Preparation of Graphene Oxide-loaded Nickel with Excellent Antibacterial Property by Magnetic Field-Assisted Scanning Jet Electrodeposition
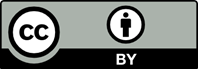
: Graphene oxide (GO) is recognized as a promising antibacterial material that is expected to be used to prepare a new generation of high-efficiency antibacterial coatings. The propensity of GO to agglomeration makes it difficult to apply it effectively. Anew method of preparing GO-loaded nickel (GNC) with excellent antibacterial property is proposed in this paper. In this work, GNC was prepared on a titanium sheet by magnetic field-assisted scanning jet electrodeposition. The massive introduction of GO on the coating was proven by energy disperse spectroscopy and Raman spectroscopy. The antibacterial performance of GNC was proven by agar plate assessment and cell living/dead staining. The detection of intracellular reactive oxygen species (ROS) and the concentration of nickel ions, indicate that the antibacterial property of GNC are not entirely derived from the nickel ions released by the coating and the intracellular ROS induced by nickel ions, but rather are due to the synergistic effect of nickel ions and GO.
1. Simoes M, Simoes LC, Vieira MJ, 2010, A Review of Current and Emergent Biofilm Control Strategies. Lwt Food Sci Technol, 43:573–83. https://doi.org/10.1016/j.lwt.2009.12.008
2. Pistone A, Scolaro C, Visco A, 2021, Mechanical Properties of Protective Coatings against Marine Fouling: A Review. Polymers (Basel), 13:173. https://doi.org/10.3390/polym13020173
3. Angell P, Urbanic K, 2000, Sulphate-reducing Bacterial Activity as a Parameter to Predict Localized Corrosion of Stainless Alloys. Corro Sci, 42:897–912. https://doi.org/10.1016/s0010-938x(99)00116-x
4. Veerachamy S, Yarlagadda T, Manivasagam G, et al., 2014, Bacterial Adherence and Biofilm Formation on Medical Implants: A Review. Proc Inst Mech Eng Part H, 228:1083–99. https://doi.org/10.1177/0954411914556137
5. Cloutier M, Mantovani D, Rosei F, 2015, Antibacterial Coatings: Challenges, Perspectives, and Opportunities. Trends Biotechnol, 33:637–52. https://doi.org/10.1016/j.tibtech.2015.09.002
6. Bandara CD, Singh S, Afara IO, et al., 2017, Bactericidal Effects of Natural Nanotopography of Dragonfly Wing on Escherichia coli. ACS Appl Mater Interfaces, 9:6746–60. https://doi.org/10.1021/acsami.6b13666
7. Ivanova EP, Hasan J, Webb HK, et al., 2012, Natural Bactericidal Surfaces: Mechanical Rupture of Pseudomonas aeruginosa Cells by Cicada Wings. Small, 8:2489–94. https://doi.org/10.1002/smll.201200528
8. Kazemzadeh-Narbat M, Lai BF, Ding C, et al., 2013, Multilayered Coating on Titanium for Controlled Release of Antimicrobial Peptides for the Prevention of Implant associated Infections. Biomaterials, 34:5969–77. https://doi.org/10.1016/j.biomaterials.2013.04.036
9. Park S, Kim HH, Bin Yang S, et al., 2018, A Polysaccharide-Based Antibacterial Coating with Improved Durability for Clear Overlay Appliances. ACS Appl Mater Interfaces, 10:17714–21. https://doi.org/10.1021/acsami.8b04433
10. Peng L, Chang L, Liu X, et al., 2017, Antibacterial Property of a Polyethylene Glycol-Grafted Dental Material. ACS Appl Mater Interfaces, 9:17688–692. https://doi.org/10.1021/acsami.7b05284
11. Zhao Q, Liu C, Su X, et al., 2013, Antibacterial Characteristics of Electroless Plating Ni-P-TiO2 Coatings. Appl Surf Sci, 274:101–4. https://doi.org/10.1016/j.apsusc.2013.02.112
12. Dreyer DR, Park S, Bielawski CW, et al., 2010, The Chemistry of Graphene Oxide. Chem Soc Rev, 39:228–40. https://doi.org/10.1039/b917103g
