Bioinks for 3D Bioprinting: A Scientometric Analysis of Two Decades of Progress
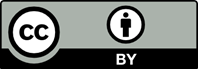
This scientometric analysis of 393 original papers published from January 2000 to June 2019 describes the development and use of bioinks for 3D bioprinting. The main trends for bioink applications and the primary considerations guiding the selection and design of current bioink components (i.e., cell types, hydrogels, and additives) were reviewed. The cost, availability, practicality, and basic biological considerations (e.g., cytocompatibility and cell attachment) are the most popular parameters guiding bioink use and development. Today, extrusion bioprinting is the most widely used bioprinting technique. The most reported use of bioinks is the generic characterization of bioink formulations or bioprinting technologies (32%), followed by cartilage bioprinting applications (16%). Similarly, the cell-type choice is mostly generic, as cells are typically used as models to assess bioink formulations or new bioprinting methodologies rather than to fabricate specific tissues. The cell-binding motif arginine-glycine-aspartate is the most common bioink additive. Many articles reported the development of advanced functional bioinks for specific biomedical applications; however, most bioinks remain the basic compositions that meet the simple criteria: Manufacturability and essential biological performance. Alginate and gelatin methacryloyl are the most popular hydrogels that meet these criteria. Our analysis suggests that present-day bioinks still represent a stage of emergence of bioprinting technology.
1. Rodríguez-Salvador M, Rio-Belver RM, Garechana-Anacabe G, 2017, Scientometric and Patentometric Analyses to Determine the Knowledge Landscape in Innovative Technologies: The Case of 3D Bioprinting. PLoS One, 12: e0180375 https://doi.org/10.1371/journal.pone.0180375
2. Rodríguez-Salvador M, Villarreal-Garza D, Álvarez MM, et al., 2019, Analysis of the Knowledge Landscape of Three dimensional Bioprinting in Latin America. Int J Bioprinting, 5:16–25. https://doi.org/10.18063/ijb.v5i2.3.240
3. Ng WL, Chua CK, Shen YF, 2019, Print Me An Organ! Why We Are Not There Yet. Prog Polym Sci, 97:101145. https://doi.org/10.1016/j.progpolymsci.2019.101145
4. Ravnic DJ, Leberfinger AN, Koduru SV, et al., 2017, Transplantation of Bioprinted Tissues and Organs: Technical and Clinical Challenges and Future Perspectives. Ann Surg, 266:48–58. https://doi.org/10.1097/SLA.0000000000002141
5. Singh AV, Ansari MHD, Wang S, et al., 2019, The Adoption of Three-dimensional Additive Manufacturing from Biomedical Material Design to 3D Organ Printing. Appl Sci, 9:811. https://doi.org/10.3390/app9040811
6. Murphy SV, Atala A, 2014, 3D Bioprinting of Tissues and Organs. Nat Biotechnol, 32:773–85. https://doi.org/10.1038/nbt.2958
7. Fang Y, Eglen RM, 2017, Three-Dimensional Cell Cultures in Drug Discovery and Development. SLAS Discov, 22:456–72. https://doi.org/10.1177/1087057117696795
8. Kačarević ŽP, Rider PM, Alkildani S, et al., 2018, An Introduction to 3D Bioprinting: Possibilities, Challenges and Future Aspects. Materials (Basel), 11:2199. https://doi.org/10.3390/ma11112199
9. Mobaraki M, Ghaffari M, Yazdanpanah A, et al., 2020, Bioinks and Bioprinting: A Focused Review. Bioprinting, 18:e00080. https://doi.org/10.1016/j.bprint.2020.e00080
10. Ashammakhi N, Ahadian S, Xu C, et al., 2019, Bioinks and Bioprinting Technologies to Make Heterogeneous and Biomimetic Tissue Constructs. Mater Today Bio, 1:100008. https://doi.org/10.1016/j.mtbio.2019.100008
11. Mironov V, Kasyanov V, Markwald RR, 2011, Organ Printing: From Bioprinter to Organ Biofabrication Line. Curr Opin Biotechnol, 22:667–73. https://doi.org/10.1016/j.copbio.2011.02.006
12. Bolívar-Monsalve EJ, Ceballos-González CF, Borrayo-Montaño KI, et al., 2021, Continuous Chaotic Bioprinting of Skeletal Muscle-like Constructs. Bioprinting, 21:e00125. https://doi.org/10.1016/j.bprint.2020.e00125
13. Choudhury D, Anand S, Naing MW, 2018, The Arrival of Commercial Bioprinters-Towards 3D Bioprinting Revolution! Int J Bioprinting, 4:1–20. https://doi.org/10.18063/IJB.v4i2.139
14. Wilson WC, Boland T, 2003, Cell and Organ Printing 1: Protein and Cell Printers. Anat Rec Part A Discov Mol Cell Evol Biol, 272:491–6. https://doi.org/10.1002/ar.a.10057
15. Ringeisen BR, Kim H, Barron JA, et al., 2004, Laser Printing of Pluripotent Embryonal Carcinoma Cells. Tissue Eng, 10:483–91. https://doi.org/10.1089/107632704323061843
16. Nakamura M, Kobayashi A, Takagi F, et al., 2005, Biocompatible Inkjet Printing Technique for Designed Seeding of Individual Living Cells. Tissue Eng, 11:1658–66. https://doi.org/0.1089/ten.2005.11.1658
17. Sun W, Starly B, Daly AC, et al., 2020, The Bioprinting Roadmap. Biofabrication, 12:022002. https://doi.org/10.1088/1758-5090/ab5158
18. Lee S, Sani ES, Spencer AR, et al., 2020, Human-Recombinant-Elastin-Based Bioinks for 3D Bioprinting of Vascularized Soft Tissues. Adv Mater, 32:1–10. https://doi.org/10.1002/adma.202003915
19. Kim SH, Yeon YK, Lee JM, et al., 2018, Precisely Printable and Biocompatible Silk Fibroin Bioink for Digital Light Processing 3D Printing. Nat Commun, 9:1620. https://doi.org/10.1038/s41467-018-03759-y
20. Zhang Z, Jin Y, Yin J, et al., 2018, Evaluation of Bioink Printability for Bioprinting Applications. Appl Phys Rev, 5:041304. https://doi.org/10.1063/1.5053979
21. Ruberu K, Senadeera M, Rana S, et al., 2021, Coupling Machine Learning with 3D Bioprinting to Fast Track Optimisation of Extrusion Printing. Appl Mater Today, 22:100914. https://doi.org/10.1016/j.apmt.2020.100914
22. Gu Y, Zhang L, Du X, et al., 2018 Reversible Physical Crosslinking Strategy with Optimal Temperature for 3D Bioprinting of Human Chondrocyte-laden Gelatin Methacryloyl Bioink. J Biomater Appl, 33:609–18. https://doi.org/10.1177/0885328218805864
23. Koo Y, Choi EJ, Lee J, et al., 2018, 3D Printed Cell-laden Collagen and Hybrid Scaffolds for In Vivo Articular Cartilage Tissue Regeneration. J Ind Eng Chem, 66:343–55. http://doi.org/10.1016/j.jiec.2018.05.049
24. Yang X, Lu Z, Wu H, et al., 2018, Collagen-alginate as Bioink for Three-Dimensional (3D) Cell Printing Based Cartilage Tissue Engineering. Mater Sci Eng C, 83:195–201. http://doi.org/10.1016/j.msec.2017.09.002
25. Shim JH, Lee JS, Kim JY, et al., 2012, Bioprinting of a Mechanically Enhanced Three-dimensional Dual Cell-laden Construct for Osteochondral Tissue Engineering Using a Multi-head Tissue/Organ Building System. J Micromech Microeng, 22:85014. https://doi.org/10.1088/0960-1317/22/8/085014
26. Lee JY, Koo Y, Kim G, 2018, Innovative Cryopreservation Process Using a Modified Core/Shell Cell-Printing with a Microfluidic System for Cell-Laden Scaffolds. ACS Appl Mater Interfaces, 10:9257–68. https://doi.org/10.1021/acsami.7b18360
27. Kim YB, Lee H, Kim GH, 2016, Strategy to Achieve Highly Porous/Biocompatible Macroscale Cell Blocks, Using a Collagen/Genipin-bioink and an Optimal 3D Printing Process. ACS Appl Mater Interfaces, 8:32230–40. https://doi.org/10.1021/acsami.6b11669
28. Baldwin P, Li DJ, Auston DA, et al., 2019, Autograft, Allograft, and Bone Graft Substitutes: Clinical Evidence and Indications for Use in the Setting of Orthopaedic Trauma Surgery. J Orthop Trauma, 33:203–13. https://doi.org/10.1097/BOT.0000000000001420
29. Frank RM, Cotter EJ, Hannon CP, et al., 2019, Cartilage Restoration Surgery: Incidence Rates, Complications, and Trends as Reported by the American Board of Orthopaedic Surgery Part II Candidates. Arthrosc J Arthrosc Relat Surg, 35:171–8. https://doi.org/10.1016/j.arthro.2018.08.028
30. Cartilage Repair Market Size, Share. Global Industry Report, 2025, n.d. viewed September 16, 2020. Available from: https://www.grandviewresearch.com/industry-analysis/cartilage-repair-regeneration-market. [Last accessed on 2020 Oct 20].
