Bio-assembling and Bioprinting for Engineering Microvessels from the Bottom Up
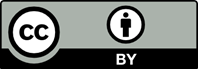
Blood vessels are essential in transporting nutrients, oxygen, metabolic wastes, and maintaining the homeostasis of the whole human body. Mass of engineered microvessels is required to deliver nutrients to the cells included in the constructed large three-dimensional (3D) functional tissues by diffusion. It is a formidable challenge to regenerate microvessels and build a microvascular network, mimicking the cellular viabilities and activities in the engineered organs with traditional or existing manufacturing techniques. Modular tissue engineering adopting the “bottom-up” approach builds one-dimensional (1D) or two-dimensional (2D) modular tissues in micro scale first and then uses these modules as building blocks to generate large tissues and organs with complex but indispensable microstructural features. Building the microvascular network utilizing this approach could be appropriate and adequate. In this review, we introduced existing methods using the “bottom-up” concept developed to fabricate microvessels including bio-assembling powered by different micromanipulation techniques and bioprinting utilizing varied solidification mechanisms. We compared and discussed the features of the artificial microvessels engineered by these two strategies from multiple aspects. Regarding the future development of engineering the microvessels from the bottom up, potential directions were also concluded.
1. Hillsley MV, Frangos JA, 1994, Bone Tissue Engineering: The Role of Interstitial Fluid Flow. Biotechnol Bioeng, 43:573–81. http://doi.org/10.1002/bit.260430706
2. Martin P, 1997, Wound Healing--Aiming for Perfect Skin Regeneration. Science, 276:75–81. http://doi.org/10.1126/science.276.5309.75
3. Bello YM, Falabella AF, Eaglstein WH, 2001, Tissue-Engineered Skin. Current Status in Wound Healing. Am J Clin Dermatol, 2:305–13. http://doi.org/10.2165/00128071-200102050-00005
4. Langer R, Vacanti JP, 1993, Tissue Engineering. Science, 260:920–6. http://doi.org/10.1126/science.8493529
5. Stock UA, Vacanti JP, 2001, Tissue Engineering: Current State and Prospects. Annu Rev Med, 52:443–51. http://doi.org/10.1146/annurev.med.52.1.443
6. Vacanti CA, 2006, The History of Tissue Engineering. J Cell Mol Med, 10:569–76. http://doi.org/10.1111/j.1582-4934.2006.tb00421.x
7. Aikawa E, Nahrendorf M, Sosnovik D, et al., 2007, Multimodality Molecular Imaging Identifies Proteolytic and Osteogenic Activities in Early Aortic Valve Disease. Circulation, 115:377–86. http://doi.org/10.1161/circulationaha.106.654913
8. Walther G, Gekas J, Bertrand OF, 2009, Amniotic Stem Cells for Cellular Cardiomyoplasty: Promises and Premises. Catheter Cardiovasc Interv, 73:917–24. http://doi.org/10.1002/ccd.22016
9. Wang X, Yan, Y, Zhang R, 2010, Gelatin-Based Hydrogels for Controlled Cell Assembly. New York: Springer.
10. Wang X, 2012, Intelligent Freeform Manufacturing of Complex Organs. Artif Organs, 36:951–61. http://doi.org/10.1111/j.1525-1594.2012.01499.x
11. Orive G, Hernández RM, Rodríguez Gascón A, et al., 2004, History, Challenges and Perspectives of Cell Microencapsulation. Trends Biotechnol, 22:87–92. http://doi.org/10.1016/j.tibtech.2003.11.004
12. Khademhosseini A, Langer R, Borenstein J, et al., 2006, Microscale Technologies for Tissue Engineering and Biology. Proc Natl Acad Sci U S A, 103:2480–7. http://doi.org/10.1073/pnas.0507681102
13. Chung BG, Kang L, Khademhosseini A, 2007, Micro-and Nanoscale Technologies for Tissue Engineering and Drug Discovery Applications. Expert Opin Drug Discov, 2:1653–68. http://doi.org/10.1517/17460441.2.12.1653
14. Miller JS, Stevens KR, Yang MT, et al., 2012, Rapid Casting of Patterned Vascular Networks for Perfusable Engineered Three-dimensional Tissues. Nat Mater, 11:768–74. http://doi.org/10.1038/nmat3357
