Developments and Opportunities for 3D Bioprinted Organoids
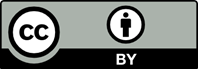
Organoids developed from pluripotent stem cells or adult stem cells are three-dimensional cell cultures possessing certain key characteristics of their organ counterparts, and they can mimic certain biological developmental processes of organs in vitro. Therefore, they have promising applications in drug screening, disease modeling, and regenerative repair of tissues and organs. However, the construction of organoids currently faces numerous challenges, such as breakthroughs in scale size, vascularization, better reproducibility, and precise architecture in time and space. Recently, the application of bioprinting has accelerated the process of organoid construction. In this review, we present current bioprinting techniques and the application of bioinks and summarize examples of successful organoid bioprinting. In the future, a multidisciplinary combination of developmental biology, disease pathology, cell biology, and materials science will aid in overcoming the obstacles pertaining to the bioprinting of organoids. The combination of bioprinting and organoids with a focus on structure and function can facilitate further development of real organs.
1. Fatehullah A, Tan SH, Barker N, 2016, Organoids as an in Vitro Model of Human Development and Disease. Nat Cell Biol, 18:246–54. https://doi.org/10.1038/ncb3312
2. Matano M, Date S, Shimokawa M, et al., 2015, Modeling Colorectal Cancer Using CRISPR-Cas9-Mediated Engineering of Human Intestinal Organoids. Nat Med, 21:256–62. https://doi.org/10.1038/nm.3802
3. Ballard DH, Boyer CJ, Alexander JS, 2019, Organoids-Preclinical Models of Human Disease. N Engl J Med, 380:1981–2.
4. Huch M, Koo BK, 2015, Modeling Mouse and Human Development using Organoid Cultures. Development, 142:3113–25. https://doi.org/10.1242/dev.118570
5. Lui JH, Hansen DV, Kriegstein AR, 2011, Development and Evolution of the Human Neocortex. Cell, 146:18–36. https://doi.org/10.1016/j.cell.2011.06.030
6. Kim J, Koo BK, Knoblich JA, 2020, Human Organoids: Model Systems for Human Biology and Medicine. Nat Rev Mol Cell Biol, 21:571–84. https://doi.org/10.1038/s41580-020-0259-3
7. Groll J, Boland T, Blunk T, et al., 2016, Biofabrication: Reappraising the Definition of an Evolving Field. Biofabrication, 8:5. https://doi.org/10.1088/1758-5090/8/1/013001
8. Heinrich MA, Liu WJ, Jimenez A, et al., 2019, 3D Bioprinting: From Benches to Translational Applications. Small, 15:47.
9. Tuan RS, Boland G, Tuli R, 2003, Adult Mesenchymal Stem Cells and Cell-Based Tissue Engineering. Arthritis Res Ther, 5:32–45.
10. Xu T, Gregory CA, Molnar P, et al., 2006, Viability and Electrophysiology of Neural Cell Structures Generated by the Inkjet Printing Method. Biomaterials, 27:3580–8. https://doi.org/10.1016/j.biomaterials.2006.01.048