13. Baek SH, Roh J, Park CY, et al., 2020, Cu-nanoflower Decorated Gold Nanoparticles-graphene Oxide Nanofiber as Electrochemical Biosensor for Glucose Detection. Mater Sci Eng C Mater, 107:110273. https://doi.org/10.1016/j.msec.2019.110273
14. Singh V, Kumar V, Kashyap S, et al., 2019, Graphene Oxide Synergistically Enhances Antibiotic Efficacy in Vancomycin-Resistant Staphylococcus aureus. ACS Appl Bio Mater, 2:1148–57. https://doi.org/10.1021/acsabm.8b00757
15. Kuila T, Mishra AK, Khanra P, et al., 2013, Recent Advances in the Efficient Reduction of Graphene Oxide and its Application as Energy Storage Electrode Materials. Nanoscale, 5:52–71. https://doi.org/10.1039/c2nr32703a
16. Shih CJ, Lin S, Sharma R, et al., 2013, Understanding the pH dependent Behavior of Graphene Oxide Aqueous Solutions: A Comparative Experimental and Molecular Dynamics Simulation Study. Langmuir, 28:235–41.
17. Hu W, Peng C, Luo W, et al., 2010, Graphene-Based Antibacterial Paper, ACS Nano 4:4317–23. https://doi.org/10.1021/nn101097v.
18. Akhavan O, Ghaderi E, 2010, Toxicity of Graphene and Graphene Oxide Nano walls Against Bacteria. ACS Nano, 4:5731–6. https://doi.org/10.1021/nn101390x
19. Ahmed F, Rodrigues DF, 2013, Investigation of Acute Effects of Graphene Oxide on Wastewater Microbial Community: A Case Study. J Hazard Mater, 256:33–9. https://doi.org/10.1016/j.jhazmat.2013.03.064
20. Carpio IE, Santos CM, Wei X, et al., 2012, Toxicity of a Polymer-graphene Oxide Composite Against Bacterial Planktonic Cells, Biofilms, and Mammalian Cells. Nanoscale, 4:4746–56. https://doi.org/10.1039/c2nr30774j
21. Zou X, Zhang L, Wang Z, et al., 2016, Mechanisms of the Antimicrobial Activities of Graphene Materials. J Am Chem Soc, 138:2064–77. https://doi.org/10.1021/jacs.5b11411
22. Tu Y, Lv M, Xiu P, et al., 2013, Destructive Extraction of Phospholipids from Escherichia coli Membranes by Graphene Nanosheets. Nat Nanotechnol, 8:594–601. https://doi.org/10.1038/nnano.2013.125
23. Konwar A, Kalita S, Kotoky J, et al., 2016, Chitosan-Iron Oxide Coated Graphene Oxide Nanocomposite Hydrogel: A Robust and Soft Antimicrobial Biofilm. ACS Appl Mater Interfaces, 8:20625–34. https://doi.org/10.1021/acsami.6b07510
24. Liu Y, Wen J, Gao Y, et al., 2018, Antibacterial Graphene Oxide Coatings on Polymer Substrate. Appl Surf Sci, 436:624–30. https://doi.org/10.1016/j.apsusc.2017.12.006
25. Zhao Z, Meng F, Tang J, et al., 2019, A Novel Method of Fabricating an Antibacterial Aluminum-matrix Composite Coating Doped Graphene/Silver-nanoparticles. Mater Lett, 245:211–4. https://doi.org/10.1016/j.matlet.2019.02.121
26. Goh GL, Zhang H, Chong TH, et al., 2021, 3D Printing of Multilayered and Multimaterial Electronics: A Review. Adv Electron Mater, 2021:2100445. https://doi.org/10.1002/aelm.202100445
27. Mahmood MA, Banica A, Ristoscu C, et al., 2021, Laser Coatings via State-of-the-Art Additive Manufacturing: A Review. Coatings, 11:296. https://doi.org/10.3390/coatings11030296
28. Saengchairat N, Tran T, Chua CK, 2017, A Review: Additive Manufacturing for Active Electronic Components. Virtual Phys Prototype, 12:31–46. https://doi.org/10.1080/17452759.2016.1253181
29. Shuai CJ, Yang ML, Deng F, et al., 2020, Forming Quality, Mechanical Properties, and Anti-inflammatory Activity of Additive Manufactured Zn-Nd Alloy. J Zhejiang Univ A, 21:876–91. https://doi.org/10.1631/jzus.A2000186
30. Veres J, Bringans RD, Chow EM, et al., 2016, Additive Manufacturing for Electronics “Beyond Moore”. San Francisco, CA, USA: Proceedings of 2016 IEEE International Electron Devices Meeting, p25-6.
