Software for Bioprinting
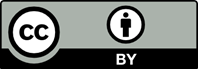
The bioprinting of heterogeneous organs is a crucial issue. To reach the complexity of such organs, there is a need for highly specialized software that will meet all requirements such as accuracy, complexity, and others. The primary objective of this review is to consider various software tools that are used in bioprinting and to reveal their capabilities. The sub-objective was to consider different approaches for the model creation using these software tools. Related articles on this topic were analyzed. Software tools are classified based on control tools, general computer-aided design (CAD) tools, tools to convert medical data to CAD formats, and a few highly specialized research-project tools. Different geometry representations are considered, and their advantages and disadvantages are considered applicable to heterogeneous volume modeling and bioprinting. The primary factor for the analysis is suitability of the software for heterogeneous volume modeling and bioprinting or multimaterial threedimensional printing due to the commonality of these technologies. A shortage of specialized suitable software tools is revealed. There is a need to develop a new application area such as computer science for bioprinting which can contribute significantly in future research work.
1. Fleming PA, Argraves WS, Gentile C, et al., 2010, Fusion of Uniluminal Vascular Spheroids: A Model for Assembly of Blood Vessels. Dev Dyn, 239:398–406. DOI: 10.1002/dvdy.22161.
2. Inamori M, Hiroshi M, Toshihisa K, 2009, An Approach for Formation of Vascularized Liver Tissue by Endothelial Cellcovered Hepatocyte Spheroid Integration. Tissue Eng Part A, 15:2029–2037. DOI: 10.1089/ten.tea.2008.0403.
3. Rouwkema J, Khademhosseini A, 2016, Vascularization and Angiogenesis in Tissue Engineering: Beyond Creating Static Networks. Trends Biotechnol, 34:733–45. DOI: 10.1016/j.tibtech.2016.03.002.
4. Hoch E, Tovar GE, Borchers K, 2014, Bioprinting of Artificial Blood Vessels: Current Approaches Towards a Demanding Goal. Eur J Cardiothorac Surg, 46:767–78. DOI: 10.1093/ejcts/ezu242.
5. Alonzo M, AnilKumar S, Roman B, et al., 2019, 3D Bioprinting of Cardiac Tissue and Cardiac Stem Cell Therapy. Transl Res, 211:64–83. DOI: 10.1016/j.trsl.2019.04.004.
6. Lee W, Debasitis JC, Lee VK, et al,. 2009, Multi-layered Culture of Human Skin Fibroblasts and Keratinocytes Through Three-dimensional Freeform Fabrication. Biomaterials, 30:1587–95. DOI: 10.1016/j.biomaterials.2008.12.009.
7. Vijayavenkataraman S, Lu WF, Fuh JY. 2016, 3D Bioprinting of Skin: A State-of-the-art Review on Modelling, Materials, and Processes. Biofabrication, 8:032001. DOI: 10.1088/1758-5090/8/3/032001.
8. Midha S, Patra P, Mohanty S, 2019, Advances in 3D Bioprinting of Bone: Progress and Challenges. J Tissue Eng Regen Med, 13:925–45. DOI: 10.1002/term.2847.
9. Adepu S, Dhiman N, Laha A, et al., 2017, Three-dimensional Bioprinting for Bone Tissue Regeneration. Curr Opin Biomed Eng, 42:22–8. DOI: 10.1016/j.cobme.2017.03.005.
10. Bulanova EA, Koudan EV, Degosserie J, et al., 2017, Bioprinting of a Functional Vascularized Mouse Thyroid Gland Construct. Biofabrication, 9:034105. DOI: 10.1088/1758-5090/aa7fdd.
11. Ma X, Liu J, Zhu W, et al., 2018, 3D Bioprinting of Functional Tissue Models for Personalized Drug Screening and In Vitro Disease Modeling. Adv Drug Deliv Rev, 132:235–51. DOI: 10.1016/j.addr.2018.06.011.
