The Technique of Thyroid Cartilage Scaffold Support Formation for Extrusion-Based Bioprinting
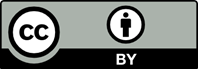
During biofabrication, a tissue scaffold may require temporary support. The aim of this study was to develop an approach of human thyroid cartilage scaffold temporal support formation. The scaffold 3D-model was based on DICOM images. XY plane projections were used to form scaffold supporting part. To verify the technique, collagen hydrogel was chosen as the main scaffold component. Gelatin was applied for the supporting part. To test the applicability of the approach, a model of thyroid cartilage scaffold with the support was printed. The scaffold corresponded to a given model, although some discrepancy in geometry was observed during verification by computed tomography.
1. Ng WL, Chua CK, Shen YF, 2019, Print Me An Organ! Why We Are Not There Yet. Prog Polym Sci, 97:101145. https://doi.org/10.1016/j.progpolymsci.2019.101145
2. Lee JM, Ng WL, Yeong WY, 2019, Resolution and Shape in Bio-Printing: Strategizing Towards Complex Tissue and Organ Printing. Appl Phys Rev, 6:11307. https://doi.org/10.1063/1.5053909
3. Engler AJ, Sen S, Sweeney HL, et al., 2006, Matrix Elasticity Directs Stem Cell Lineage Specification. Cell, 126:677–89. https://doi.org/10.1016/j.cell.2006.06.044
4. Hadden WJ, Young JL, Holle AW, et al., 2017, Stem Cell Migration and Mechanotransduction on Linear Stiffness Gradient Hydrogels. Proc Natl Acad Sci, 114:5647–52. https://doi.org/10.1073/pnas.1618239114
5. Ke D, Yi H, Est-Witte S, et al., 2019, Bioprinted Trachea Constructs With Patient-Matched Design, Mechanical and Biological Properties. Biofabrication, 12:15022. https://doi.org/10.1088/1758-5090/ab5354
6. Ouyang L, Highley CB, Sun W, et al., 2017, A Generalizable Strategy for the 3D Bioprinting of Hydrogels from Non Viscous Photo-Crosslinkable Inks. Adv Mater, 29: 1604983. https://doi.org/10.1002/adma.201604983
7. Kim SH, Yeon YK, Lee JM, et al., 2018, Publisher Correction: Precisely Printable and Biocompatible Silk Fibroin Bio-Ink for Digital Light Processing 3D Printing, Nat Commun, 9:2350. https://doi.org/10.1038/s41467-018-04517-w
8. Onofrillo C, Duchi S, O’Connell CD, et al., 2018, Biofabrication of Human Articular Cartilage: A Path towards the Development of a Clinical Treatment. Biofabrication, 10:45006. https://doi.org/10.1088/1758-5090/aad8d9
9. Kajave NS, Schmitt T, Nguyen TU, et al., 2020, Dual Crosslinking Strategy to Generate Mechanically Viable Cell-Laden Printable Constructs using Methacrylated Collagen Bio-Inks. Mater Sci Eng C Mater Biol Appl, 107:110290. https://doi.org/10.1016/j.msec.2019.110290
10. Park JH, Hong JM, Ju YM, et al., 2015, A Novel Tissue-Engineered Trachea With a Mechanical Behavior Similar to Native Trachea. Biomaterials, 62:106–15. https://doi.org/10.1016/j.biomaterials.2015.05.008
11. Gao M, Zhang H, Dong W, et al., 2017, Tissue-Engineered Trachea from a 3D-Printed Scaffold Enhances Whole-Segment Tracheal Repair. Sci Rep, 7:5246. https://doi.org/10.1038/s41598-017-05518-3
12. Osidak EO, Karalkin PA, Osidak MS, et al., 2019, Viscoll Collagen Solution as a Novel Bio-Ink for Direct 3D Bio-Printing. J Mater Sci Mater Med, 30:31.