31. Levato R, Webb WR, Otto IA, et al., 2017, The Bio in the Ink: Cartilage Regeneration with Bioprintable Hydrogels and Articular Cartilage-derived Progenitor Cells. Acta Biomater, 61:41–53. https://doi.org/10.1016/j.actbio.2017.08.005
32. You F, Eames BF, Chen X, 2017, Application of Extrusion based Hydrogel Bioprinting for Cartilage Tissue Engineering. Int J Mol Sci, 18:8–14. https://doi.org/10.3390/ijms18071597
33. Shi W, Sun M, Hu X et al., 2017, Structurally and Functionally Optimized Silk-Fibroin-Gelatin Scaffold Using 3D Printing to Repair Cartilage Injury In Vitro and In Vivo. Adv Mater, 29:1–7. https://doi.org/10.1002/adma.201701089
34. Kilian D, Ahlfeld T, Akkineni AR, et al., 2020, 3D Bioprinting of Osteochondral Tissue Substitutes In Vitro-Chondrogenesis in Multi-Layered Mineralized Constructs. Sci Rep, 10:1–17. https://doi.org/10.1038/s41598-020-65050-9
35. Zhang H, Huang H, Hao G, et al., 2021, 3D Printing Hydrogel Scaffolds with Nanohydroxyapatite Gradient to Effectively Repair Osteochondral Defects in Rats. Adv Funct Mater, 31:1–13. https://doi.org/10.1002/adfm.202006697
36. Ashammakhi N, Hasan A, Kaarela O, et al., 2019, Advancing Frontiers in Bone Bioprinting. Adv Healthc Mater, 8:1801048. https://doi.org/10.1002/adhm.201801048
37. Byambaa B, Annabi N, Yue K, et al., 2017, Bioprinted Osteogenic and Vasculogenic Patterns for Engineering 3D Bone Tissue. Adv Healthc Mater, 6:1700015. https://doi.org/10.1002/adhm.201700015
38. Li L, Yu F, Shi J, et al., 2017, In Situ Repair of Bone and Cartilage Defects Using 3D Scanning and 3D Printing. Sci Rep, 7:1–12. https://doi.org/10.1038/s41598-017-10060-3
39. Cui X, Boland T, 2009, Human Microvasculature Fabrication Using Thermal Inkjet Printing Technology. Biomaterials, 30:6221–7. http://doi.org/10.1016/j.biomaterials.2009.07.056
40. Stratesteffen H, Köpf M, Kreimendahl F, et al., 2017, GelMA collagen Blends Enable Drop-on-demand 3D Printablility and Promote Angiogenesis. Biofabrication, 9:45002. https://doi.org/10.1088/1758-5090/aa857c
41. Jia W, Gungor-Ozkerim PS, Zhang YS, et al., 2016, Direct 3D Bioprinting of Perfusable Vascular Constructs Using a Blend Bioink. Biomaterials, 106:58–68. https://doi.org/10.1016/j.biomaterials.2016.07.038
42. Rouwkema J, Khademhosseini A, 2016, Vascularization and Angiogenesis in Tissue Engineering: Beyond Creating Static Networks. Trends Biotechnol, 34:733–45. https://doi.org/10.1016/j.tibtech.2016.03.002
43. Auger FA, Gibot L, Lacroix D, 2013, The Pivotal Role of Vascularization in Tissue Engineering. Annu Rev Biomed Eng, 15:177–200. https://doi.org/10.1146/annurev-bioeng-071812-152428
44. Suntornnond R, Tan EYS, An J, et al., 2017, A Highly Printable and Biocompatible Hydrogel Composite for Direct Printing of Soft and Perfusable Vasculature-Like Structures. Sci Rep, 7:1–11.
https://doi.org/10.1038/s41598-017-17198-0
45. Tomasina C, Bodet T, Mota C, et al., 2019, Bioprinting Vasculature: Materials, Cells and Emergent Techniques. Materials (Basel), 12:2701. https://doi.org/10.3390/ma12172701
46. Miri AK, Khalilpour A, Cecen B, et al., 2019, Multiscale Bioprinting of Vascularized Models. Biomaterials, 198:204–16. https://doi.org/10.1016/j.biomaterials.2018.08.006
47. Thomas A, Orellano I, Lam T, et al., 2020, Vascular Bioprinting with Enzymatically Degradable Bioinks Via Multi-material Projection-based Stereolithography. Acta Biomater, 117:121–32. https://doi.org/10.1016/j.actbio.2020.09.033.
48. Ouyang L, Armstrong JPK, Chen Q, et al., 2020,Void-Free 3D Bioprinting for In Situ Endothelialization and Microfluidic Perfusion. Adv Funct Mater, 30:1908349. https://doi.org/10.1002/adfm.201908349
49. Song KH, Highley CB, Rouff A, et al., 2018, Complex 3D-Printed Microchannels within Cell-Degradable Hydrogels. Adv Funct Mater, 28:1–10. https://doi.org/10.1002/adfm.201801331
50. Hakimi N, Cheng R, Leng L, et al., 2018, Handheld Skin Printer: In Situ Formation of Planar Biomaterials and Tissues. Lab Chip, 18:1440–51. https://doi.org/10.1039/C7LC01236E
51. Shamloo A, Aghababaie Z, Afjoul H, et al., 2021, Fabrication and Evaluation of Chitosan/Gelatin/PVA Hydrogel Incorporating Honey for Wound Healing Applications: An In Vitro, In Vivo Study. Int J Pharm, 592:120068. https://doi.org/10.1016/j.ijpharm.2020.120068
52. Yeo M, Kim G, 2018, Three-Dimensional Microfibrous Bundle Structure Fabricated Using an Electric Field-Assisted/Cell Printing Process for Muscle Tissue Regeneration. ACS Biomater Sci Eng, 4:728–38. https://doi.org/10.1021/acsbiomaterials.7b00983
53. Merceron TK, Burt M, Seol YJ, et al., 2015, A 3D Bioprinted Complex Structure for Engineering the Muscle-tendon Unit. Biofabrication, 7:35003. https://doi.org/10.1088/1758-5090/7/3/035003
54. Venus M, Waterman J, McNab I, 2010, Basic Physiology of the Skin. Surgery, 28:469–72. https://doi.org/10.1016/j.mpsur.2010.07.011
55. García-Lizarribar A, Fernández-Garibay X, Velasco-Mallorquí F, et al., 2018, Composite Biomaterials as Long-Lasting Scaffolds for 3D Bioprinting of Highly Aligned Muscle Tissue. Macromol Biosci, 18:1800167. http://doi.org/10.1002/mabi.201800167
56. Kim BS, Kwon YW, Kong JS, et al., 2018, 3D Cell Printing of In Vitro Stabilized Skin Model and In Vivo Pre-vascularized Skin Patch Using Tissue-specific Extracellular Matrix Bioink: A Step towards Advanced Skin Tissue Engineering. Biomaterials, 168:38–53. https://doi.org/10.1016/j.biomaterials.2018.03.040
57. Skardal A, Murphy SV, Crowell K, et al., 2017, A Tunable Hydrogel System for Long-term Release of Cell-secreted Cytokines and Bioprinted In Situ Wound Cell Delivery. J Biomed Mater Res Part B Appl Biomater, 105:1986–2000. https://doi.org/10.1002/jbm.b.33736
58. Jorgensen AM, Varkey M, Gorkun A, et al., 2020, Bioprinted Skin Recapitulates Normal Collagen Remodeling in Full-Thickness Wounds. Tissue Eng Part A, 26:512–26. https://doi.org/10.1089/ten.tea.2019.0319
59. Chen S, Nakamoto T, Kawazoe N, et al., 2015, Engineering Multi-layered Skeletal Muscle Tissue by Using 3D Microgrooved Collagen Scaffolds. Biomaterials, 73:23–31. https://doi.org/10.1016/j.biomaterials.2015.09.010
60. Mozetic P, Giannitelli SM, Gori M, et al., 2017, Engineering Muscle Cell Alignment through 3D Bioprinting. J Biomed Mater Res Part A, 105:2582–8. https://doi.org/10.1002/jbm.a.36117
61. Kim JH, Kim I, Seol YJ, et al., 2020, Neural Cell Integration into 3D Bioprinted Skeletal Muscle Constructs Accelerates Restoration of Muscle Function. Nat Commun, 11:1–12. https://doi.org/10.1038/s41467-020-14930-9
62. Baltazar T, Merola J, Catarino C, et al., 2020, Three Dimensional Bioprinting of a Vascularized and Perfusable Skin Graft Using Human Keratinocytes, Fibroblasts, Pericytes, and Endothelial Cells. Tissue Eng Part A, 26:227–38.
