Electrically Conducting Hydrogels for Health care: Concept, Fabrication Methods, and Applications
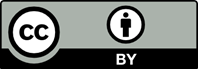
Electrically conducting hydrogels are gaining increasing attention due to their potential application in smart patches, biosensors, functional tissue engineering scaffolds, wound management, and implants. The current review focuses on these novel materials, their synthesis routes, and their composites. Special attention is paid to fabrication routes to produce functional composites with organic and inorganic components. The design of conductive hydrogels leads to inheritance of the advantages of each component and offers new features from the synergistic effects between the components, thus opening new application areas. The review also discusses the emerging role of 3D printing as an advanced approach toward new design, functionality, and material combination possibilities. The issue of lack of the spatial control with current techniques is highlighted, and possible new routes to solve it are discussed. The review will provide readers with knowledge tool to select appropriate methodology for designing desired hydrogel material composites.
1. Miyata T, Uragami T, Nakamae K, 2002, Biomolecule sensitive Hydrogels. Adv Drug Deliv Rev, 54:79–98. DOI:10.1016/s0169-409x(01)00241-1.
2. Hoffman AS, 2012, Hydrogels for Biomedical Applications. Adv Drug Deliv Rev, 64:18–23.
3. Hardy JG, Villancio-Wolter MK, Sukhavasi RC, 2015, Electrical Stimulation of Human Mesenchymal Stem Cells on Conductive Nanofibers Enhances their Differentiation Toward Osteogenic Outcomes. Macromol Rapid Commun, 36:1884–90. DOI: 10.1002/marc.201500233.
4. Guiseppi-Elie A, 2010, Electroconductive Hydrogels: Synthesis, Characterization and Biomedical Applications. Biomaterials, 31:2701–16. DOI: 10.1016/j.biomaterials.2009.12.052.
5. Distler T, Boccaccini AR, 2019, 3D Printing of Electrically Conductive Hydrogels for Tissue Engineering and Biosensors-a Review. Acta Biomater, 101:1–13. DOI: 10.1016/j.actbio.2019.08.044.
6. Nair LS, Laurencin CT, 2007, Silver Nanoparticles: Synthesis and Therapeutic Applications. J Biomed Nanotechnol, 3:301–16.
7. Endo T, Ikeda R, Yanagida Y, et al., 2008, Stimuli-responsive Hydrogel-silver Nanoparticles Composite for Development of Localized Surface Plasmon Resonance-based Optical Biosensor. Anal Chim Acta, 611:205–11. DOI: 10.1016/j.aca.2008.01.078.
8. Vimala K, Sivudu KS, Mohan YM, et al., 2009, Controlled Silver Nanoparticles Synthesis in Semi-hydrogel Networks of Poly (Acrylamide) and Carbohydrates: A Rational Methodology for Antibacterial Application. Carbohydr Polym, 75:463–71. DOI: 10.1016/j.carbpol.2008.08.009.
9. Bardajee GR, Hooshyar Z, Rezanezhad H, 2012, A Novel and Green Biomaterial Based Silver Nanocomposite Hydrogel:Synthesis, Characterization and Antibacterial Effect. J Inorg Biochem, 117:367–73. DOI: 10.1016/j.jinorgbio.2012.06.012.
10. Mohan YM, Lee K, Premkumar T, et al., 2007, Hydrogel Networks as Nanoreactors: A Novel Approach to Silver Nanoparticles for Antibacterial Applications. Polymer, 48:158–64. DOI: 10.1016/j.polymer.2006.10.045.
11. Wei QB, Fu F, Zhang YQ, et al., 2014, Preparation, Characterization, And Antibacterial Properties Of Ph-Responsive P(MMA-Co-MAA)/Silver Nanocomposite Hydrogels. J Polym Res, 21:349. DOI: 10.1007/s10965-013-0349-4.
12. Juby KA, Dwivedi C, Kumar M, et al., 2012, Silver Nanoparticle-loaded PVA/gum Acacia Hydrogel: Synthesis, Characterization and Antibacterial Study. Carbohydr Polym, 89:906–13. DOI: 10.1016/j.carbpol.2012.04.033.
13. Devaki SJ, Narayanan RK, Sarojam S, 2014, Electrically Conducting Silver Nanoparticle-polyacrylic Acid Hydrogel by In situ Reduction and Polymerization Approach. Mater Lett, 116:135–8. DOI: 10.1016/j.matlet.2013.10.110.