12. Peng W, Unutmaz D, Ozbolat IT, 2016, Bioprinting Towards Physiologically Relevant Tissue Models for Pharmaceutics. Trends Biotechnol, 34:722–32. DOI: 10.1016/j.tibtech.2016.05.013.
13. Peng W, Datta P, Ayan B, et al., 2017, 3D Bioprinting for Drug Discovery and Development in Pharmaceutics. Acta Biomater, 57:26–46. DOI: 10.1016/j.actbio.2017.05.025
14. Knowlton S, Onal S, Yu CH, et al., 2015, Bioprinting for Cancer Research. Trends Biotechnol, 33:504–13.
15. King SM, Presnell SC, Nguyen DG, et al., 2013, Development of 3D Bioprinted Human Breast Cancer for In Vitro Screening of Therapeutics Targeted Against Cancer Progression. American Society of Biology, New Orleans, LA. DOI: 10.1158/1538-7445.am2014-2034.
16. Knowlton S, Joshi A, Yenilmez B, et al., 2016, Advancing Cancer Research Using Bioprinting for Tumor-on-achip Platforms. Int J Bioprinting, 2:3–8. DOI: 10.18063/ijb.2016.02.003
17. Larsen M, Mishra R, Miller M., et al., 2015, Bioprinting of Bone. In: Essentials of 3D Biofabrication and Translation. Academic Press, Cambridge, Massachusetts. pp. 293–308. doi.org/10.1016/b978-0-12-800972-7.00017-7.
18. Duan B, 2017, State-of-the-art Review of 3D Bioprinting for Cardiovascular Tissue Engineering. Ann Biomed Eng, 45:195–209. DOI:10.1007/s10439-016-1607-5.
19. Gao Q, Liu Z, Lin Z, et al., 2017, 3D Bioprinting of Vessel-like Structures with Multilevel Fluidic Channels. ACS Biomater Sci Eng, 3:399–408. DOI: 10.1021/acsbiomaterials.6b00643.
20. Moldovan L, Babbey CM, Murphy MP, et al., 2017, Comparison of Biomaterial-dependent and-Independent Bioprinting Methods for Cardiovascular Medicine. Curr Opin Biomed Eng, 2:124–31. DOI: 10.1016/j.cobme.2017.05.009
21. Beyersdorf F, 2014, Three-dimensional Bioprinting: New Horizon for Cardiac Surgery. 46:339–41.
22. Pourchet LJ, Thepot A, Albouy M, et al., 2017, Human Skin 3D Bioprinting Using Scaffold-free Approach. Adv Healthc Mater, 6:1601101. DOI: 10.1002/adhm.201601101.
23. Murphy SV, Atala A, 2014, 3D Bioprinting of Tissues and Organs. Nat Biotechnol, 32:773–5. DOI: 10.1038/nbt.2958.
24. Müller M, Öztürk E, Arlov Ø, et al., 2017, Alginate Sulfatenanocellulose Bioinks for Cartilage Bioprinting Applications. Ann Biomed Eng, 45:210–23. DOI: 10.1007/s10439-016-1704-5.
25. Mouser VH, Melchels FP, Visser J, et al., 2016, Yield Stress Determines Bioprintability of Hydrogels Based on Gelatin-methacryloyl and Gellan Gum for Cartilage Bioprinting. Biofabrication, 8:035003. DOI: 10.1088/1758-5090/8/3/035003.
26. Di Bella C, Fosang A, Donati DM, et al., 2015, 3D Bioprinting of Cartilage for Orthopedic Surgeons: Reading between the Lines. Front Surg, 2:39. DOI: 10.3389/fsurg.2015.00039.
27. Nam KH, Smith AS, Lone S, et al., 2015, Biomimetic 3D Tissue Models for Advanced High-throughput Drug Screening. J Lab Autom, 20:201–15. DOI: 10.1177/2211068214557813.
28. Takahashi K, Tanabe K, Ohnuki M, et al., 2007, Induction of Pluripotent Stem Cells from Adult Human Fibroblasts by Defined Factors. Cell, 132:861–72. DOI: 10.1016/j.cell.2007.11.019.