63. Ng WL, Qi JTZ, Yeong WY, et al., 2018, Proof-of-concept: 3D Bioprinting of Pigmented Human Skin Constructs. Biofabrication, 10:25005. https://doi.org/10.1088/1758-5090/aa9e1e
64. Kageyama T, Yan L, Shimizu A, et al., 2019, Preparation of Hair Beads and Hair Follicle Germs for Regenerative Medicine. Biomaterials, 212:55–63. https://doi.org/10.1016/j.biomaterials.2019.05.003
65. Frías-Sánchez A, Quevedo-Moreno D, Samandari M, et al., 2021, Biofabrication of Muscle Fibers Enhanced with Plant Viral Nanoparticles Using Surface Chaotic Flows. Biofabrication. 2021:abd9d7. https://doi.org/10.1088/1758-5090/abd9d7
66. Ceballos-González CF, Bolívar-Monsalve EJ, Quevedo-Moreno DA, et al., 2020, Micro-biogeography Greatly Matters for Competition: Continuous Chaotic Bioprinting of Spatially-controlled Bacterial Microcosms. BioRxiv, 2020:199307. https://doi.org/10.1101/2020.07.12.199307
67. Kim WJ, Lee H, Lee JU, et al, 2020, Efficient Myotube Formation in 3D Bioprinted Tissue Construct by Biochemical and Topographical Cues. Biomaterials, 230:119632. https://doi.org/10.1016/j.biomaterials.2019.119632
68. Organizacion Nacional de Transplantes, 2019, El Registro Mundial de Trasplantes cifra en 139.024 los Trasplantes Realizados en el Mundo en el Último año, con un Aumento del 2,3%, viewed October 1, 2020. Available from: http://www. ont.es/prensa/NotasDePrensa/28%2008%202019%20%20 REGISTRO%20MUNDIAL%20DE%20TRASPLANTES. pdf. [Last accessed on 2020 Oct 01].
69. Organ Procurement and Transplantation Network, 2020, National Data, viewed October 5, 2020. Available from: https://www.optn.transplant.hrsa.gov/data/view-data-reports/ national-data/#.
70. Murphy SV, De Coppi P, Atala A, 2020, Opportunities and Challenges of Translational 3D Bioprinting. Nat Biomed Eng, 4:370–80. https://doi.org/10.1038/s41551-019-0471-7
71. Mirdamadi E, Tashman JW, Shiwarski DJ, et al., 2020, FRESH 3D Bioprinting a Full-Size Model of the Human Heart. ACS Biomater Sci Eng, 6:6453–9. https://doi.org/10.1021/acsbiomaterials.0c01133
72. Turunen S, Kaisto S, Skovorodkin I, et al., 2018, 3D Bioprinting of the Kidney Hype or Hope? AIMS Cell Tissue Eng, 2:119–62. https://doi.org/10.3934/celltissue.2018.3.119
73. Ali M, Kumar A Pr., Yoo JJ, et al., 2019, A Photo-Crosslinkable Kidney ECM-Derived Bioink Accelerates Renal Tissue Formation. Adv Healthc Mater, 8:1800992. http://doi.org/10.1002/adhm.201800992
74. Irvine SA, Agrawal A, Lee BH, et al., 2015, Printing Cell laden Gelatin Constructs by Free-form Fabrication and Enzymatic Protein Crosslinking. Biomed Microdevices, 17:16. https://doi.org/10.1007/s10544-014-9915-8
75. Ozbolat IT, Hospodiuk M, 2016, Current Advances and Future Perspectives in Extrusion-based Bioprinting. Biomaterials, 76:321–43. https://doi.org/10.1016/j.biomaterials.2015.10.076
76. Gudapati H, Dey M, Ozbolat I, 2016, A Comprehensive Review on Droplet-based Bioprinting: Past, Present and Future. Biomaterials, 102:20–42. https://doi.org/10.1016/j.biomaterials.2016.06.012
77. Ng WL, Lee JM, Zhou M, et al., 2020, Vat Polymerization based Bioprinting-Process, Materials, Applications and Regulatory Challenges. Biofabrication, 12:22001. https://doi.org/10.1088/1758-5090/ab6034
78. Liu W, Zhang YS, Heinrich MA, et al., 2017, Rapid Continuous Multimaterial Extrusion Bioprinting. Adv Mater, 29:1–8. https://doi.org/10.1002/adma.201604630
79. Liu W, Zhong Z, Hu N, et al., 2018, Coaxial Extrusion Bioprinting of 3D Microfibrous Constructs with Cell favorable Gelatin Methacryloyl Microenvironments. Biofabrication, 10:024102. https://doi.org/10.1088/1758-5090/aa9d44
80. Yeo M, Ha J, Lee H, et al., 2016, Fabrication of hASCs-Laden Structures Using Extrusion-based Cell Printing Supplemented with an Electric Field. Acta Biomater, 38:33–43. http://doi.org/10.1016/j.actbio.2016.04.017
81. Campos DFD, Philip MA, Gürzing S, et al., 2019, Synchronized Dual Bioprinting of Bioinks and Biomaterial Inks as a Translational Strategy for Cartilage Tissue Engineering. 3D Print Addit Manuf. 6:63–71. https://doi.org/10.1089/3dp.2018.0123
82. Betsch M, Cristian C, Lin YY, et al., 2018, Incorporating 4D into Bioprinting: Real-Time Magnetically Directed Collagen Fiber Alignment for Generating Complex Multi layered Tissues. Adv Healthc Mater, 7:1800894. https://doi.org/10.1002/adhm.201800894
83. Köpf M, Campos DFD, Blaeser A, et al., 2016, A Tailored Three-dimensionally Printable Agarose-collagen Blend Allows Encapsulation, Spreading, and Attachment of Human Umbilical Artery Smooth Muscle Cells. Biofabrication, 8:25011. https://doi.org/10.1088/1758-5090/8/2/025011
84. Tetsuka H, Shin SR, 2020, Materials and Technical Innovations in 3D Printing in Biomedical Applications. J Mater Chem B, 8:2930–50. https://doi.org/10.1039/d0tb00034e
85. Hong J, Shin Y, Kim S, et al., 2019, Complex Tuning of Physical Properties of Hyperbranched Polyglycerol-Based Bioink for Microfabrication of Cell-Laden Hydrogels. Adv Funct Mater, 29:1808750. https://doi.org/10.1002/adfm.201808750
86. Lam T, Dehne T, Krüger JP, et al., 2019, Photopolymerizable Gelatin and Hyaluronic Acid for Stereolithographic 3D Bioprinting of Tissue-engineered Cartilage. J Biomed Mater Res Part B Appl Biomater, 107:2649–57. https://doi.org/10.1002/jbm.b.34354
87. Jung H, Min K, Jeon H, et al., 2016, Physically Transient Distributed Feedback Laser Using Optically Activated Silk Bio-Ink. Adv Opt, 4:1738–43. https://doi.org/10.1002/adom.201600369
88. Heinrich MA, Liu W, Jimenez A, et al., 2019, 3D Bioprinting: From Benches to Translational Applications. Small, 15:1–47. https://doi.org/10.1002/smll.201805510
89. Gao G, Huang Y, Schilling AF, et al., 2018, Organ Bioprinting: Are We There Yet? Adv Healthc Mater, 7:1–8. https://doi.org/10.1002/adhm.201701018
90. Badwaik R, 2019, 3D Printed Organs: The Future of Regenerative Medicine. J Clin Diagnostic Res, 13:13256. https://doi.org/10.7860/jcdr/2019/42546.13256
91. Paxton N, Smolan W, Böck T, et al., 2017, Proposal to Assess Printability of Bioinks for Extrusion-based Bioprinting and Evaluation of Rheological Properties Governing Bioprintability. Biofabrication, 9:44107. https://doi.org/10.1088/1758-5090/aa8dd8
92. Ding H, Chang RC, 2018, Printability Study of Bioprinted Tubular Structures Using Liquid Hydrogel Precursors in a Support Bath. Appl Sci, 8:403. https://doi.org/10.3390/app8030403
93. Diamantides N, Wang L, Pruiksma T, et al., 2017, Correlating Rheological Properties and Printability of Collagen Bioinks: The Effects of Riboflavin Photocrosslinking and pH. Biofabrication, 9:34102. https://doi.org/10.1088/1758-5090/aa780f
94. Bulanova EA, Koudan EV, Degosserie J, et al., 2017, Bioprinting of a Functional Vascularized Mouse Thyroid Gland Construct. Biofabrication, 9:34105. https://doi.org/10.1088/1758-5090/aa7fdd
