Coaxial Electrohydrodynamic Bioprinting of Pre-vascularized Cell-laden Constructs for Tissue Engineering
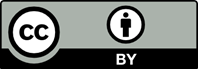
Recapitulating the vascular networks that maintain the delivery of nutrition, oxygen, and byproducts for the living cells within the three-dimensional (3D) tissue constructs is a challenging issue in the tissue-engineering area. Here, a novel coaxial electrohydrodynamic (EHD) bioprinting strategy is presented to fabricate thick pre-vascularized cell-laden constructs. The alginate and collagen/calcium chloride solution were utilized as the outer-layer and inner-layer bioink, respectively, in the coaxial printing nozzle to produce the core-sheath hydrogel filaments. The effect of process parameters (the feeding rate of alginate and collagen and the moving speed of the printing stage) on the size of core and sheath lines within the printed filaments was investigated. The core-sheath filaments were printed in the predefined pattern to fabricate lattice hydrogel with perfusable lumen structures. Endothelialized lumen structures were fabricated by culturing the core-sheath filaments with endothelial cells laden in the core collagen hydrogel. Multilayer core-sheath filaments were successfully printed into 3D porous hydrogel constructs with a thickness of more than 3 mm. Finally, 3D pre-vascularized cardiac constructs were successfully generated, indicating the efficacy of our strategy to engineer living tissues with complex vascular structures.
1. Zhu W, Qu X, Zhu J, et al., 2017, Direct 3D Bioprinting of Prevascularized Tissue Constructs with Complex Microarchitecture. Biomaterials, 124:106–15. https://doi.org/10.1016/j.biomaterials.2017.01.042
2. Baranski JD, Chaturvedi RR, Stevens KR, et al., 2013, Geometric Control of Vascular Networks to Enhance Engineered Tissue Integration and Function. Proc Natl Acad Sci U S A, 110:7586–91. https://doi.org/10.1073/pnas.1217796110
3. Bertassoni LE, Cecconi M, Manoharan V, et al., 2014, Hydrogel Bioprinted Microchannel Networks for Vascularization of Tissue Engineering Constructs. Lab Chip, 14:2202–11. https://doi.org/10.1039/c4lc00030g
4. Qiu Y, Ahn B, Sakurai Y, et al., 2018, Microvasculatureon- a-chip for the Long-term Study of Endothelial Barrier Dysfunction and Microvascular Obstruction in Disease. Nat Biomed Eng, 2:453–63. https://doi.org/10.1038/s41551-018-0224-z
5. Miller JS, Stevens KR, Yang MT, et al., 2012, Rapid Casting of Patterned Vascular Networks for Perfusable Engineered Three-dimensional Tissues. Nat Mater, 11:768. https://doi.org/10.1038/NMAT3357
6. Mao M, Bei HP, Lam CH, et al., 2020, Human-on-Leaf-Chip: A Biomimetic Vascular System Integrated with Chamber-Specific Organs. Small, 16:2000546. https://doi.org/10.1002/smll.202000546
7. He J, Mao M, Liu Y, et al., 2013, Fabrication of Nature-Inspired Microfluidic Network for Perfusable Tissue Constructs. Adv Healthc Mater, 2:1108–13. https://doi.org/10.1002/adhm.201200404
8. Lenoir L, Segonds F, Nguyen KA, et al., 2019, A Methodology to Develop a Vascular Geometry for In Vitro Cell Culture Using Additive Manufacturing. Int J Bioprint, 5:238. https://doi.org/10.18063/ijb.v5i2.238
9. Mirabella T, MacArthur JW, Cheng D, et al., 3D-printed Vascular Networks Direct Therapeutic Angiogenesis in Ischaemia. Nat Biomed Eng, 1:0083. https://doi.org/10.1038/s41551-017-0083
10. Yao R, Alkhawtani AY, Chen R, et al., 2019, Rapid and Efficient In Vivo Angiogenesis Directed by Electro-assisted Bioprinting of Alginate/Collagen Microspheres with Human Umbilical Vein Endothelial Cell Coating Layer. Int J Bioprint, 5:194. https://doi.org/10.18063/ijb.v5i2.1.194
11. Hasan A, Paul A, Vrana NE, et al., 2014, Microfluidic Techniques for Development of 3D Vascularized Tissue. Biomaterials, 35:7308–25. https://doi.org/10.1016/j.biomaterials.2014.04.091