15. Yue T, Zhao D, Phan DT, et al., 2021, A Modular Microfluidic System Based on a Multilayered Configuration to Generate Large-Scale Perfusable Microvascular Networks. Microsyst Nanoeng, 7:4. http://doi.org/10.1038/s41378-020-00229-8
16. Niklason LE, Gao J, Abbott WM, et al., 1999, Functional Arteries Grown In Vitro. Science, 284:489–93. http://doi.org/10.1126/science.284.5413.489
17. Gooch KJ, Blunk T, Courter DL, et al., 2002, Bone Morphogenetic Proteins-2, -12, and -13 Modulate In Vitro Development of Engineered Cartilage. Tissue Eng, 8:591–601. http://doi.org/10.1089/107632702760240517
18. Tranquillo RT, 2002, The Tissue-engineered Small-diameter Artery. Ann N Y Acad Sci, 961:251–4. http://doi.org/10.1111/j.1749-6632.2002.tb03094.x
19. Boublik J, Park H, Radisic M, et al., 2005, Mechanical Properties and Remodeling of Hybrid Cardiac Constructs Made From Heart Cells, Fibrin, and Biodegradable, Elastomeric Knitted Fabric. Tissue Eng, 11:1122–32. http://doi.org/10.1089/ten.2005.11.1122
20. Saito J, Kaneko M, Ishikawa Y, et al., 2021, Challenges and Possibilities of Cell-Based Tissue-Engineered Vascular Grafts. Cyborg Bionic Syst, 2021:1532103. http://doi.org/10.34133/2021/1532103.
21. Ng HY, Lee KA, Kuo CN, et al., 2018, Bioprinting of Artificial Blood Vessels. Int J Bioprint, 4:140. http://doi.org/10.18063/IJB.v4i2.140
22. Arai T, Arai F, Yamato M, 2015, Hyper Bio Assembler for 3D Cellular Systems. Japan: Springer. https://doi.org/10.1007/978-4-431-55297-0
23. Yang J, Yamato M, Sekine H, et al., 2009, Tissue Engineering Using Laminar Cellular Assemblies. Adv Mater, 21:3404–9. http://doi.org/10.1002/adma.200801990
24. Kinstlinger IS, Saxton SH, Calderon GA, et al., 2020, Generation of Model Tissues with Dendritic Vascular Networks via Sacrificial Laser-sintered Carbohydrate Templates. Nat Biomed Eng, 2020:1–17. https://doi.org/10.1038/s41551-020-0566-1
25. Bertassoni LE, Cecconi M, Manoharan V, et al., 2014, Hydrogel Bioprinted Microchannel Networks for Vascularization of Tissue Engineering Constructs. Lab A Chip, 14:2202–11. https://doi.org/10.1039/c4lc00030g
26. Matsunaga YT, Morimoto Y, Takeuchi S, 2011, Molding Cell Beads for Rapid Construction of Macroscopic 3D Tissue Architecture. Adv Mater, 23:H90–4. http://doi.org/10.1002/adma.201004375
27. Tan A, Fujisawa K, Yukawa Y, et al., 2016, Bottom-up Fabrication of Artery-Mimicking Tubular Co-cultures in Collagen-based Microchannel Scaffolds. Biomater Sci, 4:1503–14. http://doi.org/10.1039/c6bm00340k
28. Nichol JW, Khademhosseini A, 2009, Modular Tissue Engineering: Engineering Biological Tissues from the Bottom Up. Soft Matter, 5:1312–9. http://doi.org/10.1039/b814285h
29. Gurkan UA, Tasoglu S, Kavaz D, et al., 2012, Emerging Technologies for Assembly of Microscale Hydrogels. Adv Healthc Mater, 1:149–58. https://doi.org/10.1002/adhm.201200011
30. Onoe H, Okitsu T, Itou A, et al., 2013, Metre-long Cell-laden Microfibres Exhibit Tissue Morphologies and Functions. Nat Mater, 12:584–90. https://doi.org/10.1038/nmat3606
31. Connon CJ, 2015, Approaches to Corneal Tissue Engineering: Top-down or Bottom-up? Proc Eng, 110:15–20. https://doi.org/10.1016/j.proeng.2015.07.004
32. Bova L, Billi F, Cimetta E, 2020, Mini-review: Advances in 3D Bioprinting of Vascularized Constructs. Biol Direct, 15:22. http://doi.org/10.1186/s13062-020-00273-4
33. Mironov V, Visconti RP, Kasyanov V, et al., 2009, Organ Printing: Tissue Spheroids as Building Blocks. Biomaterials, 30:2164–74. http://doi.org/10.1016/j.biomaterials.2008.12.084
34. Fennema E, Rivron N, Rouwkema J, et al., 2013, Spheroid Culture as a Tool for Creating 3D Complex Tissues. Trends Biotechnol, 31:108–15. https://doi.org/10.1016/j.tibtech.2012.12.003
35. Rivron NC, Vrij EJ, Rouwkema J, et al., 2012, Tissue Deformation Spatially Modulates VEGF Signaling and Angiogenesis. Proc Natl Acad Sci, 109:6886–91. https://doi.org/10.1073/pnas.1201626109
36. Torisawa YS, Chueh BH, Huh D, et al., 2007. Efficient Formation of Uniform-sized Embryoid Bodies Using a Compartmentalized Microchannel Device. Lab Chip, 7:770–6. https://doi.org/10.1039/B618439A.