11. Li X, Liu B, Pei B, et al., 2020, Inkjet Bioprinting of Biomaterials. Chem Rev, 120:10793–833.
12. Xu C, Chai W, Yong H, et al., 2015, Scaffold-Free Inkjet Printing of Three-Dimensional Zigzag Cellular Tubes. Biotechnol Bioeng, 109:3152–60. https://doi.org/10.1002/bit.24591
13. Lee YB, Polio S, Lee W, et al., 2010, Bio-Printing of Collagen and VEGF-Releasing Fibrin Gel Scaffolds for Neural Stem Cell Culture. Exp Neurol, 223:645–52. https://doi.org/10.1016/j.expneurol.2010.02.014
14. Mandrycky C, Wang Z, Kim K, et al., 2016, 3D Bioprinting for Engineering Complex Tissues. Biotechnol Adv, 34:422–34. https://doi.org/10.1016/j.biotechadv.2015.12.011
15. Vijayavenkataraman S, Wei-Cheng Y, Lu WF, et al., 2018, 3D Bioprinting of Tissues and Organs for Regenerative Medicine. Adv Drug Deliv Rev, 132:296–332. https://doi.org/10.1016/j.addr.2018.07.004
16. Othon CM, Wu X, Anders JJ, et al., 2008, Single-Cell Printing to form Three-Dimensional Lines of Olfactory Ensheathing Cells. Biomed Mater, 3:034101. https://doi.org/10.1088/1748-6041/3/3/034101
17. Pirlo RK, Wu P, Liu J, et al., 2011, PLGA/Hydrogel Biopapers as a Stackable Substrate for Printing HUVEC Networks Via BioLP. Biotechnol Bioeng, 109:262–73. https://doi.org/10.1002/bit.23295
18. Li J, Chen M, Fan X, et al., 2016, Recent Advances in Bioprinting Techniques: Approaches, Applications and Future Prospects. J Transl Med, 14:271. https://doi.org/10.1186/s12967-016-1028-0
19. Bertassoni LE, Cardoso JC, Manoharan V, et al., 2014, Direct-Write Bioprinting of Cell-Laden Methacrylated Gelatin Hydrogels. Biofabrication, 6:024105. https://doi.org/10.1088/1758-5082/6/2/024105
20. Brassard JA, Nikolaev M, Hübscher T, et al., 2020, Recapitulating Macro-Scale Tissue Self-Organization Through Organoid Bioprinting. Nat Mater, 20:22–9. https://doi.org/10.1038/s41563-020-00803-5
21. Daly AC, Prendergast ME, Hughes AJ, et al., 2021, Bioprinting for the Biologist. Cell, 184:18–32. https://doi.org/10.1016/j.cell.2020.12.002
22. Cui H, Nowicki M, Fisher JP, et al., 2016, 3D Bioprinting for Organ Regeneration. Adv Healthc Mater, 6:1601118. https://doi.org/10.1002/adhm.201601118
23. Quan H, Zhang T, Xu H, et al., 2020, Photo-Curing 3D Printing Technique and Its Challenges. Bioact Mater, 5:110–5.
24. Creff J, Courson R, Mangeat T, et al., 2019. Fabrication of 3D Scaffolds Reproducing Intestinal Epithelium Topography by High-Resolution 3D Stereolithography, In: 45th International Conference on Micro and Nano Engineering (MNE 2019). https://doi.org/10.1016/j.biomaterials.2019.119404
25. Norotte C, Marga FS, Niklason LE, et al., 2009, Scaffold-free vascular tissue engineering using bioprinting. Biomaterials, 30:5910–7. https://doi.org/10.1016/j.biomaterials.2009.06.034
26. Nair LS, Laurencin CT, 2007, Biodegradable Polymers as Biomaterials. Prog Polym Sci, 32:762–98.
27. Zhang H, Huang H, Hao G, et al., 2021, 3D Printing Hydrogel Scaffolds with Nanohydroxyapatite Gradient to Effectively Repair Osteochondral Defects in Rats. Adv Funct Mater, 31:2006697. https://doi.org/10.1002/adfm.202006697
28. Skardal A, Devarasetty M, Kang HW, et al., 2016, Bioprinting Cellularized Constructs Using a Tissue-specific Hydrogel Bioink. J Vis Exp, 2016:e53606. https://doi.org/10.3791/53606
29. Markstedt K, Mantas A, Tournier I, et al., 2015, 3D Bioprinting Human Chondrocytes with Nanocellulose-Alginate Bioink for Cartilage Tissue Engineering Applications. Biomacromolecules, 16:1489–96. https://doi.org/10.1021/acs.biomac.5b00188
30. Gruene M, Pflaum M, Hess C, et al., Laser Printing of Three-Dimensional Multicellular Arrays for Studies of Cell-Cell and Cell-Environment Interactions. Tissue Eng Part C Methods, 17:973–82. https://doi.org/10.1089/ten.tec.2011.0185
31. Flores-Torres S, Peza-Chavez O, Kuasne H, et al., 2021, Alginate-gelatin-Matrigel hydrogels enable the development and multigenerational passaging of patient-derived 3D bioprinted cancer spheroid models. Biofabrication, 13:025001 https://doi.org/10.1088/1758-5090/abdb87