14. Xiao H, Xia Y, Cai C, 2013, Conducting Polymer Hydrogel Reactor for Synthesizing Au Nanoparticles and Its Use in the Reduction of P-nitrophenol. J Nanopart Res, 15:1521. DOI: 10.1007/s11051-013-1521-9.
15. Zhai D, Liu B, Shi Y, 2013, Highly Sensitive Glucose Sensor Based on Pt Nanoparticle/Polyaniline Hydrogel Heterostructures. ACS Nano, 7:3540–6. DOI: 10.1021/nn400482d.
16. Fuhrer R, Athanassiou EK, Luechinger NA, et al., 2009, Crosslinking Metal Nanoparticles into the Polymer Backbone of Hydrogels Enables Preparation of Soft, Magnetic Field driven Actuators with Muscle-like Flexibility. Small, 5(3):383–8. DOI: 10.1002/smll.200801091.
17. Mittal H, Ballav N, Mishra SB, 2014, Gum Ghatti and Fe3O4 Magnetic Nanoparticles Based Nanocomposites for the Effective Adsorption of Methylene Blue from Aqueous Solution. J Ind Eng Chem, 20:2184–92. DOI: 10.1016/j.jiec.2013.09.049.
18. Yuan B, Cademartiri L, 2015, Flexible One-dimensional Nanostructures: A Review. J Mater Sci Technol, 31: 607–15.
19. Gong S, Cheng W, 2017, One‐dimensional Nanomaterials for Soft Electronics. Adv Electron Mater, 3:1600314. DOI:10.1002/aelm.201600314.
20. Lim C, Shin Y, Jung J, 2019, Stretchable Conductive Nanocomposite Based on Alginate Hydrogel and Silver Nanowires for Wearable Electronics. APL Mater, 7:031502. DOI: 10.1063/1.5063657.
21. Cho Y, Borgens RB, 2010, The Effect of An Electrically Conductive Carbon Nanotube/Collagen Composite on Neurite Outgrowth of PC12 Cells. J Biomed Mater Res A, 95:510–7. DOI: 10.1002/jbm.a.32841.
22. Shin SR, Jung SM, Zalabany M, et al., 2013, Carbonnanotube-embedded Hydrogel Sheets for Engineering Cardiac Constructs and Bioactuators. ACS Nano, 7:2369–80. DOI: 10.1021/nn305559j.
23. Pok S, Vitale F, Eichmann SL, et al., 2014, Biocompatible Carbon Nanotube Chitosan Scaffold Matching the Electrical Conductivity of the Heart. ACS Nano, 8:9822–32. DOI: 10.1021/nn503693h.
24. Liu XW, Huang YX, Sun XF, et al., 2014, Conductive Carbon Nanotube Hydrogel as a Bioanode for Enhanced Microbial Electrocatalysis. ACS Appl Mater Interfaces, 6:8158−64. DOI: 10.1021/am500624k.
25. Chen Z, To JW, Wang C, et al., 2014, A Three-dimensionally Interconnected Carbon Nanotube-conducting Polymer Hydrogel Network for High-performance Flexible Battery Electrodes. Adv Energy Mater, 4:1400207. DOI: 10.1002/aenm.201400207.
26. Kumar GG, Hashmi S, Karthikeyan C, et al., 2014, Graphene Oxide/Carbon Nanotube Composite Hydrogels-Versatile Materials for Microbial Fuel Cell Applications. Macromol Rapid Commun, 35:1861–5. DOI: 10.1002/marc.201400332.
27. Chen PY, Courchesne NM, Hyder MN, et al., 2015, Carbon Nanotube-polyaniline Core-shell Nanostructured Hydrogel for Electrochemical Energy Storage. RSC Adv, 5:37970–9977. DOI: 10.1039/c5ra02944a.
28. Sayyar S, Murray E, Thompson BC, et al., 2015, Processable Conducting Graphene/Chitosan Hydrogels for Tissue Engineering. J Mater Chem B, 3:481-90.
29. Jo H, Sim M, Kim S, et al., 2017, Electrically Conductive Graphene/Polyacrylamide Hydrogels Produced by Mild Chemical Reduction for Enhanced Myoblast Growth and Differentiation. Acta Biomater, 48:100–9. DOI: 10.1016/j. actbio.2016.10.035.