37. Tamayol A, Akbari M, Annabi N, et al., 2013, Fiber-based Tissue Engineering: Progress, Challenges, and Opportunities. Biotechnol Adv, 31:669–87. https://doi.org/10.1016/j.biotechadv.2012.11.007
38. Teshima T, Onoe H, Kuribayashiashiashias K, et al., 2014, Parylene Mobile Microplates Integrated with an Enzymatic Release for Handling of Single Adherent Cells. Small, 10:912–21. https://doi.org/10.1002/smll.201301993
39. Kuribayashi-Shigetomi K, Onoe H, Takeuchi S, 2012, Cell Origami: Self-Folding of Three-Dimensional Cell-Laden Microstructures Driven by Cell Traction Force. PLoS One, 7:e51085. https://doi.org/10.1371/journal.pone.0051085
40. Yue, T, Nakajima M, Tajima H, et al., 2013. Fabrication of Microstructures Embedding Controllable Particles inside Dielectrophoretic Microfluidic Devices. Int J Adv Robot Syst, 10:132–40. https://doi.org/10.5772/55598
41. Gach PC, Wang Y, Phillips C, et al., 2011, Isolation and Manipulation of Living Adherent Cells by Micromolded Magnetic Rafts. Biomicrofluidics, 5:32002–12. https://doi.org/10.1063/1.3608133
42. Liu Z, Takeuchi M, Nakajima M, et al., 2016, Batch Fabrication of Microscale Gear-Like Tissue by Alginate-Poly-L-lysine (PLL) Microcapsules System. IEEE Robot Autom Lett, 1:206–12. https://doi.org/10.1109/lra.2016.2514500
43. Yokoyama U, Tonooka Y, Koretake R, et al., 2017, Arterial Graft with Elastic Layer Structure Grown From Cells. Sci Rep, 7:140–55. https://doi.org/10.1038/s41598-017-00237-1
44. Hinds MT, Rowe RC, Ren Z, et al., 2006, Development of a Reinforced Porcine Elastin Composite Vascular Scaffold. J Biomed Mater Res Part A, 77A:458–69. https://doi.org/10.1002/jbm.a.30571
45. Rutz AL, Lewis PL, Shah RN, 2017, Toward Next-generation Bioinks: Tuning Material Properties Pre-and Post-printing to Optimize Cell Viability. MRS Bull, 42:563–70. https://doi.org/10.1557/mrs.2017.162
46. Mobaraki M, Ghaffari M, Yazdanpanah A, et al., 2020, Bioinks and Bioprinting: A Focused Review. Bioprinting, 18:e00080–95. https://doi.org/10.1016/j.bprint.2020.e00080
47. Jia J, Richards DJ, Pollard S, et al., 2014, Engineering Alginate as Bioink for Bioprinting. Acta Biomater, 10:4323–31. https://doi.org/10.1016/j.actbio.2014.06.034
48. Guvendiren M, Molde J, Soares RM, et al., 2016, Designing Biomaterials for 3D Printing. ACS Biomater Sci Eng, 2:1679–93. https://doi.org/10.1021/acsbiomaterials.6b00121
49. Hospodiuk M, Dey M, Sosnoski D, et al., 2017, The Bioink: A Comprehensive Review on Bioprintable Materials. Biotechnol Adv, 35:217–39. https://doi.org/10.1016/j.biotechadv.2016.12.006
50. Gomez-Guillen MC, Turnay J, Fernandez-Dıaz MD, et al., 2002, Structural and Physical Properties of Gelatin Extracted from Different Marine Species: A Comparative Study. Food Hydrocoll, 16:25–34. https://doi.org/10.1016/S0268-005X(01)00035-2
51. Rowley JA, Madlambayan G, Mooney DJ, 1999, Alginate Hydrogels as Synthetic Extracellular Matrix Materials. Biomaterials, 20:45–53. https://doi.org/10.1016/S0142-9612(98)00107-0
52. Demirtas TT, Irmak G, Gümüs M, et al., 2017, A Bioprintable form of Chitosan Hydrogel for Bone Tissue Engineering. Biofabrication, 9:35003. https://doi.org/10.1088/1758-5090/aa7b1d
53. Loo Y, Hauser CA, 2015, Bioprinting Synthetic Self assembling Peptide Hydrogels for Biomedical Applications. Biomed Mater, 11:14103. https://doi.org/10.1088/1748-6041/11/1/014103