13. Yang LJ, Lin WZ, Yao TJ, et al., 2003, Photo-Patternable Gelatin as Protection Layers in Low-Temperature Surface Micromachinings. Sens Actuators A Phys, 103:284–9. https://doi.org/10.1016/s0924-4247(02)00338-2
14. Gartland A, Mechler J, Mason-Savas A, et al., 2005, In Vitro Chondrocyte Differentiation using Costochondral Chondrocytes as a Source of Primary Rat Chondrocyte Cultures: An Improved Isolation and Cryopreservation Method. Bone, 37:530–44. https://doi.org/10.1016/j.bone.2005.04.034
15. Gosset M, Berenbaum F, Thirion S, et al., 2008, Primary Culture and Phenotyping of Murine Chondrocytes. Nat Protoc, 3:1253–60. https://doi.org/10.1038/nprot.2008.95
16. Apelgren P, Amoroso M, Lindahl A, et al., 2017, Chondrocytes and Stem Cells in 3D-Bioprinted Structures Create Human Cartilage In Vivo. PLoS One, 12:e0189428. https://doi.org/10.1371/journal.pone.0189428
17. Jung CS, Kim BK, Lee J, et al., 2017, Development of Printable Natural Cartilage Matrix Bio-Ink for 3D Printing of Irregular Tissue Shape. Tissue Eng Regen Med, 15:155–62. https://doi.org/10.1007/s13770-017-0104-8
18. Sun N, Shib T, Fanc Y, et al., 2018, Experimental Study on 3D Chi-Hap Scaffolds for Thyroid Cartilage Repairing. IOP Conf Ser Mater Sci Eng, 301:012018. https://doi.org/10.1088/1757-899x/301/1/012018
19. Omori K, Tada Y, Suzuki T, et al., 2008, Clinical Application of In Situ Tissue Engineering using a Scaffolding Technique for Reconstruction of the Larynx and Trachea. Ann Otol Rhinol Laryngol, 117:673–8. https://doi.org/10.1177/000348940811700908
20. Hinton TJ, Jallerat Q, Palchesko RN, et al., 2015, Three-Dimensional Printing of Complex Biological Structures by Freeform Reversible Embedding of Suspended Hydrogels. Sci Adv, 1:e1500758. https://doi.org/10.1126/sciadv.1500758
21. Lee A, Hudson AR, Shiwarski DJ, et al., 2019, 3D Bioprinting of Collagen to Rebuild Components of the Human Heart. Science, 365:482–7.
22. Bhattacharjee T, Zehnder SM, Rowe KG, et al., 2015, Writing in the Granular Gel Medium. Sci Adv, 1:e1500655. https://doi.org/10.1126/sciadv.1500655
23. Wu W, DeConinck A, Lewis JA, 2011, Omnidirectional Printing of 3D Microvascular Networks. Adv Mater, 23:H178–83. https://doi.org/10.1002/adma.201004625
24. Lee W, Lee V, Polio S, et al., 2010, On-Demand Three-Dimensional Freeform Fabrication of Multi-Layered Hydrogel Scaffold with Fluidic Channels. Biotechnol Bioeng, 105:1178–86. https://doi.org/10.1002/bit.22613
25. Miller JS, Stevens KR, Yang MT, et al., 2012, Rapid Casting of Patterned Vascular Networks for Perfusable Engineered Three-Dimensional Tissues. Nat Mater, 11(9):768–74. https://doi.org/10.1038/nmat3357
26. Bertassoni LE, Cecconi M, Manoharan V, et al., 2014, Hydrogel Bioprinted Microchannel Networks for Vascularization of Tissue Engineering Constructs. Lab Chip, 14:2202–11. https://doi.org/10.1039/c4lc00030g
27. Lee VK, Kim DY, Ngo H, et al., 2014, Creating Perfused Functional Vascular Channels using 3D Bio-Printing Technology. Biomaterials, 35:8092–102. https://doi.org/10.1016/j.biomaterials.2014.05.083
28. Lee VK, Lanzi AM, Haygan N, et al., 2014, Generation of Multi-Scale Vascular Network System within 3D Hydrogel using 3D Bio-Printing Technology. Cell Mol Bioeng, 7:460–72. https://doi.org/10.1007/s12195-014-0340-0
29. Skylar-Scott MA, Uzel SG, Nam LL, et al., 2019, Biomanufacturing of Organ-Specific Tissues with High Cellular Density and Embedded Vascular Channels. Sci Adv, 5:eaaw2459. https://doi.org/10.1126/sciadv.aaw2459
30. Dong C, Lv Y, 2016, Application of Collagen Scaffold in Tissue Engineering: Recent Advances and New Perspectives. Polymers, 8:42. https://doi.org/10.3390/polym8020042
31. Lee J, Kim G, 2018, Three-Dimensional Hierarchical Nanofibrous Collagen Scaffold Fabricated using Fibrillated Collagen and Pluronic F-127 for Regenerating Bone Tissue. ACS Appl Mater Interfaces, 10:35801–11. https://doi.org/10.1021/acsami.8b14088
32. Doyle AD, 2016, Generation of 3D Collagen Gels with Controlled Diverse Architectures. Curr Protoc Cell Biol, 72:10.20.1–16.