29. Datta P, Ayan B, Ozbolat IT, 2017, Bioprinting for Vascular and Vascularized Tissue Biofabrication. Acta Biomater, 51:1–20. DOI: 10.1016/j.actbio.2017.01.035.
30. Sabater AL, Guarnieri A, Espana EM, et al., 2013, Strategies of Human Corneal Endothelial Tissue Regeneration. Regen Med, 8:183–95.
31. Tarassoli SP, Jessop ZM, Al-Sabah A, et al., 2018, Skin Tissue Engineering Using 3D Bioprinting: An Evolving Research Field. J Plastic Reconstr Aesthet Surg, 71:615–23. DOI: 10.1016/j.bjps.2017.12.006.
32. Katakam P, Dey B, Assaleh FH, et al., 2015, Top-down and Bottom-up Approaches in 3D Printing Technologies for Drug Delivery Challenges. Crit Rev Ther Drug Carrier Syst, 32:61–87. DOI: 10.1615/critrevtherdrugcarriersyst.2014011157.
33. Binder K, 2011, In Situ Bioprinting of the Skin. Wake Forest University, North Carolina.
34. Shi Y, Wang W, 2019, 3D Inkjet Printing of the Zirconia Ceramic Implanted Teeth. Mater Lett, 261:127131. DOI: 10.1016/j.matlet.2019.127131.
35. Yan Q, Dong H, Su J, et al., 2018, A Review of 3D Printing Technology for Medical Applications. Engineering, 4:729–42.
36. Mogali SR, Yeong WY, Tan H, et al., 2018, Evaluation by Medical Students of the Educational Value of Multi-material and Multi-colored Three-dimensional Printed Models of the Upper Limb for Anatomical Education. Anat Sci Educ, 11:54–64. DOI: 10.1002/ase.1703.
37. Hutmacher DW, 2000, Scaffolds in Tissue Engineering Bone and Cartilage. Biomaterials, 21:2529–43. DOI: 10.1016/s0142-9612(00)00121-6.
38. Wu C, Luo Y, Cuniberti G, et al., 2011, Three-dimensional Printing of Hierarchical and Tough Mesoporous Bioactive Glass Scaffolds with a Controllable Pore Architecture, Excellent Mechanical Strength and Mineralization Ability. Acta Biomater, 7:2644–50. DOI: 10.1016/j.actbio.2011.03.009.
39. Gratson GM, Xu M, Lewis JA, 2004, Microperiodic Structures: Direct Writing of Three-dimensional Webs. Nature, 428:386. DOI: 10.1038/428386a.
40. Bian WG, Lei P, Liang FH, et al., 2007, Morphogenetic Protein-2 and Gel Complex on Hydroxyapatite-coated Porous Titanium to Repair Defects of Distalfemur in Rabbits. Chin J Orthop Trauma, 9:550–4.
41. Pati F, Jang J, Ha DH, et al., 2014, Printing Three-dimensional Tissue Analogues with Decellularized Extracellular Matrix Bioink. Nat Commun, 5:3935. DOI: 10.1038/ncomms4935.
42. Gulyas M, Csiszer M, Mehes E, et al., 2018, Software Tools for Cell Culture-related 3D Printed Structures. PLoS One, 13:e0203203. DOI: 10.1371/journal.pone.0203203.
43. Chua CK, Wai YY, 2014, Bioprinting: Principles and Applications. Vol. 1. World Scientific Publishing Co., Inc., New Jersey.
44. Available from: https://www.regenhu.com/3d-bioprinters/software. [Last accessed on 2020 Feb 17].
45. Available from: https://www.cellink.com/software. [Last accessed on 2020 Feb 17].
46. Available from: https://www.3dnatives.com/en/allevi-softwarebioprinting-050920195. [Last accessed on 2020 Feb 17].
47. Naing MW, Chua CK, Leong KF, et al., 2005, Fabrication of Customised Scaffolds Using Computer-aided Design and Rapid Prototyping Techniques. Rapid Prototyp J, 11:249–59. DOI: 10.1108/13552540510612938.