54. Du Y, Lo E, Ali S, et al., 2008, Directed Assembly of Cell laden Microgels for Fabrication of 3D Tissue Constructs. Proc Natl Acad Sci, 105:9522–7. https://doi.org/10.1073/pnas.0801866105
55. Elbert DL, 2011, Bottom-up Tissue Engineering. Curr Opin Biotechnol, 22:674–80. https://doi.org/10.1016/j.copbio.2011.04.001
56. Kasza KE, Rowat AC, Liu J, et al., 2007, The Cell as a Material. Curr Opin Cell Biol, 19:101–7. https://doi.org/10.1016/j.ceb.2006.12.002
57. Avci E, Ohara K, Nguyen CN, et al., 2015, High-Speed Automated Manipulation of Microobjects Using a Two-Fingered Microhand. IEEE Trans Ind Electron, 62:1070–9. https://doi.org/10.1109/TIE.2014.2347004
58. Ramadan AA, Takubo T, Mae Y, et al., 2009, Developmental Process of a Chopstick-Like Hybrid-Structure Two-Fingered Micromanipulator Hand for 3-D Manipulation of Microscopic Objects. IEEE Trans Ind Electron, 56:1121–35. https://doi.org/10.1109/TIE.2008.2008753
59. L’Heureux N, Quet SP, Labbe R, et al., 1998, A Completely Biological Tissue-Engineered Human Blood Vessel. FASEB J, 12:47–56. https://doi.org/10.1096/fasebj.12.1.47
60. Bourget JM, Gauvin R, Larouche D, et al., 2012, Human Fibroblast-derived ECM as a Scaffold for Vascular Tissue Engineering. Biomaterials, 33:9205–13. https://doi.org/10.1016/j.biomaterials.2012.09.015
61. Du Y, Ghodousi M, Qi H, et al., 2011, Sequential Assembly of Cell-laden Hydrogel Constructs to Engineer Vascular-like Microchannels. Biotechnol Bioeng, 108:1693–703. https://doi.org/10.1002/bit.23102
62. Wang H, Huang Q, Shi Q, et al., 2015, Automated Assembly of Vascular-like Microtube with Repetitive Single-step Contact Manipulation. IEEE Trans Biomed Eng, 62:2620–8. https://doi.org/10.1109/TBME.2015.2437952
63. Yue T, Nakajima M, Takeuchi M, et al., 2014, On-chip Self assembly of Cell Embedded Microstructures to Vascular-like Microtubes. Lab Chip, 14:1151. https://doi.org/10.1039/c3lc51134k
64. Yue T, Liu N, Liu Y, et al., 2019, On-Chip Construction of Multilayered Hydrogel Microtubes for Engineered Vascular-Like Microstructures. Micromachines, 10:840. https://doi.org/10.3390/mi10120840
65. Liu X, Shi Q, Wang H, et al., 2018, Automated Fluidic Assembly of Microvessel-Like Structures Using a Multimicromanipulator System. IEEE/ASME Trans Mechatron, 23:667–78. https://doi.org/10.1109/TMECH.2018.2796182
66. Sun T, Shi Q, Liang Q, et al., 2020, Fabrication of Vascular Smooth Muscle-like Tissues Based on Self-organization of Circumferentially Aligned Cells in Microengineered Hydrogels. Lab Chip, 20:3120–31. https://doi.org/10.1039/D0LC00544D
67. Sun T, Wang H, Shi Q, et al., 2016, Micromanipulation for Coiling Microfluidic Spun Alginate Microfibers by Magnetically Guided System. IEEE Robot Autom Lett, 1:808–13. https://doi.org/10.1109/LRA.2016.2524991
68. Sun T, Shi Q, Huang Q, et al., 2017, Magnetic Alginate Microfibers as Scaffolding Elements for the Fabrication of Microvascular-like Structures. Acta Biomater, 66:272–81. https://doi.org/10.1016/j.actbio.2017.11.038
69. Sun T, Huang Q, Shi Q, et al., 2015, Magnetic Assembly of Microfluidic Spun Alginate Microfibers for Fabricating Three-dimensional Cell-laden Hydrogel Constructs. Microfluid Nanofluid, 19:1169–80. https://doi.org/10.1007/s10404-015-1633-x