33. Naciri M, Kuystermans D, Al-Rubeai M, 2008, Monitoring pH and Dissolved Oxygen in Mammalian Cell Culture Using Optical Sensors. Cytotechnology, 57:245–50. https://doi.org/10.1007/s10616-008-9160-1
34. Kim YB, Lee H, Kim GH, 2016, Strategy to Achieve Highly Porous/Biocompatible Macroscale Cell Blocks, using a Collagen/Genipin-Bioink and an Optimal 3D Printing Process. ACS Appl Mater Interfaces, 8:32230–40. https://doi.org/10.1021/acsami.6b11669
35. Lee J, Yeo M, Kim W, et al., 2018, Development of a Tannic Acid Cross-Linking Process for Obtaining 3D Porous Cell-Laden Collagen Structure. Int J Biol Macromol, 110: 497–503. https://doi.org/10.1016/j.ijbiomac.2017.10.105
36. Peng YY, Glattauer V, Ramshaw JA, 2017, Stabilisation of Collagen Sponges by Glutaraldehyde Vapour Crosslinking, Int J Biomater, 2017:8947823. https://doi.org/10.1155/2017/8947823
37. Mu C, Liu F, Cheng Q, et al., 2010, Collagen Cryogel Cross-Linked by Dialdehyde Starch. Macromol Mater Eng, 295:100–7. https://doi.org/10.1002/mame.200900292
38. Drzewiecki KE, Malavade JN, Ahmed I, et al., 2017, A Thermoreversible, Photocrosslinkable Collagen Bio-Ink for Free-Form Fabrication of Scaffolds for Regenerative Medicine, Technology (Singap World Sci), 5:185–95. https://doi.org/10.1142/s2339547817500091
39. Diamantides N, Wang L, Pruiksma T, et al., 2017, Correlating Rheological Properties and Printability of Collagen Bioinks: The Effects of Riboflavin Photocrosslinking and pH. Biofabrication, 9:34102. https://doi.org/10.1088/1758-5090/aa780f
40. Shi W, Sun M, Hu X, et al., 2017, Structurally and Functionally Optimized Silk-Fibroin-Gelatin Scaffold Using 3D Printing to Repair Cartilage Injury In Vitro and In Vivo. Adv Mater, 29: 1701089. https://doi.org/10.1002/adma.201701089
41. Setayeshmehr M, Esfandiari E, Rafieinia M, et al., 2019, Hybrid and Composite Scaffolds Based on Extracellular Matrices for Cartilage Tissue Engineering. Tissue Eng Part B Rev, 25:202–24. https://doi.org/10.1089/ten.teb.2018.0245
42. Fitzsimmons R, Aquilino MS, Quigley J, et al., 2018, Generating Vascular Channels within Hydrogel Constructs using an Economical Open-Source 3D Bioprinter and Thermoreversible Gels. Bioprinting, 9:7–18. https://doi.org/10.1016/j.bprint.2018.02.001
43. Kolesky DB, Truby RL, Gladman AS, et al., 2014, 3D Bioprinting of Vascularized, Heterogeneous Cell-Laden Tissue Constructs. Adv Mater, 26:3124–30. https://doi.org/10.1002/adma.201305506
44. Paulsen SJ, Miller JS, 2015, Tissue Vascularization through 3D Printing: Will Technology Bring us Flow? Dev Dyn, 244:629–40. https://doi.org/10.1002/dvdy.24254
45. Kim HD, Lee Y, Kim Y, et al., 2017, Biomimetically Reinforced Polyvinyl Alcohol-Based Hybrid Scaffolds for Cartilage Tissue Engineering. Polymers, 9:655. https://doi.org/10.3390/polym9120655