48. Sudarmadji N, Chua CK, Leong KF, 2012, The Development of Computer-Aided System for Tissue Scaffolds (CASTS) System for Functionally Graded Tissue-Engineering Scaffolds. In: Computer-Aided Tissue Engineering. Springer, Berlin. pp. 111–123. DOI: 10.1007/978-1-61779-764-4_7.
49. Sil BC, Patel A, Crowther JM, et al., 2019, A Preliminary Investigation of Additive Manufacture to Fabricate Human Nail Plate Surrogates for Pharmaceutical Testing. Pharmaceutics, 11:250. DOI: 10.3390/pharmaceutics11060250.
50. Van der Valk DC, van der Ven CF, Blaser MC, et al., 2018, Engineering a 3D-Bioprinted Model of Human Heart Valve Disease Using Nanoindentation-based Biomechanics. Nanomaterials, 8:296. DOI: 10.3390/nano8050296.
51. Jeon O, Lee YB, Jeong H, et al., 2019, Living Cell-only Bioink and Photocurable Supporting Medium for Printing and Generation of Engineered Tissues with Complex Geometries. bioRxiv, 1:611525. DOI: 10.1101/611525.
52. Lehner BA, Schmieden DT, Meyer AS., 2017, A Straightforward Approach for 3D Bacterial Printing. ACS Synth Biol, 6:1124–30. DOI: 10.1021/acssynbio.6b00395.
53. Jeon O, Leea YB, Hinton TJ, et al., 2019, Cryopreserved Cell laden Alginate Microgel Bioink for 3D Bioprinting of Living Tissues. Mater Today Chem, 12:61–70. DOI: 10.1016/j.mtchem.2018.11.009.
54. Markstedt K, Håkanssonabd K, Toriz G, et al., 2019, Materials from Trees Assembled by 3D Printing Wood Tissue Beyond Nature Limits. Appl Mater Today, 15:280–5. DOI: 10.1016/j.apmt.2019.02.005.
55. Faramarzi N, Yazdi IK, Nabavinia M, et al., 2018, Patient specific Bioinks for 3D Bioprinting of Tissue Engineering Scaffolds. Adv Healthc Mater, 7:1701347. DOI: 10.1002/adhm.201701347.
56. Boyer CJ, Ballard DH, Yun JW, et al., 2018, Three dimensional Printing of Cell Exclusion Spacers (CES) for Use in Motility Assays. Pharm Res, 35:155. DOI: 10.1007/s11095-018-2431-4.
57. Boyer CJ, Ballard DH, Barzegar M, et al., 2018, High throughput Scaffold-free Microtissues through 3D Printing. 3D Print Med, 4:1–6. DOI: 10.1186/s41205-018-0029-4.
58. Ivanov DP, Grabowska AM, 2018, In Vitro Tissue Microarrays for Quick and Efficient Spheroid Characterization. SLAS Discov, 23:211–7.
59. Yang F, Tadepalli V, Wiley BJ, 2017, 3D Printing of a Double Network Hydrogel with a Compression Strength and Elastic Modulus Greater than those of Cartilage. ACS Biomater Sci Eng, 3:863–9. DOI: 10.1021/acsbiomaterials.7b00094.
60. Ferraz MA, Henning HH, Costa PF, et al., 2017, Improved Bovine Embryo Production in an Oviduct-on-a-Chip System: Prevention of Poly-spermic Fertilization and Parthenogenic Activation. Lab Chip, 17:905–16. DOI: 10.1039/c6lc01566b.
61. Knowlton S, Yu CH, Ersoy F, et al., 2016, 3D-printed Microfluidic Chips with Patterned, Cell-laden Hydrogel Constructs. Biofabrication, 8:025019. DOI: 10.1088/1758-5090/8/2/025019.