70. Sun T, Shi Q, Yao Y, et al., 2019, Engineered Tissue Micro-rings Fabricated from Aggregated Fibroblasts and Microfibres for a Bottom-up Tissue Engineering Approach. Biofabrication, 11:035029. https://doi.org/10.1088/1758-5090/ab1ee5
71. Sun T, Yao Y, Shi Q, et al., 2020, Template-based Fabrication of Spatially Organized 3D Bioactive Constructs Using Magnetic Low-concentration Gelation Methacrylate (GelMA) Microfibers. Soft Matter, 16:3902–13. https://doi.org/10.1039/C9SM01945F
72. Onoe H, Takeuchi S, 2008, Microfabricated Mobile Microplates for Handling Single Adherent Cells. J Micromech Microeng, 18:095003. https://doi.org/10.1088/0960-1317/18/9/095003
73. Yoshida S, Sato K, Takeuchi S, 2014, Three-Dimensional Microassembly of Cell-Laden Microplates by In Situ Gluing with Photocurable Hydrogels. Int J Automation Technol, 8(1):95–101. https://doi.org/10.20965/ijat.2014.p0095
74. Jiang X, Ferrigno R, Mrksich M, et al., 2016, Electrochemical Desorption of Self-Assembled Monolayers Noninvasively Releases Patterned Cells from Geometrical Confinements. J Am Chem Soc, 125:2366–7. https://doi.org/10.1021/ja029485c
75. Inaba R, Khademhosseini A, Suzuki H, et al., 2009, Electrochemical Desorption of Self-assembled Monolayers for Engineering Cellular Tissues. Biomaterials, 30:3573–9. https://doi.org/10.1016/j.biomaterials.2009.03.045
76. Seto Y, Inaba R, Okuyama T, et al., 2010, Engineering of Capillary-like Structures in Tissue Constructs by Electrochemical Detachment of Cells. Biomaterials, 31:2209–15. https://doi.org/10.1016/j.biomaterials.2009.11.104
77. Blaeser A, Campos DF, Weber M, et al., 2013, Biofabrication Under Fluorocarbon: A Novel Freeform Fabrication Technique to Generate High Aspect Ratio Tissue-Engineered Constructs. Biores Open Access, 2:374–84. https://doi.org/10.1089/biores.2013.0031
78. Saunders RE, Derby B, 2014, Inkjet Printing Biomaterials for Tissue Engineering: Bioprinting. Int Mater Rev, 59:430–48. https://doi.org/10.1179/1743280414Y.0000000040
79. Ramiah P, du Toit LC, Choonara YE, et al., 2020, Hydrogel-Based Bioinks for 3D Bioprinting in Tissue Regeneration. Front Mater, 7:76. https://doi.org/10.3389/fmats.2020.00076
80. Liu X, Wang X, Zhang L, et al., 2021, A Novel Method for Generating 3D Constructs with Branched Vascular Networks Using Multi-materials Bioprinting and Direct Surgical Anastomosis. bioRxiv, 3:436268. https://doi.org/10.1101/2021.03.21.436268
81. Kolesky DB, Truby RL, Gladman AS, et al., 2014, Bioprinting: 3D Bioprinting of Vascularized, Heterogeneous Cell-Laden Tissue Constructs. Adv Mater, 26:3124–30. https://doi.org/10.1002/adma.201470124
82. Iwan Z, Dietmar WH, et al., 2002, Fused Deposition Modeling of Novel Scaffold Architectures for Tissue Engineering Applications. Biomaterials, 23:1169–85. https://doi.org/10.1016/S0142-9612(01)00232-0
83. Ibrahim TO, Monika H, 2016, Current Advances and Future Perspectives in Extrusion-based Bioprinting. Biomaterials, 76:321–43. https://doi.org/10.1016/j.biomaterials.2015.10.076
84. Fielding GA, Bandyopadhyay A, Bose S, 2012, Effects of Silica and Zinc Oxide Doping on Mechanical and Biological Properties of 3D Printed Tricalcium Phosphate Tissue Engineering Scaffolds. Dent Mater, 28:113–22. https://doi.org/10.1016/j.dental.2011.09.010