30. Alam A, Meng Q, Shi G, et al., 2016, Electrically Conductive, Mechanically Robust, pH-sensitive Graphene/Polymer Composite Hydrogels. Compos Sci Technol, 127:119–26. DOI: 10.1016/j.compscitech.2016.02.024.
31. Baniasadi H, Ramazani AS, Mashayekhan S, 2015, Fabrication and Characterization of Conductive Chitosan/Gelatin-Based Scaffolds for Nerve Tissue Engineering. Int J Biol Macromol, 74:360–6. DOI: 10.1016/j.ijbiomac.2014.12.014.
32. Wang J, Li BY, Ni T, et al., 2015c, One-step Synthesis of Iodine Doped Polyaniline-Reduced Graphene Oxide Composite Hydrogel with High Capacitative Properties. Compos Sci Technol, 109:12–7.
33. Zhang L, Shi G, 2011, Preparation of Highly Conductive Graphene Hydrogels for Fabricating Supercapacitors with High Rate Capability. J Phys Chem C, 115:17206–12. DOI: 10.1021/jp204036a.
34. Xu Y, Lin Z, Huang X, et al., 2013, Flexible Solid-state Supercapacitors Based on Three-dimensional Graphene Hydrogel Films. ACS Nano, 7:4042–9. DOI: 10.1021/nn4000836.
35. Moughton AO, Hillmyer MA, Lodge TP, 2012, Multicompartment Block Polymer Micelles. Macromolecules, 45:2-19. DOI: 10.1021/ma201865s.
36. Li GL, Mohwald H, Shchukin DG, 2013, Precipitation Polymerization for Fabrication of Complex Core-shell Hybrid Particles and Hollow Structures. Chem Soc Rev, 42:3628–46. DOI: 10.1039/c3cs35517a.
37. Schlüter AD, Halperin A, Kröger M, et al., 2014, Dendronized Polymers: Molecular Objects Between Conventional Linear Polymers and Colloidal Particles. ACS Macro Lett, 3:991–8. DOI: 10.1021/mz500376e.
38. McLeish TC, 2007, Macromolecular Engineering: Precise Synthesis, Materials Properties, Applications. Weinheim, Germany: Wiley-VCH. p1605.
39. Shi XW, Hu YL, Tu K, et al., 2013, Electromechanical Polyaniline-cellulose Hydrogels with High Compressive Strength. Soft Matter, 9:10129–34. DOI: 10.1039/c3sm51490k.
40. Liang XT, Qu B, Li JR, et al., 2015, Preparation of Cellulose based Conductive Hydrogels with Ionic Liquid. React Funct Polym, 86:1–6.
41. Gilmore K, Hodgson AJ, Luan B, et al., 1994, Preparation of Hydrogel/conducting Polymer Composites. Polym Gels Netw, 2:135–43. DOI: 10.1016/0966-7822(94)90032-9.
42. Yang B, Yao F, Hao T, et al., 2016, Development of Electrically Conductive Double-Network Hydrogels via One-Step Facile Strategy for Cardiac Tissue Engineering. Adv Healthc Mater, 5:474–88. DOI: 10.1002/adhm.201500520.
43. Xiao YH, He L, Che JF, 2012, An Effective Approach for the Fabrication of Reinforced Composite Hydrogel Engineered with SWNTs, Polypyrrole and PEGDA Hydrogel. J Mater Chem, 22:8076–82. DOI: 10.1039/c2jm30601h.
44. Kim YS, Cho K, Lee HJ, et al., 2016, Highly Conductive and Hydrated PEG-Based Hydrogels for the Potential Application of a Tissue Engineering Scaffold. React Funct Polym, 109:15–22. DOI: 10.1016/j.reactfunctpolym.2016.09.003.
45. Sasaki M, Karikkineth BC, Nagamine K, et al., 2014, Highly Conductive Stretchable and Biocompatible Electrode-Hydrogel Hybrids for Advanced Tissue Engineering. Adv Healthc Mater, 3:1919–27. DOI:10.1002/adhm.201400209.