31. Choe YE, Kim GH, 2020, A PCL/Cellulose Coil-shaped Scaffold via a Modified Electrohydrodynamic Jetting Process. Virtual Phys Prototype, 15:403–16. https://doi.org/10.1080/17452759.2020.1808269
32. Goh GL, Agarwala S, Yeong WY, 2018, High Resolution Aerosol Jet Printing of Conductive Ink for Stretchable Electronics. Proceedings of the 3rd International Conference on Progress in Additive Manufacturing, p109–14.
33. Goh GL, Tay MF, Lee JM, et al., 2021, Potential of Printed Electrodes for Electrochemical Impedance Spectroscopy (EIS): Toward Membrane Fouling Detection. Adv Electron Mater, 2021:2100043. https://doi.org/10.1002/aelm.202100043
34. Wang Z, Zhang G, Huang H, et al., 2021, The Self-induced Electric-field-driven Jet Printing for Fabricating Ultrafine Silver Grid Transparent Electrode. Virtual Phys Prototy, 16:113–23. https://doi.org/10.1080/17452759.2020.1823116
35. Ning F, Cong W, Hu Y, et al., 2017, Additive Manufacturing of Carbon Fiber-reinforced Plastic Composites Using Fused Deposition Modeling: Effects of Process Parameters on Tensile Properties. J Compos Mater, 51:451–62. https://doi.org/10.1177/0021998316646169
36. Alemohammad H, Toyserkani E, 2010, Laser-assisted Additive Fabrication of Micro-sized Coatings. Adv Laser Mater Process, 2010:735–62.
37. Hirt L, Ihle S, Pan Z, et al., 2016, Template-Free 3D Microprinting of Metals Using a Force-Controlled Nanopipette for Layer-by-Layer Electrodeposition. Adv Mater, 28:2311–5. https://doi.org/10.1002/adma.201504967
38. Jiang W, Shen L, Qiu M, et al., 2018, Preparation of Ni-SiC composite coatings by magnetic field-enhanced jet electrodeposition. J Alloys Compd, 762:115–24. https://doi.org/10.1016/j.jallcom.2018.05.097
39. Qiao GY, Jing TF, Wang N, et al., 2005, High-speed Jet Electrodeposition and Microstructure of Nanocrystalline Ni-Co Alloys. Electrochim Acta, 51:85–92. https://doi.org/10.1016/j.electacta.2005.03.050
40. Shen L, Xu M, Jiang W, et al., 2019, A Novel Superhydrophobic Ni/Nip Coating Fabricated by Magnetic Field Induced Selective Scanning Electrodeposition. Appl Surf Sci, 489:25–33. https://doi.org/10.1016/j.apsusc.2019.05.335
41. Xu M, Shen L, Jiang W, et al., 2019, Fabrication of Ni- SiC Superhydrophilic Surface by Magnetic Field-assisted Scanning Electrodeposition. J Alloys Compd, 799:224–30. https://doi.org/10.1016/j.jallcom.2019.05.339
42. Ji L, Chen F, Huang H, et al., 2018, Preparation of Nickel-Graphene Composites by Jet Electrodeposition and the Influence of Graphene Oxide Concentration on the Morphologies and Properties. Surf Coat Technol, 351:212–9. https://doi.org/10.1016/j.surfcoat.2018.07.083
43. Arghavanian R, Bostani B, Parvini-Ahmadi N, et al., 2014, Field-enhanced Co-electrodeposition of Zirconia Particles with a Magnetic Shell During Ni Electrodeposition. Surf Coat Technol, 258:1171–5. https://doi.org/10.1016/j.surfcoat.2014.07.015