95. Kiyotake EA, Douglas AW, Thomas EE, et al., 2019, Development and Quantitative Characterization of the Precursor Rheology of Hyaluronic Acid Hydrogels for Bioprinting. Acta Biomater, 95:176–87. https://doi.org/10.1016/j.actbio.2019.01.041
96. Zhang B, Luo Y, Ma L, et al., 2018, 3D Bioprinting: An Emerging Technology Full of Opportunities and Challenges. Biodesign Manuf, 1:2–13. https://doi.org/10.1007/s42242-018-0004-3
97. Hölzl K, Lin S, Tytgat L, et al., 2016, Bioink Properties Before, during and after 3D Bioprinting. Biofabrication, 8:32002. https://doi.org/10.1088/1758-5090/8/3/032002
98. Donderwinkel I, Van Hest JCM, Cameron NR, 2017, Bio-inks for 3D bioprinting: Recent advances and future prospects. Polym, 8(31):4451–71. https://doi.org/10.1039/c7py00826k
99. Li J, Chen M, Fan X, et al., 2016, Recent Advances in Bioprinting Techniques: Approaches, Applications and Future Prospects. J Transl Med, 14:1–15. https://doi.org/10.1186/s12967-016-1028-0
100. Rocca M, Fragasso A, Liu W, et al., 2018, Embedded Multimaterial Extrusion Bioprinting. SLAS Technol, 23:154–63. https://doi.org/10.1177/2472630317742071
101. Trujillo-De Santiago G, Alvarez MM, Samandari M, et al., 2018, Chaotic Printing: Using Chaos to Fabricate Densely Packed Micro-and Nanostructures at High Resolution and Speed. Mater Horizons, 5:813–22. https://doi.org/10.1039/c8mh00344k
102. Chávez-Madero C, Díaz de León-Derby M, Samandari M, et al., 2020, Using Chaotic Advection for Facile High throughput Fabrication of Ordered Multilayer Micro and Nanostructures: Continuous Chaotic Printing. Biofabrication, 12:35023. https://doi.org/10.1088/1758-5090/ab84cc
103. Gungor-Ozkerim PS, Inci I, Zhang YS, et al., 2018, Bioinks for 3D Bioprinting: an Overview. Biomater Sci, 6:915–46. https://doi.org/10.1039/c7bm00765e.Bioinks
104. Campos DFD, Zhang S, Kreimendahl F, et al., 2020, Handheld Bioprinting for De Novo Vascular Formation Applicable to Dental Pulp Regeneration. Connect Tissue Res, 61:205–15. https://doi.org/10.1080/03008207.2019.1640217
105. Di Bella C, Duchi S, O’Connell CD, et al., 2018, In Situ Handheld Three-dimensional Bioprinting for Cartilage Regeneration. J Tissue Eng Regen Med, 12:611–21. https://doi.org/10.1002/term.2476
106. Singh S, Choudhury D, Yu F, et al., 2020, In Situ Bioprinting Bioprinting from Bench side to Bedside? Acta Biomater, 101:14–25. https://doi.org/10.1016/j.actbio.2019.08.045
107. Albanna M, Binder KW, Murphy SV, et al., 2019, In Situ Bioprinting of Autologous Skin Cells Accelerates Wound Healing of Extensive Excisional Full-Thickness Wounds. Sci Rep, 9:1–15. https://doi.org/10.1038/s41598-018-38366-w
108. Russell CS, Mostafavi A, Quint JP, et al., 2020, In Situ Printing of Adhesive Hydrogel Scaffolds for the Treatment of Skeletal Muscle Injuries. ACS Appl Bio Mater, 3:1568–79. https://doi.org/10.1021/acsabm.9b01176
109. Bernal PN, Delrot P, Loterie D, et al., 2019, Volumetric Bioprinting of Complex Living-Tissue Constructs within Seconds. Adv Mater, 31:1904209. https://doi.org/10.1002/adma.201904209
110. Navarro J, Calderon GA, Miller JS, et al., editors, 2019, Bioinks for Three-Dimensional Printing in Regenerative Medicine. Academic Press, London, pp. 805–830.
111. Choi YJ, Jun YJ, Kim DY, et al., 2019, A 3D Cell Printed Muscle Construct with Tissue-derived Bioink for the Treatment of Volumetric Muscle Loss. Biomaterials, 206:160–9. https://doi.org/10.1016/j.biomaterials.2019.03.036
112. Chu DT, Nguyen TPT, Nguyen LBT, et al., 2019, Adipose Tissue Stem Cells for Therapy: An Update on the Progress of Isolation, Culture, Storage, and Clinical Application. J Clin Med, 8:917. https://doi.org/10.3390/jcm8070917
113. Xu W, Zhang XX, Yang P, et al., 2019, Alginate-honey Bioinks with Improved Cell Responses for Applications as Bioprinted Tissue Engineered Constructs. ACS Appl Mater Interfaces, 6:1–10. https://doi.org/10.1007/s40898-017-0003-8
114. Zhou F, Hong Y, Liang R, et al., 2020, Rapid Printing of Bio-inspired 3D Tissue Constructs for Skin Regeneration. Biomaterials, 258:120287. https://doi.org/10.1016/j.biomaterials.2020.120287
115. Kim H, Park MN, Kim J, et al., 2019, Characterization of Cornea-specific Bioink: High Transparency, Improved In Vivo Safety. J Tissue Eng, 10:2041731418823382. https://doi.org/10.1177/2041731418823382
116. Ouyang L, Yao R, Zhao Y, et al., 2016, Effect of Bioink Properties on Printability and Cell Viability for 3D Bioplotting of Embryonic Stem Cells. Biofabrication, 8:1–12. https://doi.org/10.1088/1758-5090/8/3/035020
117. Campos DFD, Blaeser A, Korsten A, et al., 2015, The Stiffness and Structure of Three-dimensional Printed Hydrogels Direct the Differentiation of Mesenchymal Stromal Cells toward Adipogenic and Osteogenic Lineages. Tissue Eng Part A, 21:740–56. https://doi.org/10.1089/ten.tea.2014.0231
118. Ma H, Zhou Q, Chang J, et al., 2019, Grape Seed-Inspired Smart Hydrogel Scaffolds for Melanoma Therapy and Wound Healing. ACS Nano, 13:4302–11. https://doi.org/10.1021/acsnano.8b09496
119. Park J, Lee SJ, Chung S, et al., 2017, Cell-laden 3D bioprinting hydrogel matrix depending on different compositions for soft tissue engineering: Characterization and evaluation. Mater Sci Eng C, 71:678–84. https://doi.org/10.1016/j.msec.2016.10.069
120. Zhu W, Cui H, Boualam B, et al., 2018, 3D Bioprinting Mesenchymal Stem Cell-laden Construct with Core shell Nanospheres for Cartilage Tissue Engineering. Nanotechnology, 29:185101. https://doi.org/10.1088/1361-6528/aaafa1
121. Huang S, Yao B, Xie J, et al., 2016, 3D Bioprinted Extracellular Matrix Mimics Facilitate Directed Differentiation of Epithelial Progenitors for Sweat Gland Regeneration. Acta Biomater, 32:170–7. https://doi.org/10.1016/j.actbio.2015.12.039
122. Gu Q, Tomaskovic-Crook E, Wallace GG, et al., 2017, 3D Bioprinting Human Induced Pluripotent Stem Cell Constructs for In Situ Cell Proliferation and Successive Multilineage Differentiation. Adv Healthc Mater, 6:1700175. https://doi.org/10.1002/adhm.201700175
123. Li Y, Jiang X, Li L, et al., 2018, 3D Printing Human Induced Pluripotent Stem Cells with Novel Hydroxypropyl Chitin Bioink: Scalable Expansion and Uniform Aggregation. Biofabrication, 10:44101. https://doi.org/10.1088/1758-5090/aacfc3
124. Choe G, Oh S, Seok JM, et al., 2019, Graphene Oxide/alginate Composites as Novel Bioinks for Three-dimensional Mesenchymal Stem Cell Printing and Bone Regeneration Applications. Nanoscale, 11:23275–85. https://doi.org/10.1039/c9nr07643c
125. Gonzalez-Fernandez T, Rathan S, Hobbs C, et al., 2019,Pore-forming bioinks to Enable Spatio-temporally Defined Gene Delivery in Bioprinted Tissues. J Control Release, 301:13–27. https://doi.org/10.1016/j.jconrel.2019.03.006
126. Sodupe-Ortega E, Sanz-Garcia A, Pernia-Espinoza A, et al., 2018, Accurate Calibration in Multi-material 3D Bioprinting for Tissue Engineering. Materials (Basel), 11:1–19. https://doi.org/10.3390/ma11081402