46. Dai TY, Qing XT, Zhou H, et al., 2010, Mechanically Strong Conducting Hydrogels with Special Double network Structure. Synth Met, 160:791–6. DOI: 10.1016/j.synthmet.2010.01.024.
47. Elyashevich GK, Smirnov MA, 2012, New pH-responsive and Electroactive Composite Systems Containing Hydrogels and Conductive Polymers on a Porous Matrix. Polym Sci A, 54:900–8. DOI: 10.1134/s0965545x12110028.
48. Dai TY, Qing XT, Xia YY, 2009, Conducting Hydrogels with Enhanced Mechanical Strength. Polymer, 50:5236–41. DOI: 10.1016/j.polymer.2009.09.025.
49. Wang YQ, Shi Y, Pan LJ, et al., 2015, Dopant-enabled Supramolecular Approach for Controlled Synthesis of Nanostructured Conductive Polymer Hydrogels. Nano Lett, 15:7736–41. DOI: 10.1021/acs.nanolett.5b03891.
50. Sershen SR, Westcott SL, Halas NH, et al., 2002, Independent Optically Addressable Nanoparticle-polymer Optomechanical Composites. Appl Phys Lett, 80:4609. DOI: 10.1063/1.1481536.
51. Pardo-Yissar V, Gabai R, Shipway AN, et al., 2001, Gold Nanoparticle/Hydrogel Composites with Solvent‐Switchable Electronic Properties. Adv Mater, 13:1320–3. DOI: 10.1002/1521-4095(200109)13:17<1320:aidadma1320>3.0.co;2-8.
52. Wang C, Flynn NT, Langer R, 2004, Controlled Structure and Properties of Thermoresponsive Nanoparticle-hydrogel Composites. Adv Mater, 16:1074–9. DOI: 10.1002/adma.200306516.
53. Souza GR, Christianson DR, Staquicini FI, et al., 2006, Networks of Gold Nanoparticles and Bacteriophage as Biological Sensors and Cell-targeting Agents. Proc Natl Acad Sci USA, 103:1215–20. DOI: 10.1073/pnas.0509739103.
54. Wu H, Yu G, Pan L, et al., 2013, Stable Li-ion Battery Anodes by in situ Polymerization of Conducting Hydrogel to Conformally Coat Silicon Nanoparticles. Nat Commun, 4:1943. DOI: 10.1038/ncomms2941.
55. Saravanan P, Raju MP, Alam S, 2007, A Study on Synthesis and Properties of Ag Nanoparticles Immobilized Polyacrylamide Hydrogel Composites. Mater Chem Phys, 103:278–82. DOI: 10.1016/j.matchemphys.2007.02.025.
56. Zhang D, Yang J, Bao S, et al., 2013, Semiconductor Nanoparticle-based Hydrogels Prepared Via Self-initiated Polymerization Under Sunlight, Even Visible Light. Sci Rep, 3:1399. DOI: 10.1038/srep01399.
57. Vaezi M, Chianrabutra S, Mellor B, et al., 2013. Multiple Material Additive Manufacturing-Part 1: A Review. Virtual Phys Prototyp, 8:19–50. DOI: 10.1080/17452759.2013.778175.
58. Cheng K, Mukherjee P, Curthoys I, 2017, Development and Use of Augmented Reality and 3D Printing in Consulting Patient with Complex Skull Base Cholesteatoma. Virtual Phys Prototyp, 12:241–8. DOI: 10.1080/17452759.2017.1310050.
59. Espalin D, Muse DW, MacDonald E, et al., 2014, 3D Printing Multifunctionality: Structures with Electronics. Int J Adv Manuf Technol, 72:963–78. DOI: 10.1007/s00170-014-5717-7.
60. Zhao D, Liu T, Park JG, 2012, Conductivity Enhancement of Aerosol-jet Printed Electronics by Using Silver Nanoparticles Ink with Carbon Nanotubes. Microelectron Eng, 96:71–5. DOI: 10.1016/j.mee.2012.03.004.
61. Wang S, Lee JM, Yeong WY, 2015, Smart Hydrogels for 3D Bioprinting. Int J Bioprint, 1:3–14.
62. Murphy SV, Atala A, 2014, 3D Bioprinting of Tissues and Organs. Nat Biotechnol, 32:773–85. DOI: 10.1038/nbt.2958.
63. Cui H, Nowicki M, Fisher JP, et al., 2017, 3D Bioprinting for Organ Regeneration. Adv Healthc Mater, 6: 1601118. DOI: 10.1002/adhm.201601118.
