3D Bioprinting: The Roller Coaster Ride to Commercialization
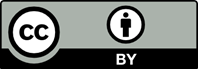
Three-dimensional (3D) bioprinting as a technology is being researched and applied since 2003. It is actually several technologies (inkjet, extrusion, laser, magnetic bioprinting, etc.) under an umbrella term “3D bioprinting.” The versatility of this technology allows widespread applications in several; however, after almost 20 years of research, there is still a limited number of cases of commercialized applications. This article discusses the potential for 3D bioprinting in regenerative medicine, drug discovery, and food industry, as well as the existing cases of companies that create commercialized products and services in the aforementioned areas and even in fashion, including their go-to-market route and financing received. We also address the main barriers to creating practical applications of 3D bioprinting within each sphere the technology that is being studied for.
1. O’Brien CM, Holmes B, Faucett S, et al., 2015, Three-Dimensional Printing of Nanomaterial Scaffolds for Complex Tissue Regeneration. Tissue Eng, 21:103–14. DOI: 10.1089/ten.teb.2014.0168.
2. Wilson WC, Boland T, 2003, Cell and Organ Printing 1: Protein and Cell Printers. Anat Rec, 272a:491–6. DOI: 10.1002/ar.a.10057.
3. Mironov V, Boland T, Trusk T, 2003, Organ Printing: Computer aided Jet-based 3D Tissue Engineering. Trends Biotechnol, 21:157–61. DOI: 10.1016/s0167-7799(03)00033-7.
4. Dababneh AB, Ozbolat IT, 2014, Bioprinting Technology: A Current State-of-the-Art Review. J Manuf Sci Eng, 136:61.
5. Duan B, Hockaday LA, Kang KH, Butcher JT, 2013, Bioprinting of Heterogeneous Aortic Valve Conduits with Alginate/Gelatin Hydrogels. J Biomed Mater Res, 101A:1255–64. DOI: 10.1002/jbm.a.34420.
6. Wang S, Lee JM, Yeong WY, 2007, Smart Hydrogels for 3D Bioprinting. J Bioprinting, 1:3–14. DOI: 10.18063/ijb.2015.01.005.
7. Campbell PG, Weiss LE, 2007, Tissue Engineering with the Aid of Inkjet Printers. Expert Opin Biol Ther, 7:1123–7.
8. Skardal A, Mack D, Kapetanovic E, 2012, Bioprinted Amniotic Fluid-Derived Stem Cells Accelerate Healing of Large Skin Wounds. Stem Cells Transl Med, 1:792–802. DOI: 10.5966/sctm.2012-0088.
9. Keriquel V, Guillemot F, Arnault I, 2010, In Vivo Bioprinting for Computer and Robotic-assisted Medical Intervention: Preliminary Study in Mice. Biofabrication, 2:14. DOI: 10.1088/1758-5082/2/1/014101.
10. Poietis Signs a Clinical Research Collaboration Contract with the Assistance Publique Hôpitaux de Marseille (APHM) to Prepare the First Clinical Trial of a Bioprinted Skin. Available from: https://www.poietis.com/poietis-signs-a clinical-research-collaboration-contract-with-the assistance publique-hopitaux-de-marseille-ap-hm-to-prepare-thefirst-clinical-trial-of-a-bioprinted-skin. DOI: 10.1016/j.tracli.2009.12.003.
11. Sen CK, Gordillo GM, Roy S, 2017, Human skin wounds: A major and snowballing threat to public health and the economy. Wound Repair Regen, 17:763–71. DOI: 10.1111/j.1524-475x.2009.00543.x.
12. Albanna M, Binder KM, Murphy S, et al., 2019, In Situ Bioprinting of Autologous Skin Cells Accelerates Wound Healing of Extensive Excisional Full Thickness Wounds. Sci Rep, 9:1856. DOI: 10.1038/s41598-018-38366-w.
13. Beckrich K, Aronovitch SA, 1999, Hospital-Acquired Pressure Ulcers: A Comparison of Costs in Medical vs.Surgical Patients. Nurs Econ, 17:263–71.
14. Miller SF, Bessey P, Lentz CW, et al., 2008, National Burn Repository 2007 Report: A Synopsis of the 2007 Call for Data. J Burn Care Res, 29:862–70. DOI: 10.1097/bcr.0b013e31818cb046.