62. Adamkiewicz M, Rubinsky B., 2015, Cryogenic 3D Printing for Tissue Engineering. Cryobiology, 71:518–21. DOI:10.1016/j.cryobiol.2015.10.152.
63. Cristovão AF, Sousa D, Silvestre F, et al., 2019, Customized Tracheal Design Using 3D Printing of a Polymer Hydrogel:Influence of UV Laser Cross-linking on Mechanical Properties. 3D Print Med, 5:12. DOI: 10.1186/s41205-019-0049-8.
64. Mussi E, Furferi R, Volpe Y, et al., 2019, Ear Reconstruction Simulation: From Handcrafting to 3D Printing. Bioengineering, 6:14. DOI: 10.3390/bioengineering6010014.
65. Germain L, Fuentesc CA, van Vuure AW, et al., 2018, 3D-printed Biodegradable Gyroid Scaffolds for Tissue Engineering Applications. Mater Des, 151:113–22. DOI: 10.1016/j.matdes.2018.04.037.
66. Gu Q, Tomaskovic-Crook E, Lozano R, et al., 2016, Functional 3D Neural Mini-tissues from Printed Gel-based Bioink and Human Neural Stem Cells. Adv Healthc Mater, 5:1429–38. DOI: 10.1002/adhm.201670060.
67. Grigoryan B, Paulsen SJ, Corbett DC, et al., 2019, Multivascular Networks and Functional Intravascular Topologies within Biocompatible Hydrogels. Science, 364:458–64.
68. Costa PF, Albers HJ, Linssen JE, et al., 2017, Mimicking Arterial Thrombosis in a 3D-printed Microfluidic In Vitro Vascular Model Based on Computed Tomography Angiography Data. Lab Chip, 17:2785–92. DOI: 10.1039/c7lc00202e.
69. Liberski AR, 2016, Three-dimensional Printing of Alginate: From Seaweeds to Heart Valve Scaffolds. QSci Connect, 2:3. DOI: 10.5339/connect.2016.3.
70. Miller JS, Stevens KR, Yang MT, et al., 2012, Rapid Casting of Patterned Vascular Networks for Perfusable Engineered Three-dimensional Tissues. Nat Mater, 11:768–74. DOI: 10.1038/nmat3357.
71. Tomov ML, Cetnar A, Do K, 2019, Patient-Specific 3-Dimensional-Bioprinted Model for In Vitro Analysis and Treatment Planning of Pulmonary Artery Atresia in Tetralogy of Fallot and Major Aortopulmonary Collateral Arteries. J Am Heart Assoc, 8: e014490. DOI: 10.1161/jaha.119.014490.
72. McCracken JM, Rauzan BM, Kjellman JC, et al., 2019, Ionic Hydrogels with Biomimetic 4D-Printed Mechanical Gradients: Models for Soft-Bodied Aquatic Organisms. Adv Funct Mater, 29:1806723. DOI: 10.1002/adfm.201806723.
73. Ammar J, 2019, Defective Computer-Aided Design Software Liability in 3d Bioprinted Human Organ Equivalents. Santa Clara High Tech Law J. 35:37–67. Available from: https://www.digitalcommons.law.scu.edu/chtlj/vol35/iss3/2. [Last accessed on 2020 Feb 16].
74. Available from: https://www.materialise.com/en/medical/mimics-innovation-suite. [Last accessed on 2020 Feb 16].
75. Available from: https://www.solidworks.com/partnerproduct/biocad. [Last accessed on 2020 Feb 16].
76. Available from: https://www.cti.gov.br/en/invesalius. [Last accessed on 2020 Feb 16].
77. Amorim P, de Moraes TF, Pedrini H, et al., 2015, In Vesalius: An Interactive Rendering Framework for Health Care Support. International Symposium on Visual Computing. Springer, Cham.
78. Available from: https://www.slicer.org. [Last accessed on 2020 Feb 16].
79. Dernowsek A, Janaina D, Alvarenga RR, et al., 2017, The Role of Information Technology in the Future of 3D Biofabrication. J 3D Print Med, 1:63–74.
