3D Topology Optimization and Mesh Dependency for Redesigning Locking Compression Plates Aiming to Reduce Stress Shielding
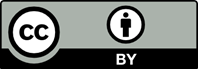
Current fixation plates for bone fracture treatments are built with biocompatible metallic materials such as stainless steel, titanium, and its alloys (e.g., Ti6Al4V). The stiffness mismatch between the metallic material of the plate and the host bone leads to stress shielding phenomena, bone loss, and healing deficiency. This paper explores the use of three dimensional topology-optimization, based on compliance (i.e., strain energy) minimization, reshaping the design domain of three locking compression plates (four-screw holes, six-screw holes, and eight-screw holes), considering different volume reductions (25, 45, and 75%) and loading conditions (bending, compression, torsion, and combined loads). A finite-element study was also conducted to measure the stiffness of each optimized plate. Thirty-six designs were obtained. Results showed that for a critical value of volume reductions, which depend on the load condition and number of screws, it is possible to obtain designs with lower stiffness, thereby reducing the risk of stress shielding.
1. Amin S, Achenback S, Atkinson E, et al., 2014, Trends in Fracture Incidence: A Population-based Study Over 20 Years. J Bone Miner Res, 29:581–9. https://doi.org/10.1002/jbmr.2072
2. Kramer D, Xu S, Kesselheim A, 2012, Regulation of Medical Devices in the United States and European Union. N Engl J Med, 366:848–55. https://doi.org/10.1056/nejmhle1113918
3. Green M, Wishart N, Young E, 2016, NJR 14th Annual Report. National Joint Registry, England.
4. Alsop H, 2013, An introduction to fractures’ in porter. In: Tidy’s Physiotherapy. 15th ed., Ch. 22. Churchill Livingstone, United Kingdom.
5. Uhthoff HK, Poitras P, Backman DS, 2006, Internal Plate Fixation of Fractures: Short History and Recent Developments. J Orthop Sci, 11:118–26. https://doi.org/10.1007/s00776-005-0984-7
6. Szypryt P, Forward D, 2009, The Use and Abuse of Locking Plates. Orthop Trauma, 23:281–90. https://doi.org/10.1016/j.mporth.2009.07.002
7. Elias CN, Lima JHC, Valiev R, et al., 2008, Biomedical Applications of Titanium and its Alloys. JOM, 60:46–9. https://doi.org/10.1007/s11837-008-0031-1
8. McNamara L, 2011, Bone as a Material in Ducheyne. In: Comprehensive Biomaterials, Elsevier, Oxford.
9. Prasad K, Bazaka O, Chua M, et al., 2017, Metallic Biomaterials: Current Challenges and Opportunities. Materials, 10:884. https://doi.org/10.3390/ma10080884
10. Mahmoud D, Elbestawi MA, 2017, Lattice Structures and Functionally Graded Materials Applications in Additive Manufacturing of Orthopedic Implants: A Review. J Manufact Mater Proc, 1:13. https://doi.org/10.3390/jmmp1020013
11. Pałka K, Pokrowiecki R, 2018, Porous Titanium Implants: A Review. Adv Eng Mater, 20:1700648. https://doi.org/10.1002/adem.201700648
12. Al-Tamimi A, Fernandes PRA, Peach C, et al., 2017, Metallic Bone Fixation Implants: A Novel Design Approach for Reducing the Stress Shielding Phenomenon. Virtual Phys Prototy, 12:141–51. https://doi.org/10.1080/17452759.2017.1307769
13. Bendsøe MP, 1989, Optimal Shape Design as a Material Distribution Problem. Struct Optim, 1:193–202. https://doi.org/10.1007/bf01650949