15. Thomas SJ, Kramer GC, Herndon DN, 2003, Burns: Military Options and Tactical Solutions. J Trauma, 54:207–18.
16. nScrypt and Taking Bioprinting to the Field. Available from: https://www.3dprintingmedia.network/bioprinting-todayseries-nscrypt. [Last accessed on 2020 Jul 27].
17. Balakhovsky Y, Ostrovskiy A, Khesuani Y, 2017, Emerging business models toward commercialization of bioprinting technology. In: 3D Printing and Biofabrication. Springer, Berlin, pp. 1–22. DOI: 10.1007/978-3-319-40498-1_25-1.
18. Available from: https://www.allevi3d.com/allevi-bioprintingin-space. [Last accessed on 2020 Jul 27].
19. Ito A, Ino K, Hayashida M, 2005, Novel Methodology for Fabrication of Tissue-Engineered Tubular Constructs Using Magnetite Nanoparticles and Magnetic Force. Tissue Eng, 11:1553–61. DOI: 10.1089/ten.2005.11.1553.
20. Luciani N, Du V, Gazeau F, et al., 2016, Successful Chondrogenesis within Scaffolds, Using Magnetic Stem Cell Confinement and Bioreactor Maturation. Acta Biomater, 37:101–10. DOI: 10.1016/j.actbio.2016.04.009.
21. Whatley BR, Li X, Zhang N, et al., 2014, Magnetic-Directed Patterning of Cell Spheroids. J Biomed Mater Res A, 102:1537–47. DOI: 10.1002/jbm.a.34797.
22. Mazuel F, Espinosa A, Luciani N, 2016, Massive Intracellular Biodegradation of Iron Oxide Nanoparticles Evidenced Magnetically at Single-Endosome and Tissue Levels. ACS Nano, 8:7627–38. DOI: 10.1021/acsnano.6b02876.
23. Tasoglu S, Yu CH, Liaudanskaya V, et al., 2015, Magnetic Levitational Assembly for Living Material Fabrication. Adv Healthc Mater, 4:1469–76. DOI: 10.1002/adhm.201500092.
24. Durmus NG, Tekin HC, Guven S, 2015, Magnetic Levitation of Single Cells. Proc Natl Acad Sci, 112:3661–8. DOI: 10.1073/pnas.1509250112.
25. Mirica KA, Ilievski F, Ellerbee AK, et al., 2011, Using Magnetic Levitation for Three Dimensional Self-Assembly. Adv Mater, 23:4134–40. DOI: 10.1002/adma.201101917.
26. Subramaniam AB, Yang D, Yu HD, et al., 2014, Noncontact Orientation of Objects in Three dimensional Space Using Magnetic Levitation. Proc Natl Acad Sci, 111:12980–85. DOI: 10.1073/pnas.1408705111.
27. Guo F, Mao Z, Chen Y, et al., 2016, Three-Dimensional Manipulation of Single Cells Using Surface Acoustic Waves. Proc Natl Acad Sci, 113:1522–7. DOI: 10.1073/pnas.1524813113.
28. Guo F, Li P, French JB, et al., 2015, Controlling Cell-Cell Interactions Using Surface Acoustic Waves. Proc Natl Acad Sci, 112:43–8. DOI: 10.1073/pnas.1422068112.
29. Ding X, Li P, Lin SC, et al., 2013, Surface Acoustic Wave Microfluidics. Lab Chip, 18:3626–49.
30. Li P, Mao Z, Peng Z, et al., 2015, Acoustic Separation of Circulating Tumor Cells. Proc Natl Acad Sci, 112:4970–5. DOI: 10.1073/pnas.1504484112.
31. Sapozhnikov OA, Bailey MR, 2013, Radiation Force of an Arbitrary Acoustic Beam on an Elastic Sphere in a Fluid. J Acoust Soc Am, 133:661–76. DOI: 10.1121/1.4773924.
32. Friend J, Yeo LY, 2011, Microscale Acoustofluidics: Microfluidics Driven via Acoustics and Ultrasonics. Rev Mod Phys, 83:647–704. DOI: 10.1103/revmodphys.83.647.
33. Zhou Y, 2016, The Application of Ultrasound in 3D Bio-Printing. Molecules, 21:590.
34. Freed LA, Langer R, Martin I, et al., 1997, Tissue Engineering of Cartilage in Space. Natl Acad Sci USA, 94:13885–90. DOI: 10.1073/pnas.94.25.13885.