32. Tirziu D, Giordano FJ, Simons M, 2010, Cell Communications in the Heart. Circulation, 122:928–37.
https://doi.org/10.1161/circulationaha.108.847731
33. Bergmann O, Zdunek S, Felker A, et al., 2015, Dynamics of Cell Generation and Turnover in the Human Heart. Cell, 161:1566–75.
34. Lee A, Hudson AR, Shiwarski DJ, et al., 2019, 3D Bioprinting of Collagen to Rebuild Components of the Human Heart. Science, 365:482–7.
35. Hinton TJ, Jallerat Q, Palchesko RN, et al., 2015, Three-Dimensional Printing of Complex Biological Structures by Freeform Reversible Embedding of Suspended Hydrogels. Sci Adv, 1:e1500758. https://doi.org/10.1126/sciadv.1500758
36. Noor N, Shapira A, Edri R, et al., 2019, 3D Printing of Personalized Thick and Perfusable Cardiac Patches and Hearts. Adv Sci, 6:1900344. https://doi.org/10.1002/advs.201900344
37. Lee J, Sutani A, Kaneko R, et al., 2020, In Vitro Generation of Functional Murine Heart Organoids via FGF4 and Extracellular Matrix. Nat Commun, 11:4283. https://doi.org/10.1038/s41467-020-18031-5
38. Drakhlis, L., Biswanath, S., Farr, CM. et al. Human heart forming organoids recapitulate early heart and foregut development. Nat Biotechnol 39:737–746 (2021).
39. Kupfer ME, Lin WH, Ravikumar V, et al., 2020, In Situ Expansion, Differentiation, and Electromechanical Coupling of Human Cardiac Muscle in a 3D Bioprinted, Chambered Organoid. Circ Res, 127:207–24. https://doi.org/10.1161/circresaha.119.316155
40. Nishinakamura R, 2019, Human Kidney Organoids: Progress and Remaining Challenges. Nat Rev Nephrol, 15:613–24. https://doi.org/10.1038/s41581-019-0176-x
41. Taguchi A, Kaku Y, Ohmori T, et al., 2014, Redefining the In Vivo Origin of Metanephric Nephron Progenitors Enables Generation of Complex Kidney Structures from Pluripotent Stem Cells. Cell Stem Cell, 14:53–67. https://doi.org/10.1016/j.stem.2013.11.010
42. Takasato M, Er PX, Chiu HS, et al., 2016, Kidney Organoids from Human iPS Cells Contain Multiple Lineages and Model Human Nephrogenesis. Nature, 536:238. https://doi.org/10.1038/nature17982
43. Homan KA, Kolesky DB, Skylar-Scott MA, et al., 2016, Bioprinting of 3D Convoluted Renal Proximal Tubules on Perfusable Chips. Scic Rep, 6:34845. https://doi.org/10.1038/srep34845
44. King SM, Higgins JW, Nino CR, et al., 2017, 3D Proximal Tubule Tissues Recapitulate Key Aspects of Renal Physiology to Enable Nephrotoxicity Testing. Front Physiol, 8:18. https://doi.org/10.3389/fphys.2017.00123
45. Lawlor KT, Vanslambrouck JM, Higgins JW, et al., Cellular Extrusion Bioprinting Improves Kidney Organoid Reproducibility and Conformation. Nat Mater, 20:260–71. https://doi.org/10.3390/mi10100676
46. Deng J, Wei WB, Chen ZZ, et al., 2019, Engineered Liver-On-A-Chip Platform to Mimic Liver Functions and Its Biomedical Applications: A Review. Micromachines, 10:26.
