB.v5i1.148
27. Qu H, 2020, Additive Manufacturing for Bone Tissue Engineering Scaffolds. Mater Today Commun, 24:101024. https://doi.org/10.1016/j.mtcomm.2020.101024
28. Vergnol G, Ginsac N, Rivory P, et al., 2016, In Vitro and In Vivo Evaluation of a Polylactic Acid-Bioactive Glass Composite for Bone Fixation Devices. J Biomed Mater Res B Appl Biomater, 104:180–91. https://doi.org/10.1002/jbm.b.33364
29. Maquet V, Boccaccini AR, Pravata L, et al., 2004, Porous poly(α-hydroxyacid)/Bioglass® Composite Scaffolds for Bone Tissue Engineering. I: Preparation and In Vitro Characterisation. Biomaterials, 25:4185–94. https://doi.org/10.1016/j.biomaterials.2003.10.082
30. Estrada SA, Armendáriz IO, García AT, et al., 2017, Evaluation of In Vitro Bioactivity of 45S5 Bioactive Glass/Poly Lactic Acid Scaffolds Produced by 3D Printing. Int J Compos Mater, 7:144–9. https://doi.org/10.5923/j.cmaterials.20170705.03
31. Alksne M, Kalvaityte M, Simoliunas E, et al., 2020, In Vitro Comparison of 3d Printed Polylactic Acid/Hydroxyapatite and Polylactic Acid/Bioglass Composite Scaffolds: Insights Into Materials for Bone Regeneration. J Mech Behav Biomed Mater, 104:103641. https://doi.org/10.1016/j.jmbbm.2020.103641
32. Distler T, Fournier N, Grünewald A, et al., 2020, Polymer-Bioactive Glass Composite Filaments for 3D Scaffold Manufacturing by Fused Deposition Modeling: Fabrication and Characterization. Front Bioeng Biotechnol, 8:1–17. https://doi.org/10.3389/fbioe.2020.00552
33. Ginsac N, Chenal JM, Meille S, et al., 2011, Crystallization Processes at the Surface of Polylactic Acid-Bioactive Glass Composites During Immersion in Simulated Body Fluid. J Biomed Mater Res Part B Appl Biomater, 99B:412–9. https://doi.org/10.1002/jbm.b.31913
34. Henrich D, Verboket RR, Schaible A, et al., 2015, Characterization of Bone Marrow Mononuclear Cells on Biomaterials for Bone Tissue Engineering In Vitro. Biomed Res Int, 2015:762407. https://doi.org/10.1155/2015/762407
35. Seebach C, Henrich D, Tewksbury R, et al., 2007, Number and Proliferative Capacity of Human Mesenchymal Stem Cells are Modulated Positively in Multiple Trauma Patients and Negatively in Atrophic Nonunions. Calcif Tissue Int, 80:294–300. https://doi.org/10.1007/s00223-007-9020-6
36. Oliveira KM, Leppik L, Keswani K, et al., 2020, Electrical Stimulation Decreases Dental Pulp Stem Cell Osteo-/Odontogenic Differentiation. Biores Open Access, 9:162–73. https://doi.org/10.1089/biores.2020.0002
37. Schätzlein E, Kicker C, Söhling N, et al., (2022) 3D-printed PLA bioglass scaffolds with controllable calcium release and MSC adhesion for bone tissue engineering. Polymers, 14:2389. https://doi.org/10.3390/polym14122389
38. Yang J, Shi G, Bei J, et al., 2002, Fabrication and Surface Modification of Macroporous Poly(L-Lactic Acid) and Poly(LLactic-Co-Glycolic Acid) (70/30) Cell Scaffolds for Human Skin Fibroblast Cell Culture. J Biomed Mater Res, 62:438–46. https://doi.org/10.1002/jbm.10318
39. Johari N, Fathi MH, Golozar MA, et al., 2012, Poly(ECaprolactone)/Nano Fluoridated Hydroxyapatite Scaffolds for Bone Tissue Engineering: In Vitro Degradation and Biocompatibility Study. J Mater Sci Mater Med, 23:763–70. https://doi.org/10.1007/s10856-011-4528-8
40. Livak KJ, Schmittgen TD, 2001, Analysis of Relative Gene Expression Data Using Real- Time Quantitative PCR and the 2(-Delta Delta C(T)) Method. Methods, 408:402–8. https://doi.org/10.1006/meth.2001.1262
41. Wutzler S, Maier M, Lehnert M, et al., 2009, Suppression and Recovery of Lps-Stimulated Monocyte Activity after Trauma is Correlated with Increasing Injury Severity: A Prospective Clinical Study. J Trauma, 66:1273–80. https://doi.org/10.1097/TA.0b013e3181968054
42. Eldesoqi K, Henrich D, El-Kady AM, et al., 2014, Safety Evaluation of a Bioglass-Polylactic Acid Composite Scaffold Seeded with Progenitor Cells in a Rat Skull Critical-Size Bone Defect. PLoS One, 9:e87642. https://doi.org/10.1371/journal.pone.0087642
43. Azizi L, Turkki P, Huynh N, et al., 2021, Surface Modification of Bioactive Glass Promotes Cell Attachment and Spreading. ACS Omega, 6:22635–42. https://doi.org/10.1021/acsomega.1c02669
44. Vissers CA, Harvestine JN, Leach JK, 2015, Pore Size Regulates Mesenchymal Stem Cell Response to Bioglass-Loaded Composite Scaffolds. J Mater Chem B, 3:8650–8. https://doi.org/10.1039/c5tb00947b
45. Aguirre A, González A, Navarro M, et al., 2012, Control of Microenvironmental Cues with a Smart Biomaterial Composite Promotes Endothelial Progenitor Cell Angiogenesis. Eur Cells Mater, 24:90–106. https://doi.org/10.22203/eCM.v024a07
46. Kulterer B, Friedl G, Jandrositz A, et al., 2007, Gene Expression Profiling of Human Mesenchymal Stem Cells Derived from Bone Marrow During Expansion and Osteoblast Differentiation. BMC Genomics, 8:1–15. https://doi.org/10.1186/1471-2164-8-70
47. Schroeder TM, Jensen ED, Westendorf JJ, 2005, Runx2: A Master Organizer of Gene Transcription in Developing and Maturing Osteoblasts. Birth Defects Res Part C Embryo Today Rev, 75:213–25. https://doi.org/10.1002/bdrc.20043
48. Li X, Yi W, Jin A, et al., 2015, Effects of Sequentially Released BMP-2 and BMP-7 from PELA Microcapsule-Based Scaffolds on the Bone Regeneration. Am J Transl Res, 7:1417–28.
