Preheating of Gelatin Improves its Printability with Transglutaminase in Direct Ink Writing 3D Printing
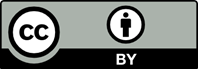
Gelatin and transglutaminase (TG) ink is increasingly popular in direct ink writing three-dimensional (3D) printing of cellular scaffolds and edible materials. The use of enzymes to crosslink gelatin chains removes the needs for toxic crosslinkers and bypasses undesired side reactions due to the specificity of the enzymes. However, their application in 3D printing remains challenging primarily due to the rapid crosslinking that leads to the short duration of printable time. In this work, we propose the use of gelatin preheated for 7 days to extend the duration of the printing time of the gelatin ink. We first determined the stiffness of freshly prepared gelatin (FG) and preheated gelatin (PG) (5 – 20% w/w) containing 5% w/w TG. We selected gelatin hydrogels made from 7.5% w/w FG and 10% w/w PG that yielded similar stiffness for subsequent studies to determine the duration of the printable time. PG inks exhibited longer time required for gelation and a smaller increase in viscosity with time than FG inks of similar stiffness. Our study suggested the advantage to preheat gelatin to enhance the printability of the ink, which is essential for extrusion-based bioprinting and food printing.
1. Ng WL, Chua CK, Shen YF, 2019, Print me an Organ! Why we are not there yet. Prog. Polym. Sci, 2019:101145. DOI:10.1016/j.progpolymsci.2019.101145.
2. Lee JM, Ng WL, Yeong WY, 2019, Resolution and Shape in Bioprinting: Strategizing Towards Complex Tissue and Organ Printing. Appl Phys Rev, 6:011307. DOI: 10.1063/1.5053909.
3. Guo SZ, Qiu K, Meng F, et al., 2017, 3D printed stretchable tactile sensors. Adv Mater, 29:1701218. DOI: 10.1002/adma.201701218.
4. Voon SL, An J, Wong G, et al., 2019, 3D Food Printing: A Categorised Review of Inks and their Development. Virtual Phys Prototyp, 14:203–18.
5. Karyappa R, Hashimoto M, 2019, Chocolate-based Ink Three-dimensional Printing (Ci3DP). Sci Rep, 9:1–11. DOI: 10.1038/s41598-019-50583-5.
6. Gariboldi MI, Best SM, 2015, Effect of Ceramic Scaffold Architectural Parameters on Biological Response. Front Bioeng Biotech, 3:151. DOI: 10.3389/fbioe.2015.00151.
7. Sun H, Zhu F, Hu Q, et al., 2014, Controlling Stem Cell mediated Bone Regeneration Through Tailored Mechanical Properties of Collagen Scaffolds. Biomaterials, 35:1176–84. DOI: 10.1016/j.biomaterials.2013.10.054.
8. Kuttappan S, Mathew D, Nair MB, 2016, Biomimetic Composite Scaffolds Containing Bioceramics and Collagen/Gelatin for Bone Tissue Engineering a Mini Review. Int J Biol Macromol, 93:1390–401. DOI: 10.1016/j.ijbiomac.2016.06.043.
9. Irvine SA, Agrawal A, Lee BH, et al., 2015, Printing Cell laden Gelatin Constructs by Free-form Fabrication and Enzymatic Protein Crosslinking. Biomed Microdevices, 17:16. DOI: 10.1007/s10544-014-9915-8.
10. Bettadapur A, Suh GC, Geisse NA, et al., 2016, Prolonged Culture of Aligned Skeletal Myotubes on Micromolded Gelatin Hydrogels. Sci Rep, 6:28855. DOI: 10.1038/srep28855.
11. Osorio FA, Bilbao E, Bustos R, et al., 2007, Effects of Concentration, Bloom Degree, and pH on Gelatin Melting and Gelling Temperatures Using Small Amplitude Oscillatory Rheology. Int J Food Prop, 10:841–51. DOI: 10.1080/10942910601128895.
12. Pepelanova I, Kruppa K, Scheper T, et al., 2018, Gelatin- Methacryloyl (GelMA) Hydrogels with Defined Degree of Functionalization as a Versatile Toolkit for 3D Cell Culture and Extrusion Bioprinting. Bioengineering (Basel), 5:55. DOI: 10.3390/bioengineering5030055.
13. Karyappa R, Ching T, Hashimoto M, 2020, Embedded Ink Writing (EIW) of Polysiloxane Inks. ACS Appl Mater Inter, 12:23565–75. DOI: 10.1021/acsami.0c03011.
14. Karyappa R, Ohno A, Hashimoto M, 2019, Immersion Precipitation 3D Printing (ip 3DP). Mater Horiz, 6:1834–44. DOI: 10.1039/c9mh00730j.
15. Melocchi A, Parietti F, Maroni A, et al., 2016, Hot-melt Extruded Filaments Based on Pharmaceutical Grade Polymers for 3D Printing by Fused Deposition Modeling. Int J Pharm, 509:255–63. DOI: 10.1016/j.ijpharm.2016.05.036.
16. Ghazal AF, Zhang M, Liu Z, 2019, Spontaneous Color Change of 3D Printed Healthy Food Product over Time after Printing as a Novel Application for 4D Food Printing. Food Bioproc Tech., 12:1627–45. DOI: 10.1007/s11947-019-02327-6.
17. Dhariwala B, Hunt E, Boland T, 2004, Rapid Prototyping of Tissue-engineering Constructs, Using Photopolymerizable Hydrogels and Stereolithography. Tissue Eng, 10:1316–22. DOI: 10.1089/1076327042500256.