47. Huch M, Dorrell C, Boj SF, et al., 2013, In Vitro Expansion of Single Lgr5(+) Liver Stem Cells Induced by Wnt-Driven Regeneration. Nature, 494:247–50. https://doi.org/10.1038/nature11826
48. Takebe T, Sekine K, Enomura M, et al., 2013, Vascularized and Functional Human Liver from an iPSC-Derived Organ Bud Transplant. Nature, 499:481–4. https://doi.org/10.1038/nature12271
49. Tostões RM, Leite SB, Serra M, et al., 2012, Human Liver Cell Spheroids in Extended Perfusion Bioreactor Culture for Repeated-Dose Drug Testing. Hepatology, 55:1227–36. https://doi.org/10.1002/hep.24760
50. Tobias G, Alicia R, Alexander T, et al., 2018, Bioprinting Perfusion-Enabled Liver Equivalents for Advanced Organ-On-A-Chip Applications. Genes, 9:176.
51. Kang D, Hong G, An S, et al., 2020, Bioprinting of Multiscaled Hepatic Lobules within a Highly Vascularized Construct. Small, 16:1905505. https://doi.org/10.1002/smll.201905505
52. Kaur S, Tripathi DM, Ghosh S, 2020, Three-Dimensional Bioprinted Hepatorganoids in Liver Failure. Gut, 70:998–9. https://doi.org/10.1136/gutjnl-2020-322317
53. Boj SF, Hwang CI, Baker LA, et al., 2015, Organoid Models of Human and Mouse Ductal Pancreatic Cancer. Cell, 160:324–38.
54. Chua CW, Shibata M, Lei M, et al., 2014, Single Luminal Epithelial Progenitors Can Generate Prostate Organoids in Culture. Nat Cell Biol, 16:951. https://doi.org/10.1038/ncb3047
55. Linnemann JR, Miura H, Meixner LK, et al., 2015, Quantification of Regenerative Potential in Primary Human Mammary Epithelial Cells. Development, 142:3239–51.
56. Sarvestani SK, Signs S, Hu B, et al., 2021, Induced Organoids Derived from Patients with Ulcerative Colitis Recapitulate Colitic Reactivity. Nat Commun, 12:262. https://doi.org/10.1038/s41467-020-20351-5
57. Qu ML, Xiong L, Lyu YL, et al., Establishment of Intestinal Organoid Cultures Modeling Injury-Associated Epithelial Regeneration. Cell Res, 31:259–71. https://doi.org/10.1038/s41422-020-00453-x
58. Curvello R, Kerr G, Micati DJ, et al., 2021, Engineered Plant-Based Nanocellulose Hydrogel for Small Intestinal Organoid Growth. Adv Sci, 8:2002135. https://doi.org/10.1002/advs.202002135
59. Lukonin I, Serra D, Meylan LC, et al., 2020, Phenotypic Landscape of Intestinal Organoid Regeneration. Nature, 586:275–80.
60. Kim WJ, Kim GH, 2020, An Intestinal Model with a Finger-Like Villus Structure Fabricated Using a Bioprinting Process and Collagen/SIS-Based Cell-Laden Bioink. Theranostics, 10:2495–508. https://doi.org/10.7150/thno.41225
61. Madden LR, Nguyen TV, Garcia-Mojica S, et al., 2018, Bioprinted 3D Primary Human Intestinal Tissues Model Aspects of Native Physiology and ADME/Tox Functions. Iscience, 2:156–67. https://doi.org/10.1016/j.isci.2018.03.015
62. Huang L, Holtzinger A, Jagan I, et al., 2015, Ductal Pancreatic Cancer Modeling and Drug Screening Using Human Pluripotent Stem Cell-and Patient-Derived Tumor Organoids. Nat Med, 21:1364–71. https://doi.org/10.1158/1538-7445.panca16-b45
63. Dominijanni A, Mazzocchi A, Shelkey E, et al., 2020, Bioengineered Tumor Organoids. Curr Opin Biomed Eng, 13:168–73.
64. Ma L, Li Y, Wu Y, et al., 2020, The Construction of In Vitro Tumor Models Based on 3D Bioprinting. Biodes Manuf, 3:227–36.