12. Ng WL, Chua CK, Shen YF, 2019, Print Me An Organ! Why We Are Not There Yet. Prog Polym Sci, 97:101145. https://doi.org/10.1016/j.progpolymsci.2019.101145
13. Li X, Liu B, Pei B, et al., 2020, Inkjet Bioprinting of Biomaterials. Chem Rev, 120:10793–833. https://doi.org/10.1021/acs.chemrev.0c00008
14. Jiang T, Munguia-Lopez JG, Flores-Torres S, et al., 2019, Extrusion Bioprinting of Soft Materials: An Emerging Technique for Biological Model Fabrication. Appl Phys Rev, 6:30. https://doi.org/10.1063/1.5059393
15. Ng WL, Lee JM, Zhou M, et al., 2020, Vat Polymerization based Bioprinting-Process, Materials, Applications and Regulatory Challenges. Biofabrication, 12:022001. https://doi.org/10.1088/1758-5090/ab6034
16. Kolesky DB, Homan KA, Skylar-Scott MA, et al., 2016, Three-dimensional Bioprinting of Thick Vascularized Tissues. Proc Natl Acad Sci, 113:3179–84. https://doi.org/10.1073/pnas.1521342113
17. Kolesky DB, Truby RL, Gladman AS, et al., 2014, 3D Bioprinting of Vascularized, Heterogeneous Cell-laden Tissue Constructs. Adv Mater, 26:3124. https://doi.org/10.1002/adma.201305506
18. Feng F, He J, Li J, et al., 2019, Multicomponent Bioprinting of Heterogeneous Hydrogel Constructs Based on Microfluidic Printheads. Int J Bioprint, 5:202. https://doi.org/10.18063/ijb.v5i2.202
19. Kang HW, Lee SJ, Ko IK, et al., 2016, A 3D Bioprinting System to Produce Human-scale Tissue Constructs with Structural Integrity. Nat Biotechnol, 34:312–9. https://doi.org/10.1038/nbt.3413
20. Murphy SV, Atala A, 2014, 3D Bioprinting of Tissues and Organs. Nat Biotechnol, 32:773. https://doi.org/10.1038/nbt.2958
21. He J, Zhang B, Li Z, et al., 2020, High-resolution Electrohydrodynamic Bioprinting: A New Biofabrication Strategy for Biomimetic Micro/Nanoscale Architectures and Living Tissue Constructs. Biofabrication, 12:042002. https://doi.org/10.1088/1758-5090/aba1fa
22. Huo H, Liu F, Luo Y, et al., 2020, Triboelectric Nanogenerators for Electro-assisted Cell Printing. Nano Energy, 67:104150. https://doi.org/10.1016/j.nanoen.2019.104150
23. Gasperini L, Maniglio D, Motta A, et al., An Electrohydrodynamic Bioprinter for Alginate Hydrogels Containing Living Cells. Tissue Eng Part C Methods, 21:123–32. https://doi.org/10.1089/ten.tec.2014.0149
24. Workman VL, Tezera LB, Elkington PT, et al., 2014, Controlled Generation of Microspheres Incorporating Extracellular Matrix Fibrils for Three-Dimensional Cell Culture. Adv Funct Mater, 24:2648–57. https://doi.org/10.1002/adfm.201303891
25. Sampson SL, Saraiva L, Gustafsson K, et al., Cell Electrospinning: An In Vitro and In Vivo Study. Small, 10:78–82. https://doi.org/10.1002/smll.201300804
26. Jayasinghe SN, Qureshi AN, Eagles PA, 2006, Electrohydrodynamic Jet Processing: An Advanced Electric-Field-Driven Jetting Phenomenon for Processing Living Cells. Small, 2:216–9. https://doi.org/10.1002/smll.200500291
27. Wang J, Huang R, Chen H, et al., 2019, Personalized Single-Cell Encapsulation Using E-Jet 3D Printing with AC-Pulsed Modulation. Macromol Mater Eng, 304:1800776. https://doi.org/10.1002/mame.201800776
28. Yeo M, Ha J, Lee H, et al., 2016, Fabrication of hASCs-laden Structures Using Extrusion-based Cell Printing Supplemented with an Electric Field. Acta Biomater, 38:33–43. https://doi.org/10.1016/j.actbio.2016.04.017
29. He J, Zhao X, Chang J, et al., 2017, Microscale Electro-Hydrodynamic Cell Printing with High Viability. Small, 2017:1702626. https://doi.org/10.1002/smll.201702626
30. Liang HT, He JK, Chang JK, et al., 2018, Coaxial Nozzle assisted Electrohydrodynamic Printing for Microscale 3D Cell-laden Constructs. Int J Bioprint, 4:8. https://doi.org/10.18063/IJB.v4i1.127