Electrohydrodynamic Jet-Printed Ultrathin Polycaprolactone Scaffolds Mimicking Bruch’s Membrane for Retinal Pigment Epithelial Tissue Engineering
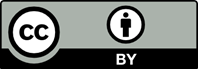
Age-related macular degeneration (AMD) is the leading cause of visual loss and affects millions of people worldwide. Dysfunction of the retinal pigment epithelium (RPE) is associated with the pathogenesis of AMD. The purpose of this work is to build and evaluate the performance of ultrathin scaffolds with an electrohydrodynamic jet (EHDJ) printing method for RPE cell culture. We printed two types of ultrathin (around 7 µm) polycaprolactone scaffolds with 20 μm and 50 μm pores, which possess mechanical properties resembling that of native human Bruch’s membrane and are biodegradable. Light microscopy and cell proliferation assay showed that adult human retinal pigment epithelial (ARPE-19) cells adhered and proliferated to form a monolayer on the scaffolds. The progress of culture matured on the scaffolds was demonstrated by immunofluorescence (actin, ZO-1, and Na+ /K+ -ATPase) and Western blot analysis of the respective proteins. The RPE cells cultured on EHDJ-printed scaffolds with 20 μm pores presented higher permeability, higher transepithelial potential difference, and higher expression level of Na+ /K+ -ATPase than those cultured on Transwell inserts. These findings suggest that the EHDJ printing can fabricate scaffolds that mimic Bruch’s membrane by promoting maturation of RPE cells to form a polarized and functional monolayered epithelium with potential as an in vitro model for studying retinal diseases and treatment methods
1. Wong WL, Su X, Li X, et al., 2014, Global Prevalence of Age-Related Macular Degeneration and Disease Burden Projection for 2020 and 2040: A Systematic Review and Meta-Analysis. Lancet Glob Health, 2:e106–16. http://doi.org/10.1016/S2214-109X(13)70145-1
2. Bagewadi S, Parameswaran S, Subramanian K, et al., 2021, Tissue Engineering Approaches Towards the Regeneration of Biomimetic Scaffolds for Age-Related Macular Degeneration. J Mater Chem B, 9:5935–53. http://doi.org/10.1039/D1TB00976A
3. Liu Z, Yu N, Holz FG, et al., 2014, Enhancement of Retinal Pigment Epithelial Culture Characteristics and Subretinal Space Tolerance of Scaffolds with 200 nm Fiber Topography. Biomaterials, 35:2837–50. http://doi.org/10.1016/j.biomaterials.2013.12.069
4. Sharma R, Khristov V, Rising A, et al., 2019, Clinical-Grade Stem Cell-Derived Retinal Pigment Epithelium Patch Rescues Retinal Degeneration in Rodents and Pigs. Sci Transl Med, 11:eaat5580. http://doi.org/10.1126/scitranslmed.aat5580
5. Hunt N C, Hallam D, Chichagova V, et al., 2018, The Application of Biomaterials to Tissue Engineering Neural Retina and Retinal Pigment Epithelium. Adv Healthc Mater, 7:1800226. http://doi.org/10.1002/adhm.201800226
6. Harris TI, Paterson CA, Farjood F, et al., 2019, Utilizing Recombinant Spider Silk Proteins to Develop a Synthetic Bruch’s Membrane for Modeling the Retinal Pigment Epithelium. ACS Biomater Sci Eng, 5:4023-36. http://doi. org/10.1021/acsbiomaterials.9b00183
7. Tan EY, Agarwala S, Yap YL, et al., 2017, Novel Method for the Fabrication of Ultrathin, Free-Standing and Porous Polymer Membranes for Retinal Tissue Engineering. J Mater Chem B, 5:5616–22. http://doi.org/10.1039/C7TB00376E
8. Chan SY, Chan BQ, Liu Z, et al., 2017, Electrospun Pectin-Polyhydroxybutyrate Nanofibers for Retinal Tissue Engineering. ACS Omega, 2:8959–68. http://doi.org/10.1021/acsomega.7b01604
9. Krishna L, Nilawar S, Ponnalagu M, et al., 2020, Fiber Diameter Differentially Regulates Function of Retinal Pigment and Corneal Epithelial Cells on Nanofibrous Tissue Scaffolds. ACS Appl Bio Mater, 3:823–37. http://doi.org/10.1021/acsabm.9b00897
10. Murphy AR, Truong YB, O’Brien CM, et al., 2020, Bio-Inspired Human In Vitro Outer Retinal Models: Bruch’s Membrane and Its Cellular Interactions. Acta Biomater, 104:1–16. http://doi.org/10.1016/j.actbio.2020.01.013
11. Klingeborn M, Dismuke WM, Skiba NP, et al., 2017, Directional Exosome Proteomes Reflect Polarity-Specific Functions in Retinal Pigmented Epithelium Monolayers. Sci Rep, 7:4901. http://doi.org/10.1038/s41598-017-05102-9
12. Apel P, 2001, Track Etching Technique in Membrane Technology. Radiat Meas, 34:559–66. https://doi.org/10.1016/S1350-4487(01)00228-1