35. Lamb J, 2016, Scurvy: The Disease of Discovery.
36. Peng W, Unutmaz D, Ozbolat IT, 2016, Bioprinting towards Physiologically Relevant Tissue Models for Pharmaceutics. Trends Biotechnol, 34:722–32. DOI: 10.1016/j.tibtech.2016.05.013.
37. Ozbolat IT, Peng W, Ozbolat V, 2016, Application Areas of 3D Bioprinting. Drug Discov Today, 21:1257–71. DOI: 10.1016/j.drudis.2016.04.006.
38. Peng W, Datta P, Ayan B, et al., 2017, 3D Bioprinting for Drug Discovery and Development in Pharmaceutics. Acta Biomater, 57:26–46.
39. Peng W, Datta P, Wu Y, et al., 2018, Challenges in Bio-fabrication of Organoid Cultures. Adv Exp Med Biol, 1107:53-71.
40. Ozbolat IT, Yin Y, 2013, Bioprinting toward Organ Fabrication: Challenges and Future Trends. IEEE Trans Biomed Eng, 60:691–9. DOI: 10.1109/tbme.2013.2243912.
41. Haisler WL, Timm DM, Gage JA, et al., 2013, Three-Dimensional Cell Culturing by Magnetic Levitation. Nat Protoc, 8:1940–9. DOI: 10.1038/nprot.2013.125.
42. De Moor L, Merovci I, Baetens S, et al., 2018, High throughput Fabrication of Vascularized Spheroids for Bioprinting. Biofabrication, 10:035009. DOI: 10.1088/1758-5090/aac7e6.
43. Ling K, Huang G, Liu J, et al., 2015, Bioprinting-Based High-Throughput Fabrication of Three- Dimensional MCF-7 Human Breast Cancer Cellular Spheroids. Engineering, 1:269–74. DOI: 10.15302/j-eng-2015062.
44. Mordwinkin NM, Lee AS, Wu JC, 2013, Patient-Specific Stem Cells and Cardiovascular Drug Discovery. JAMA, 310:39–40. DOI: 10.1001/jama.2013.282409.
45. Ma X, Liu J, Zhu W, et al., 2018, 3D Bioprinting of Functional Tissue Models for Personalized Drug Screening and In Vitro Disease Modeling. Adv Drug Deliv Rev, 132:235–51.
46. Satpathy A, Datta P, Wu Y, et al., 2018, Developments with 3D Bioprinting for Novel Drug Discovery. Expert Opin Drug Discov, 13:1115–29. DOI: 10.1080/17460441.2018.1542427.
47. Nguyen DG, Funk J, Robbins JB, et al., 2016, Bioprinted 3D Primary Liver Tissues Allow Assessment of Organ-Level Response to Clinical Drug Induced Toxicity In Vitro. PLoS One,11:e0158674. DOI: 10.1371/journal.pone.0158674.
48. Massa S, Sakr MA, Seo J, et al., 2017, Bioprinted 3D Vascularized Tissue Model for Drug Toxicity Analysis. Biomicrofluidics, 11:044109.
49. Сhang R, Emami K, Wu H, et al., 2010, Biofabrication of a Three-Dimensional Liver Micro-Organ as an In Vitro Drug Metabolism Model. Biofabrication, 2:045004. DOI: 10.1088/1758-5082/2/4/045004.
50. Snyder JE, Hamid Q, Wang C, et al., 2011, Bioprinting Cell-laden Matrigel for Radioprotection Study of Liver by Pro-drug Conversion in a Dual-tissue Microfluidic Chip. Biofabrication, 3:034112. DOI: 10.1088/1758- 5082/3/3/034112.
51. Ji S, Guvendiren M, 2017, Recent Advances in Bioink Design for 3D Bioprinting of Tissues and Organs. Front Bioeng Biotechnol, 5:23. DOI: 10.3389/fbioe.2017.00023.
52. Hong S, Lee JY, Hwang C, et al., 2016, Inhibition of Rho-Associated Protein Kinase Increases the Angiogenic Potential of Mesenchymal Stem Cell Aggregates via Paracrine Effects. Tissue Eng Part A, 22:233–43. DOI: 10.1089/ten.tea.2015.0289.