18. Skardal A, Zhang J, McCoard L, et al., 2010, Photocrosslinkable Hyaluronan-gelatin Hydrogels for Two-step Bioprinting. Tissue Eng Part A, 16:2675–85. DOI: 10.1089/ten.tea.2009.0798.
19. Billiet T, Gevaert E, De Schryver T, et al., 2014, The 3D Printing of Gelatin Methacrylamide Cell-laden Tissue engineered Constructs with High Cell Viability. Biomaterials, 35: 49–62. DOI: 10.1016/j.biomaterials.2013.09.078.
20. Duan B, Hockaday LA, Kang KH, et al., 2013, 3D Bioprinting of Heterogeneous Aortic Valve Conduits with Alginate/Gelatin Hydrogels. J Biomed Mater ResA, 101:1255–64. DOI: 10.1002/jbm.a.34420.
21. Kirchmajer DM, Iii RG, 2015, An Overview of the Suitability of Hydrogel-forming Polymers for Extrusion-based 3D-printing. J Mater Chem B, 3:4105–17. DOI: 10.1039/c5tb00393h.
22. Chang R, Nam J, Sun W, 2008, Effects of Dispensing Pressure and Nozzle Diameter on Cell Survival from Solid Freeform Fabrication Based Direct Cell Writing. Tissue Eng Part A, 14:41–8. DOI: 10.1089/ten.2007.0004.
23. Mondal MI, 2019, Cellulose-Based Superabsorbent Hydrogels. Springer, Berlin, Germany.
24. Tice L, Moore A, 1952, Heat Denatured Gelatin. J Am Pharm Assoc, 41:631–3.
25. Qi J, Zhang WW, Feng XC, et al., 2018, Thermal Degradation of Gelatin Enhances its Ability to Bind Aroma Compounds: Investigation of Underlying Mechanisms. Food Hydrocoll, 83:497–510. DOI: 10.1016/j.foodhyd.2018.03.021.
26. Xing Q, Yates K, Vogt C, et al., 2014, Increasing Mechanical Strength of Gelatin Hydrogels by Divalent Metal Ion Removal. Sci Rep, 4:4706. DOI: 10.1038/srep04706.
27. Janmey PA, Miller RT, 2011, Mechanisms of Mechanical Signaling in Development and Disease. J Cell Sci, 124:9–18.
28. Solon J, Levental I, Sengupta K, et al., 2007, Fibroblast Adaptation and Stiffness Matching to Soft Elastic Substrates. Biophys J, 93:4453–61. DOI: 10.1529/biophysj.106.101386.
29. Mogha P, Srivastava A, Kumar S, et al., 2019, Hydrogel Scaffold with Substrate Elasticity Mimicking Physiological niche Promotes Proliferation of Functional Keratinocytes. RSC Adv, 9:10174–83. DOI: 10.1039/c9ra00781d.
30. Chan SW, Rizwan M, Yim EK, 2020, Emerging Methods for Enhancing Pluripotent Stem Cell Expansion. Front Cell Dev Biol, 8:70. DOI: 10.3389/fcell.2020.00070.
31. Tan JJ, Tee JK, Chou KO, et al., 2018, Impact of Substrate Stiffness on Dermal Papilla Aggregates in Microgels. Biomater Sci, 6:1347–57.
32. Croome R, 1959, The Rapid Formation and Breakdown of Gelatin Gels, and the Temperature‐dependence of their Rigidity. J Sci Food Agric, 10:394–400. DOI: 10.1002/jsfa.2740100708.
33. Gerardo H, Lima A, Carvalho J, et al., 2019, Soft Culture Substrates Favor Stem-like Cellular Phenotype and Facilitate Reprogramming of Human Mesenchymal Stem/Stromal Cells (hMSCs) through Mechanotransduction. Sci Rep, 9:1–18. DOI: 10.1038/s41598-019-45352-3.
34. Kureel SK, Mogha P, Khadpekar A, et al., 2019, Soft Substrate Maintains Proliferative and Adipogenic Differentiation Potential of Human Mesenchymal Stem Cells on Long-term Expansion by Delaying Senescence. Biol Open, 8:bio039453.DOI: 10.1101/364059.
35. Gilbert PM, Havenstrite KL, Magnusson KE, et al., 2010, Substrate Elasticity Regulates Skeletal Muscle Stem Cell Self-renewal in Culture. Science, 329:1078–81. DOI: 10.1126/science.1191035.
36. Ouyang L, Yao R, Zhao Y, et al., 2016, Effect of Bioink Properties on Printability and Cell Viability for 3D Bioplotting of Embryonic Stem Cells. Biofabrication, 8:035020. DOI: 10.1088/1758-5090/8/3/035020.
37. Nauenberg M, 2014, A Paradox with the Hagen-Poiseuille Relation for Viscous Fluid Flow. Am J Phys, 82:82–5. DOI: 10.1119/1.4825138.
38. Mulder M, 2000, Membrane preparation. In: Encyclopedia of Separation Science: Phase Inversion Membranes. Elsevier, Amsterdam, Netherlands, pp. 3331–3346. DOI: 10.1016/b0-12-226770-2/05271-6.
39. Zhu S, Stieger MA, van der Goot AJ, et al., 2019, Extrusion based 3D Printing of Food Pastes: Correlating Rheological Properties with Printing Behaviour. Innov Food Sci Emerg, 58:102214. DOI: 10.1016/j.ifset.2019.102214.
40. Schneider CA, Rasband WS, Eliceiri KW, 2012, NIH Image to ImageJ: 25 Years of Image Analysis. Nat Methods, 9:671–5. DOI: 10.1038/nmeth.2089.