13. Kim MY, Li DJ, Pham LK, et al., 2014, Microfabrication of High-Resolution Porous Membranes for Cell Culture. J Memb Sci, 452:460–9. https://doi.org/10.1016/j.memsci.2013.11.034
14. Liu Z, Parikh BH, Tan QS, et al., 2021, Surgical Transplantation of Human RPE Stem Cell-Derived RPE Monolayers into Non-Human Primates with Immunosuppression. Stem Cell Rep, 16:237–51. http://doi.org/10.1016/j.stemcr.2020.12.007
15. Liu H, Jing L, Sun J, et al., 2021, An Overview of Scaffolds for Retinal Pigment Epithelium Research. Proc Manuf, 53:492–9. http://doi.org/10.1016/j.promfg.2021.06.051
16. Liu H, Vijayavenkataraman S, Wang D, et al., 2017, Influence of Electrohydrodynamic Jetting Parameters on the Morphology of PCL Scaffolds. Int J Bioprint, 3:009. http://doi.org/10.18063/IJB.2017.01.009
17. Jing L, Sun M, Xu P, et al., 2021, Non-invasive In Vivo Imaging and Monitoring of 3D-Printed Polycaprolactone Scaffolds Labeled with an NIR Region II Fluorescent Dye. ACS Appl Bio Mater, 4:3189–202. http://doi.org/10.1021/acsabm.0c01587
18. He J, Zhang B, Li Z, et al., 2020, High-Resolution Electrohydrodynamic Bioprinting: A New Biofabrication Strategy for Biomimetic Micro/Nanoscale Architectures and Living Tissue Constructs. Biofabrication, 12:042002. http://doi.org/10.1088/1758-5090/aba1fa
19. Onses MS, Sutanto E, Ferreira P M, et al., 2015, Mechanisms, Capabilities, and Applications of High-Resolution Electrohydrodynamic Jet Printing. Small, 11:4237–66. http://doi.org/10.1002/smll.201500593
20. Wu B, Takeshita N, Wu Y, et al., 2018, Pluronic F127 Blended Polycaprolactone Scaffolds Via E-Jetting for Esophageal Tissue Engineering. J Mater Sci Mater Med, 29:140. http://doi.org/10.1007/s10856-018-6148-z
21. Rashid A, Bhatia SK, Mazzitello KI, et al., 2016, RPE Cell and Sheet Properties in Normal and Diseased Eyes. Adv Exp Med Biol, 854:757–63. http://doi.org/10.1007/978-3-319-17121-0_101
22. Zhang B, He J, Lei Q, et al., 2020, Electrohydrodynamic Printing of Sub-Microscale Fibrous Architectures with Improved Cell Adhesion Capacity. Virtual Phys Prototyp, 15:62–74. http://doi.org/10.1080/17452759.2019.1662991
23. Ramachandran P, Varoquaux G, 2012, Mayavi2: 3D Scientific Data Visualization and Plottin. In: Astrophysics Source Code Library, ASCL No. 1205.1008.
24. Wu Y, Fuh JY, Wong YS, et al., 2015, Fabrication of 3D Scaffolds Via E-Jet Printing for Tendon Tissue Repair. Vol. 56833. In: International Manufacturing Science and Engineering Conference, American Society of Mechanical Engineers, p. V002T03A005. https://doi.org/10.1115/MSEC2015-9367
25. Transwell Permeable Supports, Corning Incorporated. Available from: https://www.corning.com/catalog/cls/documents/protocols/Transwell_InstructionManual.pdf [Last accessed on 2021 Oct 30].
26. Chan W, Hussain A, Marshall J, 2007, Youngs Modulus of Bruchs Membrane: implications for AMD. Invest Ophthalmol Vis Sci, 48:2187–7.
27. Mondrinos MJ, Yi YS, Wu NK, et al., 2017, Native Extracellular Matrix-Derived Semipermeable, Optically Transparent, and Inexpensive Membrane Inserts for Microfluidic Cell Culture. Lab Chip, 17:3146–58. http://doi.org/10.1039/C7LC00317J
28. Kaas JH, editor, 2020, Comparative Anatomy of Glial Cells in Mammals. In: Evolutionary Neuroscience. 2nd ed. Academic Press, London, p. 397–439.
29. Pitkänen L, Ranta VP, Moilanen H, et al., 2005, Permeability of Retinal Pigment Epithelium: Effects of Permeant Molecular Weight and Lipophilicity. Invest Ophthalmol Vis Sci, 46:641–6. http://doi.org/10.1167/iovs.04-1051
30. da Cruz L, Fynes K, Georgiadis O, et al., 2018, Phase 1 Clinical Study of an Embryonic Stem Cell-Derived Retinal Pigment Epithelium Patch in Age-Related Macular Degeneration. Nat Biotechnol, 36:328–37. http://doi.org/10.1038/nbt.4114
31. Ablonczy Z, Dahrouj M, Tang PH, et al., 2011, Human Retinal Pigment Epithelium Cells as Functional Models for the RPE In Vivo. Invest Ophthalmol Vis Sci, 52:8614–20. http://doi.org/10.1167/iovs.11-8021
32. Wetzel RK, Sweadner KJ, 2001, Immunocytochemical Localization of NaK-ATPase Isoforms in the Rat and Mouse Ocular Ciliary Epithelium. Invest Ophthalmol Vis Sci, 42:763–9.
33. Cao L, Liu J, Pu J, et al., 2018, Polarized Retinal Pigment Epithelium Generates Electrical Signals that Diminish with Age and Regulate Retinal Pathology. J Cell Mol Med, 22:5552–64. http://doi.org/10.1111/jcmm.13829