53. Hong S, Song SJ, Lee JY, et al., 2013, Cellular Behavior in Micropatterned Hydrogels by Bioprinting System Depended on the Cell Types and Cellular Interaction. J Biosci Bioeng, 116:224–30. DOI: 10.1016/j.jbiosc.2013.02.011.
54. Horvath L, Umehara Y, Jud C, et al., 2015, Engineering an In Vitro Air-Blood Barrier by 3D Bioprinting. Sci Rep, 5:7974. DOI: 10.1038/srep07974.
55. Isaacson A, Swioklo S, Connon CJ, 2018, 3D Bioprinting of a Corneal Stroma Equivalent. Exp Eye Res, 173:188–93. DOI: 10.1016/j.exer.2018.05.010.
56. Madden LR, Nguyen TV, Garcia-Mojica S, et al., 2018, Bioprinted 3D Primary Human Intestinal Tissues Model Aspects of Native Physiology and ADME/Tox Functions. iScience, 2:156–67. DOI: 10.1016/j.exer.2018.05.010.
57. Homan KA, Kolesky DB, Skylar-Scott MA, et al., 2016, Bioprinting of 3D Convoluted Renal Proximal Tubules on Perfusable Chips. Sci Rep, 6:34845. DOI: 10.1038/srep34845.
58. Mozetic P, Giannitelli SM, Gori M, et al., 2017, Engineering Muscle Cell Alignment through 3D Bioprinting. J Biomed Mater Res A, 105:2582–8. DOI: 10.1002/jbm.a.36117.
59. Agrawal G, Aung A, Varghese S, 2017, Skeletal Muscle-ona-Chip: An In Vitro Model to Evaluate Tissue Formation and Injury. Lab Chip, 17:3447–61. DOI: 10.1039/c7lc00512a.
60. Wang Z, Lee SJ, Cheng HJ, et al., 2018, 3D Bioprinted Functional and Contractile Cardiac Tissue Constructs. Acta Biomater, 70:48–56.
61. Dai X, Ma C, Lan Q, et al., 2016, 3D Bioprinted Glioma Stem Cells for Brain Tumor Model and Applications of Drug Susceptibility. Biofabrication, 8:045005. DOI: 10.1088/1758-5090/8/4/045005.
62. Xu F, Celli J, Rizvi I, et al., 2011, A Three-Dimensional In Vitro Ovarian Cancer Coculture Model Using a High throughput Cell Patterning Platform. Biotechnol J, 6:204–12. DOI: 10.1002/biot.201000340.
63. Zhao Y, Yao R, Ouyang L, et al., 2014, Three-Dimensional Printing of Hela Cells for Cervical Tumor Model In Vitro. Biofabrication, 6:035001. DOI: 10.1088/1758-5082/6/3/035001.
64. Koch L, Deiwick A, Schlie S, et al., 2012, Skin Tissue Generation by Laser Cell Printing. Biotechnol Bioeng, 109:1855–63.
65. Tseng H, Gage JA, Shen T, et al., 2015, A Spheroid Toxicity Assay Using Magnetic 3D Bioprinting and Real Time Mobile Device-Based Imaging. Sci Rep, 5:13987. DOI: 10.1038/srep13987.
66. Tran JL, 2015, To Bioprint or Not to Bioprint. N C J Law Tech, 15:123–78.
67. Murphy SV, Atala A, 2014, 3D Bioprinting of Tissues and Organs. Nat Biotechnol, 32:773–85. DOI: 10.1038/nbt.2958.
68. Groll J, Boland T, Blunk T, et al., 2016, Biofabrication: Reappraising the Definition of Elemoso, et al. International Journal of Bioprinting (2020)–Volume 6, Issue 3 15 an Evolving Field. Biofabrication, 8:013001. Doi: 10.1088/1758-5090/8/1/013001.
69. Lamb J, 2018, FDA Code of Federal Regulations 21CFR1271. In: Scurvy: The Disease of Discovery. Princeton University Press, Princeton, New Jersey. pp. 15–20.
70. Di Prima M, Coburn J, Hwang D, et al., 2016, Additively Manufactured Medical Products the FDA Perspective. 3D Print Med, 2:1. DOI: 10.1186/s41205-016-0005-9.