64. Lee JM, Ng WL, Yeong WY, 2019, Resolution and Shape in Bioprinting: Strategizing Towards Complex Tissue and Organ Printing. Appl Phys Rev, 6(1):011307. DOI: 10.1063/1.5053909.
65. Landers R, Mülhaupt R, 2000, Desktop Manufacturing of Complex Objects, Prototypes and Biomedical Scaffolds by Means of Computer-assisted Design Combined with Computer-guided 3D Plotting of Polymers and Reactive Oligomers. Macromol Mater Eng, 282:17–21. DOI: 10.1002/1439-2054(20001001)282:1<17: aidmame17>3.0.co;2-8.
66. Sun J, Ng JH, Fuh YH, et al., 2009, Comparison of Micro-dispensing Performance Between Micro-valve and Piezoelectric Printhead. Microsyst Technol, 15:1437–48. DOI: 10.1007/s00542-009-0905-3.
67. Chua CK, Leong KF, 2015, 3D Printing and Additive Manufacturing: Principles and Applications. 4th ed. Singapore: World Scientific Publishing.
68. Luo Y, Lode A, Akkineni AR, et al., 2015, Concentrated Gelatin/alginate Composites for Fabrication of Predesigned Scaffolds with a Favorable Cell Response by 3D Plotting. RSC Adv, 5:43480–8. DOI: 10.1039/c5ra04308e.
69. Sun C, Fang N, Wu DM, et al., 2005, Projection Micro stereolithography Using Digital Micro-mirror Dynamic Mask. Sens Actuators a Phys, 121:113–20. DOI: 10.1016/j.sna.2004.12.011.
70. Hölzl K, Lin S, Tytgat L, et al., 2016, Bioink Properties Before, During and after 3D Bioprinting. Biofabrication, 8:032002. DOI: 10.1088/1758-5090/8/3/032002.
71. Jang TS, Jung HD, Pan HM, et al., 2017, 3D Printing of Hydrogel Composite Systems: Recent Advances in Technology for Tissue Engineering. Int J Bioprint, 4:1–28.
72. Donderwinkel I, van Hest JC, Cameron NR, 2017, Bio-inks for 3D Bioprinting: Recent Advances and Future Prospects. Polym Chem, 8:4451–71. DOI: 10.1039/c7py00826k.
73. Sayyar S, Gambhir S, Chung J, et al., 2017, 3D Printable Conducting Hydrogels Containing Chemically Converted Graphene. Nanoscale, 9:2038–50. DOI: 10.1039/c6nr07516a.
74. Fantino E, Roppolo I, Zhang D, et al., 2018, 3D Printing/Interfacial Polymerization Coupling for the Fabrication of Conductive Hydrogel. Macromol Mater Eng, 303:1700356. DOI: 10.1002/mame.201700356.
75. Odent J, Wallin TJ, Pan W, et al., 2017, Highly Elastic, Transparent, and Conductive 3D-Printed Ionic Composite Hydrogels. Adv Funct Mater, 27:1701807. DOI: 10.1002/adfm.201701807.
76. Zhou Y, Layani M, Wang S, et al., 2018, Fully Printed Flexible Smart Hybrid Hydrogels. Adv Funct Mater, 28:1705365. DOI: 10.1002/adfm.201705365.
77. Heo DN, Lee SJ, Timsina R, et al., 2019, Development of 3D Printable Conductive Hydrogel with Crystallized PEDOT: PSS for Neural Tissue Engineering. Mater Sci Eng C Mater Biol Appl, 99:582–90. DOI: 10.1016/j.msec.2019.02.008.
78. Sekine S, Ido Y, Miyake T, et al., 2010, Conducting Polymer Electrodes Printed on Hydrogel. J Am Chem Soc, 132:13174–5. DOI: 10.1021/ja1062357.
79. Ahn Y, Lee H, Lee D, et al., 2014, Highly Conductive and Flexible Silver Nanowire-Based Microelectrodes on Biocompatible Hydrogel. ACS Appl Mater Interfaces, 6:18401–7. DOI: 10.1021/am504462f.
