3D Printed Gene-activated Octacalcium Phosphate Implants for Large Bone Defects Engineering
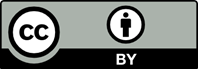
The aim of the study was the development of three-dimensional (3D) printed gene-activated implants based on octacalcium phosphate (OCP) and plasmid DNA encoding VEGFA. The first objective of the present work involved design and fabrication of gene-activated bone substitutes based on the OCP and plasmid DNA with VEGFА gene using 3D printing approach of ceramic constructs, providing the control of its architectonics compliance to the initial digital models. X-ray diffraction, scanning electron microscopy (SEM), Fourier transform infrared spectroscopy, and compressive strength analyses were applied to investigate the chemical composition, microstructure, and mechanical properties of the experimental samples. The biodegradation rate and the efficacy of plasmid DNA delivery in vivo were assessed during standard tests with subcutaneous implantation to rodents in the next stage. The final part of the study involved substitution of segmental tibia and mandibular defects in adult pigs with 3D printed gene-activated implants. Biodegradation, osteointegration, and effectiveness of a reparative osteogenesis were evaluated with computerized tomography, SEM, and a histological examination. The combination of gene therapy and 3D printed implants manifested the significant clinical potential for effective bone regeneration in large/critical size defect cases.
1. Number of All-listed Procedures for Discharges from Shortly stay Hospitals, 2010. Available from: http//www.cdc.gov/nchs/data/nhds/10Detaileddiagnosesprocedures/2010det10_alllistedprocedures.pdf. [Last accessed on 2020 March 01].
2. Rogers GF, Greene AK, 2017, Autogenous Bone Graft: Basic Science and Clinical Implications. J Craniofac Surg, 23:323–7.
3. Hernandez I, Kumar A, Joddar B, 2017, A Bioactive Hydrogel and 3D-Printed Polycaprolactone System for Bone Tissue Engineering. Gels, 3:26. DOI: 10.3390/gels3030026.
4. Ge Z, Tian X, Heng BC, et al., 2009, Histological Evaluation of Osteogenesis of 3D-Printed Poly-lactic-co-glycolic Acid (PLGA) Scaffolds in a Rabbit Model. Biomed Mater, 4:021001.DOI: 10.1088/1748-6041/4/2/021001.
5. Shim JH, Kim SE, Park JY, et al., 2014, Three-dimensional Printing of rhBMP-2-loaded Scaffolds with Long-term Delivery for Enhanced Bone Regeneration in a Rabbit Diaphyseal Defect. Tissue Eng Part A, 20:1980–92.DOI: 10.1089/ten.tea.2013.0513.
6. Ahlfeld T, Doberenz F, Kilian D, et al., 2018, Bioprinting of Mineralized Constructs Utilizing Multichannel Plotting of a Self-setting Calcium Phosphate Cement and a Cell-laden Bioink. Biofabrication, 10:045002. DOI: 10.1088/1758-5090/aad36d.
7. Dorozhkin SV, 2009, Calcium Orthophosphates in Nature Biology and Medicine. Materials, 2:399–498.DOI: 10.3390/ma2020399.
8. Liu Y, Cooper PR, Barralet JE, et al., 2007, Influence of Calcium Phosphate Crystal Assemblies on the Proliferation and Osteogenic Gene Expression of Rat Bone Marrow Stromal Cells. Biomaterials, 28:1393–1403.DOI: 10.1016/j.biomaterials.2006.11.019.
9. Deev RV, Drobyshev AY, Bozo IY, et al., 2015, Ordinary and Activated Bone Grafts: Applied Classification and the Main Features. Biomed Res Int, 2015:365050. DOI: 10.1155/2015/365050.
10. Murphy SV, Atala A, 2014, 3D Bioprinting of Tissues and Organs. Nat Biotechnol, 32:773–85.DOI: 10.1038/nbt.2958.
11. Komlev VS, Popov VK, Mironov AV, et al., 2015, 3D Printing of Octacalcium Phosphate Bone Substitutes. Front Bioeng Biotechnol, 3:81.
12. Barinov SM, Vakhrushev IV, Komlev VS, et al., 2015, 3D Printing of Ceramic Matrices for Engineering of Bone Tissue. Inorg Mater, 6:316–322.DOI: 10.1177/1074248415574336.
13. Deev RV, Bozo IY, Mzhavanadze ND, et al., 2015, pCMVvegf165 Intramuscular Gene Transfer is an Effective Method of Treatment for Patients with Chronic Lower Limb Ischemia. J Cardiovasc Pharmacol Ther, 20:473–82.
14. Bozo IY, Komlev VS, Drobyshev AY, et al., 2015, Method for Creating a Personalized Gene-activated Implant for Regenerating Bone Tissue. Patent No. EP3130342.
15. Fowler BO, Marković M, Brown WE, 1993, Octacalcium Phosphate. 3. Infrared and Raman Vibrational Spectra. Chem Mater, 5:1417–23.DOI: 10.1021/cm00034a009.