85. Wang Y, Huang X, Shen Y, et al., 2019, Direct Writing Alginate Bioink Inside Pre-polymers of Hydrogels to Create Patterned Vascular Networks. J Mater Sci, 54:883–7892. https://doi.org/10.1007/s10853-019-03447-2
86. Bohandy J, Kim BF, Adrian FJ, 1986, Metal Deposition from a Supported Metal Film Using an Excimer Laser. J Appl Phys, 60:1538–9. https://doi.org/10.1063/1.337287
87. Duocastella M, Colina M, Fernández-Pradas JM, et al., 2007, Study of the Laser-induced Forward Transfer of Liquids for Laser Bioprinting. Appl Surf Sci, 253:7855–9. https://doi.org/10.1016/j.apsusc.2007.02.097
88. Kihara T, Kojima M, Horade M, et al., 2016, A Channel Device Generating Multilayer Tubular Structure In Situ Delivering Nutrients. In: The Proceedings of JSME annual Conference on Robotics and Mechatronics (Robomec). p2A2-19b5. https://doi.org/10.1299/jsmermd.2016.2A2-19b5
89. Gao Q, He Y, Fu J, et al., 2015, Coaxial Nozzle-assisted 3D Bioprinting with Built-in Microchannels for Nutrients Delivery. Biomaterials, 61:203–15. https://doi.org/10.1016/j.biomaterials.2015.05.031
90. Li S, Wang K, Jiang X, et al., 2020, Rapid Fabrication of Ready-to-Use Gelatin Scaffolds with Prevascular Networks using Alginate Hollow Fibers as Sacrificial Templates. ACS Biomater Sci Eng, 6:2297–311. https://doi.org/10.1021/acsbiomaterials.9b01834
91. Gauvin R, Chen YC, Lee JW, et al., 2012, Microfabrication of Complex Porous Tissue Engineering Scaffolds using 3D Projection Stereolithography. Biomaterials, 33:3824–34. https://doi.org/10.1016/j.biomaterials.2012.01.048.
92. Raman R, Bhaduri B, Mir M, et al., 2016, High-Resolution Projection Microstereolithography for Patterning of Neovasculature. Adv Healthc Mater, 5:610–9. https://doi.org/10.1002/adhm.201500721
93. Shanjani Y, Pan CC, Elomaa L, et al., 2015, A Novel Bioprinting Method and System for Forming Hybrid Tissue Engineering Constructs. Biofabrication, 7:045008. https://doi.org/10.1088/1758-5090/7/4/045008
94. Yan J, Huang Y, Chrisey DB, 2012, Laser-assisted Printing of Alginate Long Tubes and Annular Constructs. Biofabrication, 5:015002. https://doi.org/10.1088/1758-5082/5/1/015002
95. Tsuda Y, Yamato M, Kikuchi A, et al., 2007, Thermoresponsive Microtextured Culture Surfaces Facilitate Fabrication of Capillary Networks. Adv Mater, 19:3633–6. https://doi.org/10.1002/adma.200700988
96. Horade M, Kojima M, Kamiyama K, et al., 2014, Development of Micro-heater Array Device with Regional Selective Heating for Biochemical Applications. International Conference.
97. Kojima M, Horade M, Takata S, et al., 2018, Development of Micro Heater Array System for Cell Manipulation. IEEE International Conference on Cyborg and Bionic Systems, IEEE.
98. Heinrich MA, Liu W, Jimenez A, et al., 2019, 3D Bioprinting: From Benches to Translational Applications. Small, 15:1805510. http://dx.doi.org/10.1002/smll.201805510
99. Mandrycky C, Wang Z, Kim K, et al., 2016, 3D Bioprinting for Engineering Complex Tissues. Biotechnol Adv, 34:422–34. https://doi.org/10.1016/j.biotechadv.2015.12.011
100. Grigoryan B, Paulsen SJ, Corbett DC, et al., 2019, Multivascular Networks and Functional Intravascular Topologies Within Biocompatible Hydrogels. Science, 364:458–64. http://dx.doi.org/10.1126/science.aav9750
101. Liu LB, Wang XH, 2015, Creation of a Vascular System for Organ Manufacturing. Int J Bioprint, 1:77-86. http://dx.doi.org/10.18063/IJB.2015.01.009.