127. Liu J, Li L, Suo H, et al., 2019, 3D Printing of Biomimetic Multi-layered GelMA/nHA Scaffold for Osteochondral Defect Repair. Mater Des, 171:107708. https://doi.org/https://doi.org/10.1016/j.matdes.2019.107708
128. Admane P, Gupta AC, Jois P, et al., 2019, Direct 3D Bioprinted Full-thickness Skin Constructs Recapitulate Regulatory Signaling Pathways and Physiology of Human Skin. Bioprinting, 15:e00051. https://doi.org/10.1016/j.bprint.2019.e00051
129. Kwak H, Shin S, Lee H, et al., 2019, Formation of a Keratin Layer with Silk Fibroin-polyethylene Glycol Composite Hydrogel Fabricated by Digital Light Processing 3D Printing. J Ind Eng Chem, 72:232–40. https://doi.org/10.1016/j.jiec.2018.12.023
130. Pereira RF, Sousa A, Barrias CC, et al., 2018, A Single component Hydrogel Bioink for Bioprinting of Bioengineered 3D Constructs for Dermal Tissue Engineering. Mater Horizons, 5:1100–11. https://doi.org/10.1039/c8mh00525g
131. Gholami P, Ahmadi-Pajouh MA, Abolftahi N, et al., 2018, Segmentation and Measurement of Chronic Wounds for Bioprinting. IEEE J Biomed Health, 22:1269–77. https://doi.org/10.1109/JBHI.2017.2743526
132. Chen Y, Wang Y, Yang Q, et al., 2018, A Novel Thixotropic Magnesium Phosphate-based Bioink with Excellent Printability for Application in 3D Printing. J Mater Chem B, 6:4502–13. https://doi.org/10.1039/C8TB01196F
133. Zhu K, Chen N, Liu X, et al., 2019, A General Strategy for Extrusion Bioprinting of Bio-Macromolecular Bioinks through Alginate-Templated Dual-Stage Crosslinking. Macromol Biosci, 18:1800127. https://doi.org/10.1002/mabi.201800127
134. Cao X, Ashfaq R, Cheng F, et al., 2019, A Tumor-on-a-Chip System with Bioprinted Blood and Lymphatic Vessel Pair. Adv Funct Mater, 29:1807173. https://doi.org/10.1002/adfm.201807173
135. Sánchez-Salazar MG, Álvarez MM, Trujillo-de Santiago G, 2021, Advances in 3D Bioprinting for the Biofabrication of Tumor Models. Bioprinting, 21:e00120. https://doi.org/10.1016/j.bprint.2020.e00120
136. Lucey BP, Nelson-Rees WA, Hutchins GM, et al., 2009, Historical Perspective Henrietta Lacks, HeLa Cells, and Cell Culture Contamination. Arch Pathol Lab Med, 133:1463–7. https://doi.org/10.1043/1543-2165-133.9.1463
137. Saygili E, Dogan-Gurbuz AA, Yesil-Celiktas O, et al., 2020, 3D Bioprinting: A Powerful Tool to Leverage Tissue Engineering and Microbial Systems. Bioprinting, 18:e00071. https://doi.org/10.1016/j.bprint.2019.e00071
138. Schmieden DT, Vázquez SJB, Sangüesa H, et al., 2018, Printing of Patterned, Engineered E. coli Biofilms with a Low-Cost 3D Printer. ACS Synth Biol, 7:1328–37. https://doi.org/10.1021/acssynbio.7b00424
139. Ning E, Turnbull G, Clarke J, et al., 2019, 3D Bioprinting of Mature Bacterial Biofilms for Antimicrobial Resistance Drug Testing. Biofabrication, 11:045018. https://doi.org/10.1088/1758-5090/ab37a0
140. Huang Y, Xia A, Yang G, et al., 2018, Bioprinting Living Biofilms through Optogenetic Manipulation. ACS Synth Biol, 7:1195–200. https://doi.org/10.1021/acssynbio.8b00003
141. Balasubramanian S, Aubin-Tam ME, Meyer AS, 2019, 3D Printing for the Fabrication of Biofilm-Based Functional Living Materials. ACS Synth Biol, 8:1564–7. https://doi.org/10.1021/acssynbio.9b00192
142. Hynes WF, Chacón J, Segrè D, et al., 2018, Bioprinting Microbial Communities to Examine Interspecies Interactions in Time and Space. Biomed Phys Eng Express, 4:055010. https://doi.org/10.1088/2057-1976/aad544
143. Lode A, Krujatz F, Brüggemeier S, et al., 2015, Green Bioprinting: Fabrication of Photosynthetic Algae-laden Hydrogel Scaffolds for Biotechnological and Medical Applications. Eng Life Sci, 15:177–83. https://doi.org/10.1002/elsc.201400205
144. Maharjan S, Alva J, Cámara C, et al., 2021, Symbiotic Photosynthetic Oxygenation within 3D-Bioprinted Vascularized Tissues. Matter, 4:217–40. https://doi.org/10.1016/j.matt.2020.10.022
145. Cui X, Dean D, Ruggeri ZM, et al., 2010, Cell Damage Evaluation of Thermal Inkjet Printed Chinese Hamster Ovary Cells. Biotechnol Bioeng, 106:963–9. https://doi.org/10.1002/bit.22762
146. Ozler SB, Bakirci E, Kucukgul C, et al., 2017, Three dimensional Direct Cell Bioprinting for Tissue Engineering. J Biomed Mater Res Part B Appl Biomater, 105:2530–44. https://doi.org/10.1002/jbm.b.33768
147. Hoffman AS, 2012, Hydrogels for Biomedical Applications. Adv Drug Deliv Rev, 64:18–23. https://doi.org/10.1016/j.addr.2012.09.010
148. Chiew CSC, Poh PE, Pasbakhsh P, et al., 2014, Physicochemical Characterization of Halloysite/Alginate Bionanocomposite Hydrogel. Appl Clay Sci, 101:444–54. https://doi.org/10.1016/j.clay.2014.09.007
149. Xiong R, Zhang Z, Chai W, et al., 2015, Freeform Dropon-demand Laser Printing of 3D Alginate and Cellular Constructs. Biofabrication, 7:45011. https://doi.org/10.1088/1758-5090/7/4/045011
150. Freeman FE, Kelly DJ, 2017, Tuning Alginate Bioink Stiffness and Composition for Controlled Growth Factor Delivery and to Spatially Direct MSC Fate within Bioprinted Tissues. Sci Rep, 7:17042. https://doi.org/10.1038/s41598-017-17286-1
151. Jeon O, Lee YB, Hinton TJ, et al., 2019, Cryopreserved Cell laden Alginate Microgel Bioink for 3D Bioprinting of Living Tissues. Mater Today Chem, 12:61–70. https://doi.org/10.1016/j.mtchem.2018.11.009
152. Hiller T, Berg J, Elomaa L, et al., 2018, Generation of a 3D Liver Model Comprising Human Extracellular Matrix in an Alginate/Gelatin-Based Bioink by Extrusion Bioprinting for Infection and Transduction Studies. Int J Mol Sci, 19:1–17. https://doi.org/10.3390/ijms19103129
153. de Ruijter M, Ribeiro A, Dokter I, et al., 2019, Simultaneous Micropatterning of Fibrous Meshes and Bioinks for the Fabrication of Living Tissue Constructs. Adv Healthc Mater, 8:e1800418. https://doi.org/10.1002/adhm.201800418
154. Yue K, Trujillo-de Santiago G, Alvarez MM, et al., 2015, Synthesis, Properties, and Biomedical Applications of Gelatin Methacryloyl (GelMA) Hydrogels. Biomaterials, 73:254–71 https://doi.org/10.1016/j.biomaterials.2015.08.045
155. Lee BH, Lum N, Seow LY, et al., 2016, Synthesis and Characterization of Types A and B Gelatin Methacryloyl for Bioink Applications. Materials (Basel), 9:4–7. https://doi.org/10.3390/ma9100797
156. Chen X, Du W, Cai Z, et al., 2020, Uniaxial Stretching of Cell-Laden Microfibers for Promoting C2C12 Myoblasts Alignment and Myofibers Formation. ACS Appl Mater Interfaces, 12:2162–70. https://doi.org/10.1021/acsami.9b22103
157. Wang Z, Tian Z, Menard F, et al., 2017, Comparative Study of Gelatin Methacrylate Hydrogels from Different Sources for Biofabrication Applications. Biofabrication, 9:44101. https://doi.org/10.1088/1758-5090/aa83cf
158. Offengenden M, Chakrabarti S, Wu J, 2018, Chicken Collagen Hydrolysates Differentially Mediate Anti-inflammatory Activity and Type I Collagen Synthesis on Human Dermal Fibroblasts. Food Sci Hum Wellness, 7:138–47. https://doi.org/10.1016/j.fshw.2018.02.002
159. Lee J, Park CH, Kim CS, 2018, Microcylinder-laden Gelatin based Bioink Engineered for 3D Bioprinting. Mater Lett, 233:24–7. https://doi.org/10.1016/j.matlet.2018.08.138