80. Shay T, Velev OD, Dickey MD, 2018, Soft Electrodes Combining Hydrogel and Liquid Metal. Soft Matter, 14:3296–303. DOI: 10.1039/c8sm00337h.
81. Agarwala S, Lee JM, Ng WL, et al., 2018, A Novel 3D Bioprinted Flexible and Biocompatible Hydrogel Bioelectronic Platform. Biosens Bioelectron, 102:365–71. DOI: 10.1016/j.bios.2017.11.039.
82. Jaffari SA, Turner AP, 1995, Recent Advances in Amperometric Glucose Biosensors for In vivo Monitoring. Physiol Meas, 16:1–15. DOI: 10.1088/0967-3334/16/1/001.
83. Wisniewski N, Moussy F, Reichert WM, 2000, Characterization of Implantable Biosensor Membrane Biofouling. Fresenius J Anal Chem, 366:611–21. DOI: 10.1007/s002160051556.
84. Abraham S, Brahim S, Ishihara K, et al., 2005, Molecularly Engineered p(HEMA)-Based Hydrogels for Implant Biochip Biocompatibility. Biomaterials, 26:4767–78. DOI: 10.1016/j.biomaterials.2005.01.031.
85. Saha S, Sarkar P, Sarkar M, et al., 2015, Electroconductive Smart Polyacrylamide-polypyrrole (PAC-PPY) Hydrogel: A Device for Controlled Release of Risperidone. RSC Adv, 5, 27665–73. DOI: 10.1039/c5ra03535j.
86. Tao Y, Cheng G, Zhang M, et al., 2015, A General Route to 2D Nanoleaves and Nanoplates of Polyaniline. Russ J Phys Chem A, 89:2267–70.
87. Sharma K, Kaith BS, Kumar V, et al., 2014, Gum Ghatti Based Novel Electrically Conductive Biomaterials: A Study of Conductivity and Surface Morphology. Express Polym Lett, 8:267–81. DOI: 10.3144/expresspolymlett.2014.30.
88. Sharma K, Kumar V, Kaith BS, et al., 2015, Evaluation of Conducting Interpenetrating Network Based on Gum Ghatti-G-Poly (Acrylic Acid-Aniline) As Colon-Specific Delivery For Amoxicillin Trihydrate And Paracetamol. New J Chem,39:3021–34. DOI: 10.1039/c4nj01982b.
89. Sharma K, Kumar V, Chaudhary B, et al., 2016, Application of Biodegradable Superabsorbent Hydrogel Composite Based on Gum Ghatti-co-poly (Acrylic Acid-aniline) for Controlled Drug Delivery. Polym Degrad Stab, 124:101–11. DOI: 10.1016/j.polymdegradstab.2015.12.021.
90. Tandon B, Magaz A, Balint R, et al., 2018, Electroactive Biomaterials: Vehicles for Controlled Delivery of Therapeutic Agents for Drug Delivery and Tissue Regeneration. Adv Drug Deliv Rev, 129:148–68. DOI: 10.1016/j.addr.2017.12.012.
91. Weaver CL, LaRosa JM, Luo X, et al., 2014, Electrically Controlled Drug Delivery from Graphene Oxide Nanocomposite Films. ACS Nano, 8:1834–43. DOI: 10.1021/nn406223e.
92. Catt K, Li H, Hoang V, et al., 2018, Self-powered Therapeutic Release from Conducting Polymer/graphene Oxide Films on Magnesium. Nanomed Nanotechnol Biol Med, 14:2495–503. DOI: 10.1016/j.nano.2017.02.021.
93. Balint R, Cassidy NJ, Cartmell SH, 2014, Conductive Polymers: Towards a Smart Biomaterial for Tissue Engineering. Acta Biomater, 10:2341–53. DOI: 10.1016/j.actbio.2014.02.015.
94. Kawahara Y, Yamaoka K, Iwata M, et al., 2006, Novel Electrical Stimulation Sets the Cultured Myoblast Contractile Function to On. Pathobiology, 73:288–94. DOI: 10.1159/000099123.
95. Pedrotty DM, Koh J, Davis BH, et al., 2005, Engineering Skeletal Myoblasts: Roles of Three-dimensional Culture and Electrical Stimulation. Am J Physiol Heart Circ Physiol, 288:H1620–6. DOI: 10.1152/ajpheart.00610.2003