80. Rodrigo AR, Vladimir K, Vladimir M, et al., 2015, Organ Printing as an Information Technology. Proc Eng, 110:151–8.
81. Rezende RA, Mironov V, da Silva JV, 2016, Bioprinting Tissues and Organs. In: Reference Module in Materials Science and Materials Engineering. 1st ed., Vol. 1. Elsevier, Amsterdam, Netherlands, pp. 1-14. DOI: 10.1016/b978-0-12-803581-8.04139-4.
82. Available from: https://www.simplify3d.com. [Last accessed on 2020 Feb 15].
83. Sahai N, Gogoi M, 2020, 3D Tissue Scaffold Library Development form Medical Images for Bioprinting Application. Mater Today Proc. DOI: 10.1016/j.matpr.2019.12.063.
84. Jardini AL, Larosa MA, Filho RM, et al., 2014, Cranial Reconstruction: 3D Biomodel and Custom-built Implant Created Using Additive Manufacturing. J Craniomaxillofac Surg, 42:1877–84. DOI: 10.1016/j.jcms.2014.07.006.
85. Naghieh S, Sarker MD, Abelseth E, et al., 2019, Indirect 3D Bioprinting and Characterization of Alginate Scaffolds for Potential Nerve Tissue Engineering Applications. J Mech Behav Biomed Mater, 93:183–93. DOI: 10.31224/osf.io/pyq29.
86. Available from: https://www.ultimaker.com/software/ultimaker-cura. [Last accessed on 2020 Feb 15].
87. Available from: https://www.github.com/ultimaker/curaengine. [Last accessed on 2020 Feb 15].
88. Ariffin MK, Sukindar NA, Baharudin HT, et al., 2018, Slicer Method Comparison Using Open-source 3D Printer. IOP Conference Series: Earth and Environmental Science. Vol. 114. IOP Publishing, Bristol, United Kingdom. DOI: 10.1088/1755-1315/114/1/012018.
89. Mielczarek J, Gazdowicz G, Kramarz J, et al., 2015, A Prototype of a 3D Bioprinter. Solid State Phenomena. Vol. 237. Trans Tech Publications Ltd. DOI: 10.4028/www.scientific.net/ssp.237.221.
90. Datta S, Sarkar R, Vyas V, et al., 2018, Alginate-honey Bioinks with Improved Cell Responses for Applications as Bioprinted Tissue Engineered Constructs. J Mater Res, 33:2029–39. DOI: 10.1557/jmr.2018.202.
91. Jessop ZM, Al-Sabah A, Gardiner MD, et al., 2017, 3D Bioprinting for Reconstructive Surgery: Principles, Applications and Challenges. J Plas Reconstr Aesthetic Surg, 70:1155–70. DOI: 10.1016/j.bjps.2017.06.001.
92. Dávila JL, Freitas MS, Neto P, 2015, Software to Generate 3D Continuous Printing Paths for the Fabrication of Tissue Engineering Scaffolds. Int J Adv Manuf Technol, 84:1671–7. DOI: 10.1007/s00170-015-7866-8.
93. Malone E, Lipson H, 2007, Fab@ Home: The Personal Desktop Fabricator Kit. Rapid Prototyp J, 13:245–55. DOI: 10.1108/13552540710776197.
94. Fryazinov O, Vilbrandt T, Pasko A, 2013, Multi-scale Spacevariant FRep Cellular Structures. Comput Aided Des, 45:26–34. DOI: 10.1016/j.cad.2011.09.007.
95. Available from: http://www.hyperfun.org. [Last accessed on 2020 Feb 03].
96. Available from: http://www.uformia.com. [Last accessed on 2020 Feb 03].
97. Popov D, Sajfert V, Pop N, et al., 2020, Efficient Contouring of Functionally Represented Objects for Additive Manufacturing. Comput Aided Des. Available from: http: //github.com/Torrero/FRepCAM. [Last accessed on 2020 Jun 02].