16. Kang HW, Lee SJ, Ko IK, et al., 2016, A 3D Bioprinting System to Produce Human-scale Tissue Constructs with Structural Integrity. Nat Biotechnol, 34:312–9.DOI: 10.1038/nbt.3413.
17. Daly AC, Pitacco P, Nulty J, et al., 2018, 3D-printed Microchannel Networks to Direct Vascularisation during Endochondral Bone Repair. Biomaterials, 162:34–46.DOI: 10.1016/j.biomaterials.2018.01.057.
18. Lal H, Patralekh MK, 2018, 3D-Printing and its Applications in Orthopaedic Trauma: A Technological Marvel. J Clin Orthop Trauma, 9:260–8.DOI: 10.1016/j.jcot.2018.07.022.
19. Huang KH, Lin YH, Shie MY, et al., Effects of Bone Morphogenic Protein-2 Loaded on the 3D-Printed MesoCSScaffolds. J Formos Med Assoc, 117:879-87.DOI: 10.1016/j.jfma.2018.07.010.
20. Lee SJ, Lee D, Yoon TR, et al., 2016, Surface Modification of 3D-Printed Porous Scaffolds via Mussel-inspired Polydopamineand Effective Immobilization of rhBMP-2 to Promote Osteogenic Differentiation for Bone Tissue Engineering. Acta Biomater, 40:182–191.DOI: 10.1016/j. actbio.2016.02.006.
21. Du M, Chen B, Meng Q, et al., 2015, 3D Bioprinting of BMSC-laden Methacrylamide Gelatin Scaffolds with CBDBMP2-collagen Microfibers. Biofabrication, 7:044104.DOI: 10.1088/1758-5090/7/4/044104.
22. Deev RV, Drobyshev RV, Bozo IY, et al., 2013, Construction and Biological Effect Evaluation of Gene-activated Osteoplastic Material with Human VEGF Gene. Cell Transplant Tissue Eng, 8:78–85.
23. Feichtinger GA, Hofmann AT, Slezak P, et al., 2014, Sonoporation Increases Therapeutic Efficacy of Inducible and Constitutive BMP2/7 In Vivo Gene Delivery. Hum Gene Ther Methods, 25:57–71.DOI: 10.1089/hgtb.2013.113.
24. Evans CH, Huard J, 2015, Gene Therapy Approaches to Regenerating the Musculoskeletal System. Nat Rev Rheumatol, 11:234–42.DOI: 10.1038/nrrheum.2015.28.
25. Cunniffe GM, Gonzalez-Fernandez T, Daly A, et al.,2017, Three-dimensional Bioprinting of Polycaprolactone Reinforced Gene Activated Bioinksfor Bone Tissue Engineering. Tissue Eng Part A, 23:891–900.DOI: 10.1089/ten.tea.2016.0498.
26. Gonzalez-Fernandez T, Rathan S, Hobbs C, 2019, Pore forming Bioinksto Enable Spatio-temporally Defined Gene Delivery in Bioprinted Tissues. J Control Release, 301:13–27.DOI: 10.1016/j.jconrel.2019.03.006.
27. Komlev VS, Barinov SM, Bozo IY, et al., 2014, Bioceramics Composed of Octacalcium Phosphate Demonstrate Enhanced Biological Behaviour. ACS Appl Mater Interfaces, 6:16610–20.DOI: 10.1021/am502583p.
28. Bozo IY, Deev RV, Drobyshev AY, et al., 2016, World’s First Clinical Case of Gene-activated Bone Substitute Application. Case Rep Dent, 2016:8648949.DOI: 10.1155/2016/8648949.
29. Yang YQ, Tan YY, Wong R, et al., 2012, The Role of Vascular Endothelial Growth Factor in Ossification. Int J Oral Sci, 4:64–8.
30. Liu Y, Berendsen AD, Jia S, et al., 2012, Intracellular VEGF Regulates the Balance Between Osteoblast and Adipocyte Differentiation. J Clin Invest, 122:3101–13.DOI: 10.1172/jci61209.
31. Bozo IY, Deev RV, Drobyshev AY, et al., 2015, Efficacy of Gene-activated Osteoplastic Material Based on Octacalcium Phosphate and Plasmid DNA with VEGF Gene for Criticalsized Bone Defects Substitution. N.N. Priorov Herald Traumatol Orthop, 1:35–42.DOI: 10.32414/0869-8678-2015-1-35-42.
32. Schertzer JD, Plant DR, Lynch GS, 2006, Optimizing Plasmid-based Gene Transfer for Investigating Skeletal Muscle Structure and Function. Mol Ther, 13:795–803.DOI: 10.1016/j.ymthe.2005.09.019.
33. Keeney M, van den Beucken JJ, van der Kraan PM, et al., 2010, The Ability of a Collagen/Calcium Phosphate Scaffold to Act as its Own Vector for Gene Delivery and to Promote Bone Formation Via Transfection with VEGF (165). Biomaterials, 31:2893–902.DOI: 10.1016/j.biomaterials.2009.12.041.