Combined Porogen Leaching and Emulsion Templating to produce Bone Tissue Engineering Scaffolds
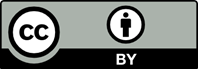
Bone has a hierarchy of porosity that is often overlooked when creating tissue engineering scaffolds where pore sizes are typically confined to a single order of magnitude. High internal phase emulsion (HIPE) templating produces polymerized HIPEs (polyHIPEs): highly interconnected porous polymers which have two length scales of porosity covering the 1–100 µm range. However, additional larger scales of porosity cannot be introduced in the standard emulsion formulation. Researchers have previously overcome this by additively manufacturing emulsions; fabricating highly microporous struts into complex macroporous geometries. This is time consuming and expensive; therefore, here we assessed the feasibility of combining porogen leaching with emulsion templating to introduce additional macroporosity. Alginate beads between 275 and 780 µm were incorporated into the emulsion at 0, 50, and 100 wt%. Once polymerized, alginate was dissolved leaving highly porous polyHIPE scaffolds with added macroporosity. The compressive modulus of the scaffolds decreased as alginate porogen content increased. Cellular performance was assessed using MLO-A5 post-osteoblasts. Seeding efficiency was significantly higher and mineralized matrix deposition was more uniformly deposited throughout porogen leached scaffolds compared to plain polyHIPEs. Deep cell infiltration only occurred in porogen leached scaffolds as detected by histology and lightsheet microscopy. This study reveals a quick, low cost and simple method of producing multiscale porosity scaffolds for tissue engineering.
1. Wu S, Liu X, Yeung KW, et al., 2014, Biomimetic Porous Scaffolds for Bone Tissue Engineering. Materials Sci Eng R Rep, 80:1–36.
2. Gupta D, Singh AK, Dravid A, et al., 2019, Multiscale Porosity in Compressible Cryogenically 3D Printed Gels for Bone Tissue Engineering. ACS Appl Mater Interfaces, 11:20437–52. DOI: 10.1021/acsami.9b05460
3. Rustom LE, Boudou T, Nemke BW, et al., 2017, Multiscale Porosity Directs Bone Regeneration in Biphasic Calcium Phosphate Scaffolds. ACS Biomater Sci Eng, 3:2768–78. DOI: 10.1021/acsbiomaterials.6b00632
4. Woodard JR, Hilldore AJ, Lan SK, et al., 2007, The Mechanical Properties and Osteo conductivity of Hydroxyapatite Bone Scaffolds with Multi-scale Porosity. Biomaterials, 28:45–54. DOI: 10.1016/j.biomaterials.2006.08.021
5. Roosa SM, Kemppainen JM, Moffitt EN, et al., 2010, The Pore Size of Polycaprolactone Scaffolds has Limited Influence on Bone Regeneration in an in vivo Model. J Biomed Mater Res A, 92:359–68. DOI: 10.1002/jbm.a.32381
6. Vand K, Kaplan D, 2005, Porosity of 3D Biomaterial Scaffolds and Osteogenesis. Biomaterials, 26:5474–91. DOI:10.1016/j.biomaterials.2005.02.002
7. Land LQ, Choong C, 2013, Three-dimensional Scaffolds for Tissue Engineering Applications: Role of Porosity and Pore Size. Tissue Eng Part B Rev, 19:485-502. DOI: 10.1089/ten.teb.2012.0437
8. Owen R, Sherborne C, Paterson T, et al., 2016, Emulsion Templated Scaffolds with Tunable Mechanical Properties for Bone Tissue Engineering. J Mech Behav Biomed Mater, 54:159–72. DOI: 10.1016/j.jmbbm.2015.09.019