98. Zhang XY, Yan XC, Fang G, et al., 2020, Biomechanical Influence of Structural Variation Strategies on Functionally Graded Scaffolds Constructed with Triply Periodic Minimal Surface. Addit Manuf, 32:101015. DOI: 10.1016/j.addma.2019.101015.
99. Tikhonov AA, Evdokimov PV, Putlyaev VI, et al., 2019, On the Choice of the Architecture of Osteoconductive Bioceramic Implants. Inorg Mater, 10:242–7.
100. Kapfer SC, Hyde ST, Mecke K, et al., 2011, Minimal Surface Scaffold Designs for Tissue Engineering. Biomaterials, 32:6875–82. DOI: 10.1016/j.biomaterials.2011.06.012.
101. Schwarz HA, 1972, Gesammelte Mathematische Abhandlungen. Vol. 260. American Mathematical Society, Providence, Rhode Island.
102. Schoen AH, 1970, Infinite Periodic Minimal Surfaces without Self-intersections. National Aeronautics and Space Administration, Washington, DC.
103. Karcher H, 1989, The Triply Periodic Minimal Surfaces of Alan Schoen and their Constant Mean Curvature Companions. Manuscr Math, 64:291–357. DOI: 10.1007/bf01165824.
104. Dinis JC, Moraes TF, Amorim HJ, et al., 2016, POMES: An Open-source Software Tool to Generate Porous/Roughness on Surface. Proced CIRP, 49:178–82. DOI: 10.1016/j.procir.2015.07.085.
105. Available from: https://www.ntopology.com. [Last accessed on 2020 Feb 05].
106. Li H, Li K, Kim T, et al., 2012, Spatial Modeling of Bone Microarchitecture. Proc SPIE, 8290:23.
107. Available from: https://www.grasshopper3d.com. [Last accessed on 2020 Feb 05].
108. Available from: https://www.autodesk.com. [Last accessed on 2020 Feb 05].
109. Robu A, Robu N, Neagu A, 2018, New Software Tools for Hydrogel-based Bioprinting. 2018 IEEE 12th International Symposium on Applied Computational Intelligence and Informatics (SACI). IEEE, Piscataway, New Jersey. DOI: 10.1109/saci.2018.8440971.
110. Robu A, Stoicu-Tivadar L, 2016, SIMMMC an Informatics Application for Modeling and Simulating the Evolution of Multicellular Systems in the Vicinity of Biomaterials. Rom J Biophys, 26:145–62.
111. Neagu A, 2017, Role of Computer Simulation to Predict the Outcome of 3D Bioprinting. J 3D Print Med, 1:103–21.
112. Glazier JA, Balter A, Popławski NJ, Anderson AR, Chaplain MA, Rejniak KA, 2007, Magnetization to Morphogenesis: A Brief History of the Glazier Graner Hogeweg Model. In: Anderson AR, Chaplain MA, Rejniak KA, editors. Single-Cell-Based Models in Biology and Medicine, Birkhäuser, Basel, Switzerland, pp. 79–106. DOI: 10.1007/978-3-7643-8123-3_4.
113. Izaguirre JA, Chaturvedi R, Huang C, et al., 2004, CompuCell, a Multi-model Framework for Simulation of Morphogenesis. Bioinformatics, 20:1129–37.
114. Swat MH, Hester SD, Balter AI, et al., 2009, Multicell Simulations of Development and Disease Using the CompuCell3D Simulation Environment. In: Maly IV, editor. Systems Biology. Humana Press, New York, USA, pp. 361–428. DOI: 10.1007/978-1-59745-525-1_13.
115. Hoehme S, Drasdo D, 2010, A Cell-based Simulation Software for Multi-cellular Systems. Bioinformatics, 26:2641–2. DOI: 10.1093/bioinformatics/btq437.