49. Bouyer M, Guillot R, Lavaud J, et al., 2016, Surface Delivery of Tunable Doses of Bmp-2 from an Adaptable Polymeric Scaffold Induces Volumetric Bone Regeneration. Biomaterials, 104:168–81. https://doi.org/10.1016/j.biomaterials.2016.06.001
50. Yan H, Wu M, Yuan Y, et al., 2014, Priming of Toll-like Receptor 4 Pathway In Mesenchymal Stem Cells Increases Expression of B Cell Activating Factor. Biochem Biophys Res Commun, 448:212–17. https://doi.org/10.1016/j.bbrc.2014.04.097
51. Dorronsoro A, Lang V, Ferrin I, et al., 2020, Intracellular Role of IL-6 in Mesenchymal Stromal Cell Immunosuppression and Proliferation. Sci Rep, 10:1–12. https://doi.org/10.1038/s41598-020-78864-4
52. Pricola KL, Kuhn NZ, Haleem-Smith H, et al., 2009, Interleukin-6 Maintains Bone Marrow-Derived Mesenchymal Stem Cell Stemness by an ERK1/2-Dependent Mechanism. J Cell Biochem, 108:577–88. https://doi.org/10.1002/jcb.22289
53. Semba T, Sammons R, Wang X, et al., 2020, Concise Review: JNK Signaling in Stem Cell Self-Renewal and Differentiation. Int J Mol Sci, 21:1–19. https://doi.org/10.3390/ijms21072613
54. Yue C, Guo Z, Luo Y, et al., 2020, C-Jun Overexpression Accelerates Wound Healing in Diabetic Rats by Human Umbilical Cord-Derived Mesenchymal Stem Cells. Stem Cells Int, 2020:7430968. https://doi.org/10.1155/2020/7430968
55. Cook GW, Benton MG, Akerley W, et al., 2020, Structural Variation and its Potential Impact on Genome Instability: Novel Discoveries in the EGFR Landscape by Long-Read Sequencing. PLoS One, 15:e0226340. https://doi.org/10.1371/journal.pone.0226340
56. Deng W, Chen H, Su H, et al., 2020, IL6 Receptor Facilitates Adipogenesis Differentiation of Human Mesenchymal Stem Cells Through Activating P38 Pathway. Int J Stem Cells, 13:142–50. https://doi.org/10.15283/ijsc19073
57. Anderson JM, Rodriguez A, Chang DT, 2008, Foreign Body Reaction to Biomaterials. Semin Immunol, 20:86–100. https://doi.org/10.1016/j.smim.2007.11.004
58. Henrich D, Seebach C, Nau C, et al., 2016, Establishment and Characterization of the Masquelet Induced Membrane Technique in a Rat Femur Critical-Sized Defect Model. J Tissue Eng Regen Med, 10:E382–96. https://doi.org/10.1002/term.1826
59. Al-Maawi S, Wang X, Sader R, et al., 2021, Multinucleated Giant Cells Induced by a Silk Fibroin Construct Express Proinflammatory Agents: An Immunohistological Study. Materials (Basel), 14:1–19. https://doi.org/10.3390/ma14144038
60. Söhling N, Ondreka M, Kontradowitz K, et al., 2022, Early Immune Response in Foreign Body Reaction is Im-Plant/Material Specific. Materials (Basel), 15:2195. https://doi.org/10.3390/ma15062195
61. Persson T, Monsef N, Andersson P, et al., 2003, Expression of the Neutrophil-Activating CXC Chemokine ENA-78/CXCL5 by Human Eosinophils. Clin Exp Allergy, 33:531–7. https://doi.org/10.1046/j.1365-2222.2003.01609.x
62. Lorenzo J, 2016, The Effects of Immune Cell Products (Cytokines and Hematopoietic Cell Growth Factors) on Bone Cells. In: Osteoimmunology. Cambridge, Massachusetts: Academic Press. p143–67. https://doi.org/10.1016/B978-0-12-800571-2.00009-8
63. Harada A, Sekido N, Akahoshi T, et al., 1994, Essential Involvement of Interleukin-8 (IL-8) in Acute Inflammation. J Leukoc Biol, 56:559–64. https://doi.org/10.1002/jlb.56.5.559
64. Hehlgans T, Pfeffer K, 2005, The Intriguing Biology of the Tumour Necrosis Factor/Tumour Necrosis Factor Receptor Superfamily: Players, Rules and the Games. Immunology, 115:1–20. https://doi.org/10.1111/j.1365-2567.2005.02143.x
65. Rossi DL, Vicari AP, Franz-Bacon K, et al., 1997, Identification Through Bioinformatics of Two New Macrophage Proinflammatory Human Chemokines: MIP-3alpha and MIP-3beta. J Immunol, 158:1033–6.