65. Drost J, Karthaus W R, Gao D, et al., Organoid Culture Systems for Prostate Epithelial and Cancer Tissue. Nat Protoc, 11:347–58. https://doi.org/10.1038/nprot.2016.006
66. Fujii M, Shimokawa M, Date S, et al., 2016, A Colorectal Tumor Organoid Library Demonstrates Progressive Loss of Niche Factor Requirements during Tumorigenesis. Cell Stem Cell, 18:827–38. https://doi.org/10.1016/j.stem.2016.04.003
67. Zhao Y, Yao R, Ouyang L, et al., 2014, Three-Dimensional Printing of Hela Cells for Cervical Tumor Model In Vitro. Biofabrication, 6:035001. https://doi.org/10.1088/1758-5082/6/3/035001
68. Cao X, Ashfaq R, Cheng F, et al., 2019, A Tumor-On-A-Chip System with Bioprinted Blood and Lymphatic Vessel Pair. Adv Funct Mater, 29:1807173. https://doi.org/10.1002/adfm.201807173
69. Yi HG, Jeong YH, Kim Y, et al., A Bioprinted Human-Glioblastoma-On-A-Chip for the Identification of Patient-Specific Responses to Chemoradiotherapy. Nat Biomed Eng, 3:509–19. https://doi.org/10.1038/s41551-019-0363-x
70. Bonneh-Barkay D, Wiley CA, 2009, Brain Extracellular Matrix in Neurodegeneration. Brain Pathol, 19:573–85. https://doi.org/10.1111/j.1750-3639.2008.00195.x
71. Stiles J, Jernigan TL, 2010, The Basics of Brain Development. Neuropsychol Rev, 20:327–48.
72. Trevino AE, Sinnott-Armstrong N, Andersen J, et al., 2020, Chromatin Accessibility Dynamics in a Model of Human Forebrain Development. Science, 367:eaay1645. https://doi.org/10.1126/science.aay1645
73. Li YC, Jodat YA, Samanipour R, et al., 2021, Toward a Neurospheroid Niche Model: Optimizing Embedded 3D Bioprinting for Fabrication of Neurospheroid Brain-Like Co-Culture Constructs. Biofabrication, 13:015014. https://doi.org/10.1088/1758-5090/abc1be
74. Willerth SM, 2018, Bioprinting Neural Tissues using Stem Cells as a Tool for Screening Drug Targets for Alzheimer’s Disease. J 3D Print Med, 2:1–4.
75. Skylar-Scott M, Huang J, Lu A, et al., 2020, An Orthogonal Differentiation Platform for Genomically Programming Stem Cells, Organoids, and Bioprinted Tissues, bioRxiv. https://doi.org/10.1101/2020.07.11.198671
76. Rothenbücher T, Gürbüz H, Emnéus J, et al., 2021, Next Generation Human Brain Models: Engineered Flat Brain Organoids Featuring Gyrification. Biofabrication, 13:011001. https://doi.org/10.1088/1758-5090/abc95e
77. Abigail I, Stephen S, Connon CJ, 2018, 3D Bioprinting of a Corneal Stroma Equivalent. Exp Eye Res, 173:188–93. https://doi.org/10.1016/j.exer.2018.05.010
78. Kim J, Shim IK, Hwang DG, et al., 2019, 3D Cell Printing of Islet-Laden Pancreatic Tissue-Derived Extracellular Matrix Bioink Constructs for Enhancing Pancreatic Functions. J Mater Chem B. 7:4592. https://doi.org/10.1039/c9tb90097g
79. Han Y, Yang L, Duan X, et al., 2020, Identification of Candidate COVID-19 Therapeutics using hPSC-Derived Lung Organoids, bioRxiv.