14. Bendsøe MP, Sigmund O, 2004, Topology Optimization by Distribution of Isotropic Material in Topology Optimization: Theory, Methods, and Applications. Springer Berlin Heidelberg, Berlin, Heidelberg. https://doi.org/10.1007/978-3-662-05086-6_1
15. British Standard, 1991, Implants for Osteosynthesis-Part 23: Bone Plates-Section 23.1 Method for Determination of Bending Strength and Stiffness. British Standard, United Kingdom. https://doi.org/10.3403/00189873u
16. Sigmund O, Petersson J, 1998, Numerical Instabilities in Topology Optimization: A Survey on Procedures Dealing with Checkerboards, Mesh-dependencies and Local Minima. Struct Optim, 16:68–75. https://doi.org/10.1007/bf01214002
17. ASTM Standard, 2017, Standard Test Method for Flexural Properties of Unreinforced and Reinforced Plastics and Electrical Insulating Materials by Four-point Bending, ASTM Standard, United States, pD6272–17. https://doi.org/10.1520/d6272-02r08e01
18. Buhl T, Pedersen C, Sigmund O, 2000, Stiffness Design of Geometrically Nonlinear Structures Using Topology Optimization. Struct Multidisc Optim, 19:93–104. https://doi.org/10.1007/s001580050089
19. Iqbal T, Wang L, Li D, et al., 2019, A General Multi objective Topology Optimization Methodology Developed for Customized Design of Pelvic Prostheses. Med Eng Phys, 69:8–16. https://doi.org/10.1016/j.medengphy.2019.06.008
20. Saravana KG, George S, 2017, Optimization of Custom Cementless Stem Using Finite Element Analysis and Elastic Modulus Distribution for Reducing Stress-shielding Effect. Proc Inst Mech Eng H, 231:149–59. https://doi.org/10.1177/0954411916686125
21. Chuah HG, Rahim IA, Yusof MI, 2010, Topology Optimisation of Spinal Interbody Cage for Reducing Stress Shielding Effect. Comput Methods Biomech Biomed Eng, 13:319–26. https://doi.org/10.1080/10255840903208189
22. Woo S, Simon B, Akeson W, et al., 1977, An Interdisciplinary Approach to Evaluate the Effect of Internal Fixation Plate on Long Bone Remodeling. J Biomech, 10:87–95. https://doi.org/10.1016/0021-9290(77)90072-0
23. Goodship A, Kenwright J, 1985, The Influence of Induced Micromovement Upon the Healing of Experimental Tibial Fractures. J Bone Joint Surg Br, 67:650–5. https://doi.org/10.1302/0301-620x.67b4.4030869
24. Claes L, Augat P, Suger G, et al., 1997, Influence of Size and Stability of the Osteotomy Gap on the Success of Fracture Healing. J Orthop Res, 15:577–84. https://doi.org/10.1002/jor.1100150414
25. Keller TS, Mao Z, Spengler DM, 1990, Young’s Modulus, Bending Strength, and Tissue Physical Properties of Human Compact Bone. J Orthop Res, 8:592–603. https://doi.org/10.1002/jor.1100080416
26. Al-Tamimi AA, Quental C, Folgado J, et al., 2020, Stress Analysis in a Bone Fracture Fixed with Topology-optimised Plates. Biomech Model Mechanobiol, 19:693–9. https://doi.org/10.1007/s10237-019-01240-3
27. Liu Y, Fan Y, Jiang X, et al., 2017. A Customized Fixation Plate with Novel Structure Designed by Topological Optimization for Mandibular Angle Fracture Based on Finite Element Analysis. BioMedical Eng Online, 16:1–17. https://doi.org/10.1186/s12938-017-0422-z
28. Zegard T, Paulino GH, 2016, Bridging Topology Optimization and Additive Manufacturing. Struct Multidiscip Optim, 53:175–92. https://doi.org/10.1007/s00158-015-1274-4
29. Al-Tamimi AA, Hernandez MA, Omar A, et al., 2020, Mechanical, Biological and Tribological Behaviour of Fixation Plates 3D Printed by Electron Beam and Selective Laser Melting. Int J Adv Manufact Technol, 109: 673–88. https://doi.org/10.1007/s00170-020-05676-1