71. Hourd P, Medcalf N, Segal J, et al., 2015, A 3D Bioprinting Exemplar of the Consequences of the Regulatory Requirements on Customized Processes. Regen Med, 10:863–83.
72. Available from: https://www.nasdaq.com/articles/depthlook- organovo. [Last accessed on 2012 Aug 20].
73. Available from: https://www.cellink.com/exclusive-withcellink- co-founders-erik-gatenholm-and-dr-hector-martinezin-medgadget. [Last accessed on 2020 Jul 27].
74. Available from: https://www.engineering.com/3DPrinting/3DPrintingArticles/ArticleID/9713/Japanese-BioPrinting-Firm-Closes-12M-Series-B-Funding.aspx. [Last accessed on 2020 Jul 27].
75. Available from: https://www.poietis.com/poietis-closes-a-5me-financing-round-to-accelerate-the-development-ofits-bio-printing-platform-for-tissue-therapy-manufacturing. [Last accessed on 2020 Jul 27].
76. Available from: https://www.betakit.com/aspect-biosystemsraises- 26-million-cad-in-series-a-for-3d-printing-of-humantissues. [Last accessed on 2020 Jul 27].
77. Available from: https://www.3dprintingindustry.com/news/allevi-launches-bioink-kit-for-3d-printing-multi-layeredskin-cells-151813. [Last accessed on 2020 Jul 27].
78. Available from: https://www.marketwatch.com/story/beyondmeat-soars-163-in-biggest-popping-us-ipo-since-2000. [Last accessed on 2019 Apr 02].
79. Available from: https://www.techcrunch.com/2019/09/05/novameat-has-a-platform-for-3d-printing-steaks-and-has-newmoney-to-take-it-to-market. [Last accessed on 2020 Jul 27].
80. Available from: https://www.forbes.com/sites/jimvinoski/2019/09/25/with-food-grade-3-d-printingredefine-meat-is-out-to-well-redefine-meat/#2971f42a75c7.[Last accessed on 2020 Jul 27].
81. Wilks M, Phillips CJ, 2017, Attitudes to In Vitro Meat: A Survey of Potential Consumers in the United States. PLoS One, 12:e0171904. DOI: 10.1371/journal.pone.0171904.
82. Available from: https://www.mosameat.com/blog/2019/11/15/mosa-meat-on-netflixs-explained. [Last accessed on 2020 Jul 27].
83. Available from: https://www.wired.com/story/the-high-costof-lab-to-table-meat. [Last accessed on 2020 Jul 27].
84. Available from: https://www.bloomberg.com/news/articles/2019-10-07/startup-aleph-farms-hitches-ride-on-issto-make-space-meat?srnd=premium-europe. [Last accessed on 2020 Jul 27].
85. Available from: https://www.bloomberg.com/news/articles/2019-10-07/startup-aleph-farms-hitches-ride-on-issto-make-space-meat. [Last accessed on 2020 Jul 27].
86. Ben-Arye T, Shulamit L, 2019, Tissue Engineering for Clean Meat Production. Front Sustain Food Syst,3:46. Doi: 10.3389/fsufs.2019.00046.
87. Available from: https://www.foodnavigator-usa.com/Article/2019/02/11/From-edible-scaffolding-to-bio-printing-What-would-an-industrial-scale-cell-based-meat-plant-looklike. [Last accessed on 2020 Jul 27].
88. Novik E, Yarmush ML, Freedman RB, et al., 2016, Compositions and Methods of Functionally Enhanced In Vitro Cell Culture System. Patent No. 20120129207A1 US.
89. JAKAB, 2014, Gabor Forgacs Francoise MargaKaroly Robert. Engineered Comestible Meat. Patent No. 8703216B2 US.
90. Yao L, Ou J, Wang G, et al., 2013, bioPrint: A Liquid Deposition Printing System. 3D Print Addit Manuf, 2:168–79. DOI: 10.1089/3dp.2015.0033.
91. Wang W, Yao L, Cheng CY, et al., 2017, Harnessing the Hygroscopic and Biofluorescent. Sci Adv, 3:e1601984.
92. Karoly J, Francoise M, Ryan K, et al., 2019, Non-medical Applications of Tissue Engineering: Biofabrication of a Leather-like Material. Mater Today Sustain, 5:100018. DOI: 10.1016/j.mtsust.2019.100018.