160. Ying GL, Jiang N, Maharjan S, et al., 2018, Aqueous Two-Phase Emulsion Bioink-Enabled 3D Bioprinting of Porous Hydrogels. Adv Mater, 30:1805460. https://doi.org/10.1002/adma.201805460
161. Yin J, Yan M, Wang Y, et al., 2018, 3D Bioprinting of Low-Concentration Cell-Laden Gelatin Methacrylate (GelMA) Bioinks with a Two-Step Cross-linking Strategy. ACS Appl Mater Interfaces, 10:6849–57. https://doi.org/10.1021/acsami.7b16059
162. Na K, Shin S, Lee H, et al., Effect of Solution Viscosity on Retardation of Cell Sedimentation in DLP 3D Printing of Gelatin Methacrylate/Silk Fibroin Bioink. J Ind Eng Chem, 61:340–7. https://doi.org/10.1016/j.jiec.2017.12.032
163. Wang Z, Abdulla R, Parker B, et al., 2015, A Simple and High- Resolution Stereolithography-based 3D Bioprinting System Using Visible Light Crosslinkable Bioinks. Biofabrication, 7:45009. https://doi.org/10.1088/1758-5090/7/4/045009
164. Rutz AL, Hyland KE, Jakus AE, et al., 2015, A Multimaterial Bioink Method for 3D Printing Tunable, Cell-Compatible Hydrogels. Adv Mater, 27:1607–14. https://doi.org/10.1002/adma.201405076
165. Shin JH, Kang HW, 2018, The Development of Gelatin-Based Bio-Ink for Use in 3D Hybrid Bioprinting. Int J Precis Eng Manuf, 19:767–71. https://doi.org/10.1007/s12541-018-0092-1
166. Nichol JW, Koshy ST, Bae H, et al., 2010, Cell-laden Microengineered Gelatin Methacrylate Hydrogels. Biomaterials, 31:5536–44. https://doi.org/10.1016/j.biomaterials.2010.03.064
167. Peak CW, Stein J, Gold KA, et al., 2018, Nanoengineered Colloidal Inks for 3D Bioprinting. Langmuir, 34:917–25. https://doi.org/10.1021/acs.langmuir.7b02540
168. Nemir S, Hayenga HN, West JL, 2010, PEGDA Hydrogels with Patterned Elasticity: Novel Tools for the Study of Cell Response to Substrate Rigidity. Biotechnol Bioeng, 105:636–44. https://doi.org/10.1002/bit.22574
169. López-Marcial GR, Zeng AY, Osuna C, et al., 2018, Agarose-Based Hydrogels as Suitable Bioprinting Materials for Tissue Engineering. ACS Biomater Sci Eng, 4:3610–6. https://doi.org/10.1021/acsbiomaterials.8b00903
170. Abelseth E, Abelseth L, De la Vega L, et al., 2019, 3D Printing of Neural Tissues Derived from Human Induced Pluripotent Stem Cells Using a Fibrin-Based Bioink. ACS Biomater Sci Eng, 5:234–3. https://doi.org/10.1021/acsbiomaterials.8b01235
171. Cappelletti RM, 2012, Fibrinogen and Fibrin: Structure and Functional Aspects. J Thromb Haemost, 3:1894–904.
172. de Melo BAG, Jodat YA, Cruz EM, et al., 2020, Strategies to Use Fibrinogen as Bioink for 3D Bioprinting Fibrin-based Soft and Hard Tissues. Acta Biomater, 117:60–76. https://doi.org/10.1016/j.actbio.2020.09.024
173. Chameettachal S, Midha S, Ghosh S, 2016, Regulation of Chondrogenesis and Hypertrophy in Silk Fibroin-Gelatin-Based 3D Bioprinted Constructs. ACS Biomater Sci Eng, 2:1450–63. https://doi.org/10.1021/acsbiomaterials.6b00152
174. Rodriguez MJ, Dixon TA, Cohen E, et al., 2018, 3D Freeform Printing of Silk Fibroin. Acta Biomater, 71:379–87. https://doi.org/10.1016/j.actbio.2018.02.035
175. Chawla S, Midha S, Sharma A, et al., 2018, Silk-Based Bioinks for 3D Bioprinting. Adv Healthc Mater, 7:1–23. https://doi.org/10.1002/adhm.201701204
176. Kuss MA, Harms R, Wu S, et al., 2017, Short-term Hypoxic Preconditioning Promotes Prevascularization in 3D Bioprinted Bone Constructs with Stromal Vascular Fraction Derived Cells. RSC Adv, 7:29312–20. https://doi.org/10.1039/c7ra04372d
177. Mouser VHM, Abbadessa A, Levato R, et al., 2017, Development of a Thermosensitive HAMA-containing Bio-ink for the Fabrication of Composite Cartilage Repair Constructs. Biofabrication, 9:15026. https://doi.org/10.1088/1758-5090/aa6265
178. Ma K, Zhao T, Yang L, et al., 2020, Application of Robotic assisted In Situ 3D Printing in Cartilage Regeneration with HAMA Hydrogel: An In Vivo Study. J Adv Res, 23:123–32. https://doi.org/10.1016/j.jare.2020.01.010
179. Zhou M, Lee BH, Tan YJ, et al., 2019, Microbial Transglutaminase Induced Controlled Crosslinking of Gelatin Methacryloyl to Tailor Rheological Properties for 3D Printing. Biofabrication, 11:25011. https://doi.org/10.1088/1758-5090/ab063f
180. Salinas-Fernández S, Santos M, Alonso M, et al., 2020, Genetically Engineered Elastin-like Recombinamers with Sequence-based Molecular Stabilization as Advanced Bioinks for 3D Bioprinting. Appl Mater Today, 18:100500. https://doi.org/10.1016/j.apmt.2019.100500
181. Rinoldi C, Costantini M, Kijeńska-Gawrońska E, et al., 2019, Tendon Tissue Engineering: Effects of Mechanical and Biochemical Stimulation on Stem Cell Alignment on Cell-Laden Hydrogel Yarns. Adv Healthc Mater, 8(7):1801218. https://doi.org/10.1002/adhm.201801218
182. Swaminathan S, Hamid Q, Sun W, et al., 2019, Bioprinting of 3D Breast Epithelial Spheroids for Human Cancer Models. Biofabrication, 11:025003. https://doi.org/10.1088/1758-5090/aafc49
183. Gu Q, Tomaskovic-Crook E, Lozano R, et al., 2016, Functional 3D Neural Mini-Tissues from Printed Gel-Based Bioink and Human Neural Stem Cells. Adv Healthc Mater, 5:1429–38. https://doi.org/https://doi.org/10.1002/adhm.201600095
184. Zhang K, Fu Q, Yoo J, et al., 2017, 3D Bioprinting of Urethra with PCL/PLCL Blend and Dual Autologous Cells in Fibrin Hydrogel: An In Vitro Evaluation of Biomimetic Mechanical Property and Cell Growth Environment. Acta Biomater, 50:154–64. https://doi.org/10.1016/j.actbio.2016.12.008
185. Wang Y, Shi W, Kuss M, et al., 2018, 3D Bioprinting of Breast Cancer Models for Drug Resistance Study. ACS Biomater Sci Eng, 4:4401–11. https://doi.org/10.1021/acsbiomaterials.8b01277
186. Kuss M, Kim J, Qi D, et al., 2018, Effects of Tunable, 3D-bioprinted Hydrogels on Human Brown Adipocyte Behavior and Metabolic Function. Acta Biomater, 71:486–95. https://doi.org/10.1016/j.actbio.2018.03.021
187. Ebrahimi M, Ostrovidov S, Salehi S, et al., 2018, Enhanced Skeletal Muscle Formation on Microfluidic Spun Gelatin Methacryloyl (GelMA) Fibres Using Surface Patterning and Agrin Treatment. J Tissue Eng Regen Med, 12:2151–63. https://doi.org/10.1002/term.2738
188. Wang Y, Kankala RK, Zhu K, et al., 2019, Coaxial Extrusion of Tubular Tissue Constructs Using a Gelatin/GelMA Blend Bioink. ACS Biomater Sci Eng, 5:5514–24. https://doi.org/10.1021/acsbiomaterials.9b00926
189. Parthiban SP, Rana D, Jabbari E, et al., 2017, Covalently Immobilized VEGF-Mimicking Peptide with Gelatin Methacrylate Enhances Microvascularization of Endothelial Cells. Acta Biomater, 51:330–40. https://doi.org/10.1016/j.actbio.2017.01.046
190. Shao L, Gao Q, Zhao H, et al., 2018, Fiber-Based Mini Tissue with Morphology-Controllable GelMA Microfibers. Small, 14:1–8. https://doi.org/10.1002/smll.201802187
191. Lee VK, Kim DY, Ngo H, et al., 2014, Creating Perfused Functional Vascular Channels Using 3D Bio-printing Technology. Biomaterials, 35:8092–102. https://doi.org/10.1016/j.biomaterials.2014.05.083