44. Jena G, Anandkumar B, Sofia S, et al., 2020, Fabrication of Silanized GO Hybrid Coating on 316L SS with Enhanced Corrosion Resistance and Antibacterial Properties for Marine Applications. Surf Coat Technol, 402:126295. https://doi.org/10.1016/j.surfcoat.2020.126295
45. Jena G, Vanithakumari SC, Polaki SR, et al., 2019, Electrophoretically Deposited Graphene Oxide-polymer Bilayer Coating on Cu-Ni Alloy with Enhanced Corrosion Resistance in Simulated Chloride Environment. J Coat Technol Res, 16:1317–35. https://doi.org/10.1007/s11998-019-00213-6
46. Gautier N, 1995, Theoretical Study of the Interaction between a Magnetic Nanotip and a Magnetic Surface. Phys Rev B, 52:7352–62. https://doi.org/10.1103/PhysRevB.52.7352
47. Stone HA, Lister JR, Brenner MP, 1999, Drops with Conical Ends in Electric and Magnetic Fields. Proc R Soc A Math Phys Eng Sci, 455:329–47. https://doi.org/10.1098/rspa.1999.0316
48. Pavithra CL, Sarada BV, Rajulapati KV, et al., 2014, A New Electrochemical Approach for the Synthesis of Copper-Graphene Nanocomposite Foils with High Hardness. Sci Rep, 4:4049. https://doi.org/10.1038/srep04049
49. Qiu J, Liu L, Qian S, et al., 2021, Why does Nitrogen-doped Graphene Oxide Lose the Antibacterial Activity? J Mater Sci Technol, 62:44–51. https://doi.org/10.1016/j.jmst.2020.05.051
50. Shuai C, Guo W, Gao C, et al., 2018, An nMgO Containing Scaffold: Antibacterial Activity, Degradation Properties and Cell Responses. Int J Bioprint, 4:120. https://doi.org/10.18063/IJB.v4i1.120
51. Xie B, Zhao MC, Xu R, et al., 2021, Biodegradation, Antibacterial Performance, and Cytocompatibility of a Novel ZK30-Cu-Mn Biomedical Alloy Produced by Selective Laser Melting. Int J Bioprint, 7:78–89. https://doi.org/10.18063/ijb.v7i1.300
52. Park HJ, Kim JY, Kim J, et al., 2009, Silver-ion-mediated Reactive Oxygen Species Generation Affecting Bactericidal Activity. Water Res, 43:1027–32. https://doi.org/10.1016/j.watres.2008.12.002
53. Chernousova S, Epple M, 2013, Silver as Antibacterial Agent: Ion, Nanoparticle, and Metal. Angew Chemie Int Ed, 52:1636–53. https://doi.org/10.1002/anie.201205923
54. Kedziora A, Speruda M, Krzyzewska E, et al., 2018, Similarities and Differences between Silver Ions and Silver in Nanoforms as Antibacterial Agents. Int J Mol Sci, 19:444. https://doi.org/10.3390/ijms19020444
55. Lemire JA, Harrison JJ, Turner RJ, 2013, Antimicrobial Activity of Metals: Mechanisms, Molecular Targets and Applications. Nat Rev Microbiol, 11:371–84. https://doi.org/10.1038/nrmicro3028
56. Perreault F, de Faria AF, Nejati S, et al., 2015, Antimicrobial Properties of Graphene Oxide Nanosheets: Why Size Matters. ACS Nano, 9:7226–36. https://doi.org/10.1021/acsnano.5b02067
57. Chung H, Kim MJ, Ko K, et al., 2015, Effects of Graphene Oxides on Soil Enzyme Activity and Microbial Biomass. Sci Total Environ, 514:307–13. https://doi.org/10.1016/j.scitotenv.2015.01.077
58. Gurunathan S, Han JW, Dayem AA, et al., 2013, Antibacterial Activity of Dithiothreitol Reduced Graphene Oxide. J Ind Eng Chem, 19:1280–8. https://doi.org/10.1016/j.jiec.2012.12.029