116. Brakke KA, 1992, The Surface Evolver. Exp Math, 1:141–65.
117. Rezende R. A., V. Mironov, and J. V. L. da Silva. 2016, Bioprinting Tissues and Organs. In: Reference Module in Materials Science and Materials Engineering. Elsevier Amsterdam, Netherlands, pp. 1–14. DOI: 10.1016/b978-0-12-803581-8.04139-4.
118. Rezende RA, Vladimir K, Vladimir M, Lopes Da JV, 2015, Organ Printing as an Information Technology. Proc Eng, 110:151–8.
119. Rezende R, Laureti CA, da Silva JV, et al., 2011, Towards Simulation of a Bioreactor Environment for Biofabricated Tissue Maturation. In: Bártolo PJ, editor. Innovative Developments in Virtual and Physical Prototyping. CRC Press, Boca Raton, Florida. DOI: 10.1201/b11341-110.
120. Burdge DA, Libourel IG, 2014, Open-source Software to Control Bioflo Bioreactors. PLoS One, 9:e92108. DOI: 10.1371/journal.pone.0092108.
121. Available from: http://www.ils-automation.com. [Last accessed on 2020 Feb 06].
122. Available from: https://www.lambda-instruments.com/fnetfermentor-control-software. [Last accessed on 2020 Feb 06].
123. Available from: http://www.fermentor.net/feature/fermentationcontrol-software. [Last accessed on 2020 Feb 06].
124. Available from: https://www.bioprocessonline.com/doc/biocommand-software-0002. [Last accessed on 2020 Feb 06].
125. Weiguo L, Schmidt B, 2006, Parallel Pattern-based Systems for Computational Biology: A Case Study. IEEE Trans Parallel Distrib Syst, 17:750–63. DOI: 10.1109/tpds.2006.109.
126. Carsten M, John M, Röhl M, et al., 2008, Hierarchical Modeling for Computational Biology. In: International School on Formal Methods for the Design of Computer, Communication and Software Systems. Springer, Berlin, Heidelberg.
127. Davide C, 2017, Ten Quick Tips for Machine Learning in Computational Biology. BioData Min, 10:35.
128. Turlif V, Pasko A, Vilbrandt C, 2009, Fabricating Nature. Technoetic Arts, 7:165–73. DOI: 10.1386/tear.7.2.165/1.
129. Alexander P, Fryazinov O, Vilbrandt T, et al., 2011, Procedural Function-based Modelling of Volumetric Microstructures. Graph Models, 73:165–81. DOI: 10.1016/j.gmod.2011.03.001
130. Alexander P, Adzhiev V, Sourin A, et al., 1995, Function Representation in Geometric Modeling: Concepts, Implementation and Applications. Vis Comput, 11:429–46. DOI: 10.1007/s003710050034
131. Alexander P, Adzhiev V, Schmitt B, et al., 2001, Constructive Hypervolume Modeling. Graph Models, 63:413–42. DOI: 10.1006/gmod.2001.0560.
132. Vladimir VS, Basnakian AG, Pasko AA, et al., 1995, Simulation of a Growing Mammalian Cell Colony: Collision-based Packing Algorithm for Deformable Particles. Comput Graph, 1:437–47. DOI: 10.1016/b978-0-12-227741-2.50035-9.
133. Available from: https://www.github.com/curv3d/curv. [Last accessed on 2020 Feb 19].
134. Available from: https://libfive.com. [Last accessed on 2020 Feb 19].
135. Available from: https://www.materialise.com. [Last accessed on 2020 Feb 19].
136. Available from: https://www.tinkercad.com. [Last accessed on 2020 Feb 19].
137. Available from: http://www.meshmixer.com. [Last accessed on 2020 Feb 19].
138. Available from: https://www.blender.org. [Last accessed on 2020 Feb 19].
139. Available from: https://www.github.com/jcdinis/POMES. [Last accessed on 2020 Feb 19].
140. Available from: https://www.ntopology.com/ntop-platform. [Last accessed on 2020 Feb 19].
141. Available from: https://www.autodesk.com/products/netfabb/overview. [Last accessed on 2020 Feb 19].