80. Grigoryan B, Paulsen SJ, Corbett DC, et al., 2019, Biomedicine Multivascular Networks and Functional Intravascular Topologies within Biocompatible Hydrogels. Science, 364:458–64.
81. Vichas A, Zallen JA, 2011, Translating Cell Polarity into Tissue Elongation. Semin Cell Dev Biol, 22:858–64. https://doi.org/10.1016/j.semcdb.2011.09.013
82. Davis GE, Cleaver OB, 2014, Outside in: Inversion of Cell Polarity Controls Epithelial Lumen Formation. Dev Cell, 31:140–2. https://doi.org/10.1016/j.devcel.2014.10.011
83. Reid JA, Mollica PM, Bruno RD, et al., 2018, Consistent and Reproducible Cultures of Large-Scale 3D Mammary Epithelial Structures using an Accessible Bioprinting Platform. Breast Cancer Res, 20:122. https://doi.org/10.1186/s13058-018-1045-4
84. Kim E, Choi S, Kang B, et al., 2020, Creation of Bladder Assembloids Mimicking Tissue Regeneration and Cancer. Nature, 588:664–9. https://doi.org/10.1038/s41586-020-3034-x
85. Fujii M, Matano M, Toshimitsu K, et al., 2018, Human Intestinal Organoids Maintain Self-Renewal Capacity and Cellular Diversity in Niche-Inspired Culture Condition. Cell Stem Cell, 23:787–93. https://doi.org/10.1016/j.stem.2018.11.016
86. Hu H, Gehart H, Artegiani B, et al., 2018, Long-Term Expansion of Functional Mouse and Human Hepatocytes as 3D Organoids. Cell, 175:1591–606.e19. https://doi.org/10.1016/j.cell.2018.11.013
87. Sato T, Vries RG, Snippert HJ, et al., 2009, Single Lgr5 Stem Cells Build Crypt-Villus Structures In Vitro Without a Mesenchymal Niche. Nature, 459:262–5. https://doi.org/10.1038/nature07935
88. Sato T, Stange DE, Ferrante M, et al., 2011, Long-Term Expansion of Epithelial Organoids From Human Colon, Adenoma, Adenocarcinoma, and Barrett’s Epithelium. Gastroenterology, 141:1762–72. https://doi.org/10.1053/j.gastro.2011.07.050
89. Wilson WC, Boland T, 2003, Cell and Organ Printing 1: Protein and Cell Printers. Anat Rec A Discov Mol Cell Evol Biol, 272A:491–6. https://doi.org/10.1002/ar.a.10057
90. Rawal P, Tripathi DM, Ramakrishna S, et al., Prospects for 3D Bioprinting of Organoids. Biodes Manuf, 4:627–40. https://doi.org/10.1007/s42242-020-00124-1
91. Yap KK, Gerrand YW, Dingle AM, et al., 2020, Liver Sinusoidal Endothelial Cells Promote the Differentiation and Survival of Mouse Vascularised Hepatobiliary Organoids. Biomaterials, 251:120091. https://doi.org/10.1016/j.biomaterials.2020.120091
92. Silvestri V L, Henriet E, Linville R M, et al., 2020, A Tissue-Engineered 3D Microvessel Model Reveals the Dynamics of Mosaic Vessel Formation in Breast Cancer. Cancer Res, 80:4288–301. https://doi.org/10.1158/0008-5472.can-19-1564
93. Markou M, Kouroupis D, Badounas F, et al., 2020, Tissue Engineering Using Vascular Organoids From Human Pluripotent Stem Cell Derived Mural Cell Phenotypes. Front Bioeng Biotechnol, 8:278. https://doi.org/10.3389/fbioe.2020.00278
94. Song KH, Highley CB, Rouff A, et al., 2018, Complex 3D-Printed Microchannels within Cell-Degradable Hydrogels. Adv Funct Mater, 28:1801331. https://doi.org/10.1002/adfm.201801331
95. Humphreys BD, 2021, Bioprinting Better Kidney Organoids. Nat Mater, 20:128–30.