59. Das KK, Das SN, Dhundasi SA, 2008, Nickel, its Adverse Health Effects and Oxidative. Indian J Med Res, 128:412–25.
60. Abdel-Rahman LH, Abu-Dief AM, Moustafa H, et al., 2020, Design and Nonlinear Optical Properties (NLO) Using DFT Approach of New Cr(III), VO(II), and Ni(II) Chelates Incorporating Tri-dentate Imine Ligand for DNA Interaction, Antimicrobial, Anticancer Activities and Molecular Docking Studies. Arab J Chem, 13:649–70. https://doi.org/10.1016/j.arabjc.2017.07.007
61. Ohtste N, Hirano Y, Yamaguchi K, et al., 2019, Surface Characteristics, Ni ion Release, and Antibacterial Efficacy of Anodized NiTi Alloy Using HNO3 Electrolyte of Various Concentrations. Appl Surf Sci, 492:785–91. https://doi.org/10.1016/j.apsusc.2019.06.243
62. Vanithakumari SC, Jena G, Sofia S, et al., 2020, Fabrication of Superhydrophobic Titanium Surfaces with Superior Antibacterial Properties Using Graphene Oxide and Silanized Silica Nanoparticles. Surf Coat Technol, 400:126074. https://doi.org/10.1016/j.surfcoat.2020.126074
63. Liu S, Zeng TH, Hofmann M, et al., 2011, Antibacterial Activity of Graphite, Graphite Oxide, Graphene Oxide, and Reduced Graphene Oxide: Membrane and Oxidative Stress. ACS Nano, 5:6971–80. https://doi.org/10.1021/nn202451x
64. Salas E C, Sun Z, Luttge A, et al., 2010, Reduction of Graphene Oxide via Bacterial Respiration. ACS Nano, 4:4852–6. https://doi.org/10.1021/nn101081t
65. Panda S, Rout TK, Prusty AD, et al., 2018, Electron Transfer Directed Antibacterial Properties of Graphene Oxide on Metals. Adv Mater, 30:1702149. https://doi.org/10.1002/adma.201702149
66. Qiu J, Wang D, Geng H, et al., 2017, How Oxygen-Containing Groups on Graphene Influence the Antibacterial Behaviors. Adv Mater Interfaces, 4:1700228. https://doi.org/10.1002/admi.201700228
67. Pieper H, Chercheja S, Eigler S, et al., 2016, Endoperoxides Revealed as Origin of the Toxicity of Graphene Oxide. Angew Chem Int Edi, 55:405–7. https://doi.org/10.1002/anie.201507070
68. Zhao J, Wang Z, White JC, et al., 2014, Graphene in the Aquatic Environment: Adsorption, Dispersion, Toxicity and Transformation. Environ Sci Technol, 48:9995–10009. https://doi.org/10.1021/es5022679
69. Wang G, Feng H, Gao A, et al., 2016, Extracellular Electron Transfer from Aerobic Bacteria to Au-Loaded TiO2 Semiconductor without Light: A New Bacteria-Killing Mechanism Other than Localized Surface Plasmon Resonance or Microbial Fuel Cells. ACS Appl Mater Interfaces, 8:24509–16. https://doi.org/10.1021/acsami.6b10052
70. Hassan RY, Wollenberger U, 2016, Mediated Bioelectrochemical System for Biosensing the Cell Viability of Staphylococcus aureus. Ana Bioanal Chem, 408:579–87. https://doi.org/10.1007/s00216-015-9134-z