192. Jia W, Gungor-Ozkerim PS, Zhang YS, et al., 2016, Direct 3D Bioprinting of Perfusable Vascular Constructs Using a Blend Bioink. Biomaterials, 106:58–68. https://doi.org/10.1016/j.biomaterials.2016.07.038
193. Shao L, Gao Q, Xie C, et al., 2020, Sacrificial Microgel-laden Bioink-enabled 3D Bioprinting of Mesoscale Pore Networks. Biodesign Manuf, 3:30–9. https://doi.org/10.1007/s42242-020-00062-y
194. Haring AP, Thompson EG, Tong Y, et al., 2019, Process-and Bio-inspired Hydrogels for 3D Bioprinting of Soft Freestanding Neural and Glial Tissues. Biofabrication, 11:25009. https://doi.org/10.1088/1758-5090/ab02c9
195. Saadati A, Hassanpour S, Hasanzadeh M, et al., 2019, Immunosensing of Breast Cancer Tumor Protein CA 15-3 (Carbohydrate Antigen 15.3) Using a Novel Nano-bioink: A New Platform for Screening of Proteins in Human Biofluids by Pen-on-paper Technology. Int J Biol Macromol, 132:748–58. https://doi.org/10.1016/j.ijbiomac.2019.03.170
196. Mokhtari H, Kharaziha M, Karimzadeh F, et al., 2019, An Injectable Mechanically Robust Hydrogel of Kappacarrageenan- dopamine Functionalized Graphene Oxide for Promoting Cell Growth. Carbohydr Polym, 214:234–49. https://doi.org/10.1016/j.carbpol.2019.03.030
197. Aldrich A, Kuss MA, Duan B, et al., 2019, 3D Bioprinted Scaffolds Containing Viable Macrophages and Antibiotics Promote Clearance of Staphylococcus aureus Craniotomy-Associated Biofilm Infection. ACS Appl Mater Interfaces, 11:12298–307. https://doi.org/10.1021/acsami.9b00264
198. Noh I, Kim N, Tran HN, et al., 2019, 3D Printable Hyaluronic Acid-based Hydrogel for its Potential Application as a Bioink in Tissue Engineering. Biomater Res, 23:3. https://doi.org/10.1186/s40824-018-0152-8
199. Arya N, Forget A, Sarem M, et al., 2019, RGDSP Functionalized Carboxylated Agarose as Extrudable Carriers for Chondrocyte Delivery. Mater Sci Eng C, 99:103–11. https://doi.org/10.1016/j.msec.2019.01.080
200. Ooi HW, Mota C, ten Cate AT, et al., 2018, Thiol-Ene Alginate Hydrogels as Versatile Bioinks for Bioprinting. Biomacromolecules, 19:3390–400. https://doi.org/10.1021/acs.biomac.8b00696
201. Kérourédan O, Bourget JM, Rémy M, et al., 2019, Micropatterning of Endothelial Cells to Create a Capillary like Network with Defined Architecture by Laser-assisted Bioprinting. J Mater Sci Mater Med, 30:28. https://doi.org/10.1007/s10856-019-6230-1
202. Miri AK, Nieto D, Iglesias L, et al., 2018, Microfluidics- Enabled Multimaterial Maskless Stereolithographic Bioprinting. Adv Mater, 30:1800242. https://doi.org/10.1002/adma.201800242
203. Jang J, Park HJ, Kim SW, et al., 2017, 3D Printed Complex Tissue Construct Using Stem Cell-laden Decellularized Extracellular Matrix Bioinks for Cardiac Repair. Biomaterials, 112:264–74. https://doi.org/10.1016/j.biomaterials.2016.10.026
204. Shin S, Kwak H, Hyun J, 2018, Melanin Nanoparticle-Incorporated Silk Fibroin Hydrogels for the Enhancement of Printing Resolution in 3D-Projection Stereolithography of Poly(ethylene glycol)-Tetraacrylate Bio-ink. ACS Appl Mater Interfaces, 10:23573–82. https://doi.org/10.1021/acsami.8b05963
205. Laternser S, Keller H, Leupin O, et al., 2018, A Novel Microplate 3D Bioprinting Platform for the Engineering of Muscle and Tendon Tissues. SLAS Technol Transl Life Sci Innov, 23:599–613. https://doi.org/10.1177/2472630318776594
206. Zhu K, Shin SR, van Kempen T, et al., 2017, Gold Nanocomposite Bioink for Printing 3D Cardiac Constructs. Adv Funct Mater, 27:1605352. https://doi.org/10.1002/adfm.201605352
207. Zhai X, Ruan C, Ma Y, et al., 2018, 3D-Bioprinted Osteoblast-Laden Nanocomposite Hydrogel Constructs with Induced Microenvironments Promote Cell Viability, Differentiation, and Osteogenesis both In Vitro and In Vivo. Adv Sci, 5:1700550. https://doi.org/10.1002/advs.201700550
208. Lee D, Lee D, Won Y, et al., 2016, Insertion of Vertically Aligned Nanowires into Living Cells by Inkjet Printing of Cells. Small, 12:1446–57. https://doi.org/10.1002/smll.201502510
209. Hashmi MU, Khan F, Khalid N, et al., 2017, Hydrogels Incorporated with Silver Nanocolloids Prepared from Antioxidant Rich Aerva javanica as Disruptive Agents against Burn Wound Infections. Colloids Surfaces A Physicochem Eng Asp, 529:475–86. https://doi.org/10.1016/j.colsurfa.2017.06.036
210. Maharjan B, Kumar D, Awasthi GP, et al., 2019, Synthesis and Characterization of Gold/Silica Hybrid Nanoparticles Incorporated Gelatin Methacrylate Conductive Hydrogels for H9C2 Cardiac Cell Compatibility Study. Compos Part B Eng, 177:107415. https://doi.org/10.1016/j.compositesb.2019.107415
211. Hasnidawani JN, Azlina HN, Norita H, et al., 2016, Synthesis of ZnO Nanostructures Using Sol-Gel Method. Procedia Chem, 19:211–6. https://doi.org/10.1016/j.proche.2016.03.095
212. Salavati-Niasari M, Soofivand F, Sobhani-Nasab A, et al., 2017, Facile Synthesis and Characterization of CdTiO3 Nanoparticles by Pechini Sol-gel Method. J Mater Sci Mater Electron, 28:14965–73. https://doi.org/10.1007/s10854-017-7369-5
213. Rahali K, Ben Messaoud G, Kahn CJF, et al., 2017, Synthesis and Characterization of Nano functionalized Gelatin Methacrylate Hydrogels. Int J Mol Sci, 18:2675. https://doi.org/10.3390/ijms18122675
214. Kusior A, Kollbek K, Kowalski K, et al., 2016, Sn and Cu Oxide Nanoparticles Deposited on TiO2 Nanoflower 3D Substrates by Inert Gas Condensation Technique. Appl Surf Sci, 380:193–202. https://doi.org/10.1016/j.apsusc.2016.01.204
215. Iqbal J, Abbasi BA, Ahmad R, et al., 2020, Biogenic Synthesis of Green and Cost Effective Iron Nanoparticles and Evaluation of their Potential Biomedical Properties. J Mol Struct, 1199:126979. https://doi.org/10.1016/j.molstruc.2019.126979
216. Nunes R, Baião A, Monteiro D, et al., 2020, Zein Nanoparticles as Low-cost, Safe, and Effective Carriers to Improve the Oral Bioavailability of Resveratrol. Drug Deliv Transl Res, 10:826–37. https://doi.org/10.1007/s13346-020-00738-z
217. Peak CW, Singh KA, Adlouni M, et al., 2019, Printing Therapeutic Proteins in 3D using Nanoengineered Bioink to Control and Direct Cell Migration. Adv Healthc Mater, 8:1801553. https://doi.org/10.1002/adhm.201801553
218. Chimene D, Peak CW, Gentry JL, et al., 2018, Nanoengineered Ionic-Covalent Entanglement (NICE) Bioinks for 3D Bioprinting. ACS Appl Mater Interfaces, 10:9957–68. https://doi.org/10.1021/acsami.7b19808
219. Wilson SA, Cross LM, Peak CW, et al., 2017, Shear-Thinning and Thermo-Reversible Nanoengineered Inks for 3D Bioprinting. ACS Appl Mater Interfaces, 9:43449–58. https://doi.org/10.1021/acsami.7b13602
220. Ahlfeld T, Cidonio G, Kilian D, et al., 2017, Development of a Clay Based Bioink for 3D Cell Printing for Skeletal Application. Biofabrication, 9:34103. https://doi.org/10.1088/1758-5090/aa7e96
221. Olsen TR, Casco M, Herbst A, et al., 2016, Longitudinal Stretching for Maturation of Vascular Tissues Using Magnetic Forces. Bioengineering, 3:29.
222. Ojansivu M, Rashad A, Ahlinder A, et al., 2019, Wood-based Nanocellulose and Bioactive Glass Modified Gelatin-alginate Bioinks for 3D Bioprinting of Bone Cells. Biofabrication, 11:35010. https://doi.org/10.1088/1758-5090/ab0692
223. Reakasame S, Trapani D, Detsch R, et al., 2018, Cell Laden Alginate-keratin Based Composite Microcapsules Containing Bioactive Glass for Tissue Engineering Applications. J Mater Sci Mater Med, 29:185. https://doi.org/10.1007/s10856-018-6195-5
224. Trampe E, Koren K, Akkineni AR, et al., 2018, Functionalized Bioink with Optical Sensor Nanoparticles for O2 Imaging in 3D-Bioprinted Constructs. Adv Funct Mater, 28:1804411. https://doi.org/10.1002/adfm.201804411
225. Allig S, Mayer M, Thielemann C. Workflow for Bioprinting of Cell-laden Bioink. Lek Tech, 48:46–51.
226. DeSimone E, Schacht K, Pellert A, et al., 2017, Recombinant Spider Silk-Based Bioinks. Biofabrication, 9:44104. https://doi.org/10.1088/1758-5090/aa90db
227. Echalier C, Levato R, Mateos-Timoneda MA, et al., 2017, Modular Bioink for 3D Printing of Biocompatible Hydrogels: Sol-gel Polymerization of Hybrid Peptides and Polymers. RSC Adv, 7:12231–35. https://doi.org/10.1039/C6RA28540F
228. Gao G, Zhang XF, Hubbell K, C et al., 2017, NR2F2 Regulates Chondrogenesis of Human Mesenchymal Stem Cells in Bioprinted Cartilage. Biotechnol Bioeng, 114:208–16. https://doi.org/10.1002/bit.26042
229. Daly AC, Cunniffe GM, Sathy BN, et al., 2016, 3D Bioprinting of Developmentally Inspired Templates for Whole Bone Organ Engineering. Adv Healthc Mater, 5:2353–62. https://doi.org/10.1002/adhm.201600182
230. Lee DY, Lee H, Kim Y, et al., 2016, Phage as Versatile Nanoink for Printing 3-D Cell-laden Scaffolds. Acta Biomater, 29:112–24. https://doi.org/10.1016/j.actbio.2015.10.004
231. Lozano R, Stevens L, Thompson BC, et al., 2015, 3D Printing of Layered Brain-like Structures Using Peptide Modified Gellan Gum Substrates. Biomaterials, 67:264–73. https://doi.org/10.1016/j.biomaterials.2015.07.022
232. Schacht K, Jüngst T, Schweinlin M, et al., 2015, Biofabrication of Cell-Loaded 3D Spider Silk Constructs. Angew Chemie Int Ed, 54:2816–20. https://doi.org/10.1002/anie.201409846
233. Jia J, Richards DJ, Pollard S, et al., 2014, Engineering Alginate as Bioink for Bioprinting. Acta Biomater, 10:4323–31. https://doi.org/10.1016/j.actbio.2014.06.034
234. Chung JHY, Naficy S, Yue Z, et al., 2013, Bio-ink Properties and Printability for Extrusion Printing Living Cells. Biomater Sci, 1:763–73. https://doi.org/10.1039/C3BM00012E
235. Poon YF, Cao Y, Liu Y, et al., 2010, Hydrogels Based on Dual Curable Chitosan-graft-Polyethylene Glycol graft-Methacrylate: Application to Layer-by-Layer Cell Encapsulation. ACS Appl Mater Interfaces, 2:2012–25. https://doi.org/10.1021/am1002876
236. Ji S, Almeida E, Guvendiren M, 2019, 3D Bioprinting of Complex Channels within Cell-laden Hydrogels. Acta Biomater, 95:214–24. https://doi.org/10.1016/j.actbio.2019.02.038
237. Park J, Lee SJ, Lee H, et al., 2018, Three Dimensional Cell Printing with Sulfated Alginate for Improved Bone Morphogenetic Protein-2 Delivery and Osteogenesis in Bone Tissue Engineering. Carbohydr Polym, 196:217–24. https://doi.org/10.1016/j.carbpol.2018.05.048
238. Luo Y, Luo G, Gelinsky M, et al., 2017, 3D Bioprinting Scaffold Using Alginate/Polyvinyl Alcohol Bioinks. Mater Lett, 189:295–8. https://doi.org/10.1016/j.matlet.2016.12.009
239. Poldervaart MT, Wang H, van der Stok J, et al., 2013, Sustained Release of BMP-2 in Bioprinted Alginate for Osteogenicity in Mice and Rats. PLoS One, 8:e72610. https://doi.org/10.1371/journal.pone.0072610.
240. Cunniffe GM, Gonzalez-Fernandez T, Daly A, et al., 2017, Three-Dimensional Bioprinting of Polycaprolactone Reinforced Gene Activated Bioinks for Bone Tissue Engineering. Tissue Eng Part A, 23:891–900. https://doi.org/10.1089/ten.tea.2016.0498
241. Rathan S, Dejob L, Schipani R, Haffner B, Möbius ME, Kelly DJ. Fiber Reinforced Cartilage ECM Functionalized Bioinks for Functional Cartilage Tissue Engineering. Adv Healthc Mater, 8:1801501.
242. Kesti M, Fisch P, Pensalfini M, et al., 2016, Guidelines for Standardization of Bioprinting: A Systematic Study of Process Parameters and their Effect on Bioprinted Structures. BioNanoMaterials, 17:193–204. https://doi.org/10.1515/bnm-2016-0004
243. Acosta-Vélez GF, Zhu TZ, Linsley CS, et al., 2018, Photocurable Poly(Ethylene Glycol) as a Bioink for the Inkjet 3D Pharming of Hydrophobic Drugs. Int J Pharm, 546:145–53. https://doi.org/10.1016/j.ijpharm.2018.04.056
244. Gao G, Lee JH, Jang J, et al., 2017, Tissue Engineered Bio-Blood-Vessels Constructed Using a Tissue-Specific Bioink and 3D Coaxial Cell Printing Technique: A Novel Therapy for Ischemic Disease. Adv Funct Mater, 27:1700798. https://doi.org/10.1002/adfm.201700798
245. Acosta-Vélez GF, Linsley CS, Craig MC, et al., 2017, Photocurable Bioink for the Inkjet 3D Pharming of Hydrophilic Drugs. Bioengineering, 4:11.
246. Erdem A, Darabi MA, Nasiri R, et al., 2020, 3D Bioprinting of Oxygenated Cell-Laden Gelatin Methacryloyl Constructs. Adv Healthc Mater, 9:1-12. https://doi.org/10.1002/adhm.201901794