9. Sherborne C, Owen R, Reilly GC, et al., 2018, Light-based Additive Manufacturing of PolyHIPEs: Controlling the Surface Porosity for 3D Cell Culture Applications. Mater Des, 156:494–503. DOI: 10.1016/j.matdes.2018.06.061
10. Malayeri A, Sherborne C, Paterson T, et al., 2016, Osteosarcoma growth on trabecular bone mimicking structures manufactured via laser direct write. Int J Bioprint, 2:67–72. DOI: 10.18063/ijb.2016.02.005
11. Owen R, Sherborne C, Reilly GC, et al., 2015, Data for the Analysis of PolyHIPE Scaffolds with Tunable Mechanical Properties for Bone Tissue Engineering. Data Brief, 5:616–20. DOI: 10.1016/j.dib.2015.09.051
12. Paterson TE, Gigliobianco G, Sherborne C, et al., 2018, Porous Microspheres Support Mesenchymal Progenitor Cell Ingrowth and Stimulate Angiogenesis. APL Bioeng, 2:026103. DOI: 10.1063/1.5008556
13. Wang AJ, Paterson T, Owen R, et al., 2016, Photocurable High Internal Phase Emulsions (HIPEs) Containing Hydroxyapatite for Additive Manufacture of Tissue Engineering Scaffolds with Multi-scale Porosity. Mater Sci Eng C, 67:51–8. DOI: 10.1016/j.msec.2016.04.087
14. Whitely M, Rodriguez-Rivera G, Waldron C, et al., 2019, Porous PolyHIPE Microspheres for Protein Delivery from an Injectable Bone Graft. Acta Biomat, 93:169–79. DOI: 10.1016/j.actbio.2019.01.044
15. Lee A, Langford CR, Rodriguez-Lorenzo LM, et al., 2017, Bioceramic Nanocomposite Thiol-acrylate polyHIPE Scaffolds for Enhanced Osteoblastic Cell Culture in 3D. Biomat Sci, 5:2035–47. DOI: 10.1039/c7bm00292k
16. Dikici BA, Reilly GC, Claeyssens F, 2020, Boosting the Osteogenic and Angiogenic Performance of Multiscale Porous Polycaprolactone Scaffolds by in vitro Generated Extracellular Matrix Decoration. ACS Appl Mater Interfaces, 12:12510–24. DOI: 10.1021/acsami.9b23100
17. Dikici BA, Sherborne C, Reilly GC, et al., 2019, Emulsion Templated Scaffolds Manufactured from Photocurable Polycaprolactone. Polymer, 175:243–54. DOI: 10.1016/j.polymer.2019.05.023
18. Dikici BA, Dikici S, Reilly GC, et al., 2019, A Novel Bilayer Polycaprolactone Membrane for Guided Bone Regeneration: Combining Electrospinning and Emulsion Templating. Materials (Basel), 12:12162643. DOI: 10.3390/ma12162643
19. Cameron NR, 2005, High Internal Phase Emulsion Templating as a Route to Well-defined Porous Polymers. Polymer, 46:1439–49. DOI: 10.1016/j.polymer.2004.11.097
20. Krajnc PH, 2014, PolyHIPEs from Methyl Methacrylate: Hierarchically Structured Microcellular Polymers with Exceptional Mechanical Properties. Polymer, 55:4420–4. DOI: 10.1016/j.polymer.2014.07.007
21. Iand G, Silverstein MS, 2010, Polymerized pickering HIPEs: Effects of synthesis parameters on porous structure. J Polym Sci Part A Polym Chem, 48:1516–25. DOI: 10.1002/pola.23911
22. Bokhari M, Carnachan RJ, Przyborski SA, et al., 2007, Emulsion-templated Porous Polymers as Scaffolds for Three Dimensional Cell Culture: Effect of Synthesis Parameters on Scaffold Formation and Homogeneity. J Mater Chem, 17:4088–94. DOI: 10.1039/b707499a
23. Carnachan RJ, Bokhari M, Przyborski SA, et al., 2006, Tailoring the Morphology of Emulsion-templated Porous Polymers. Soft Matter, 2:608–16. DOI: 10.1039/b603211g
24. Richez A, Deleuze H, Vedrenne P, et al., 2005, Preparation of Ultra-low-density Microcellular Materials. J Appl Polym Sci, 96:2053–63. DOI: 10.1002/app.21668
25. Xu H, Zheng X, Huang Y, et al., 2016, Interconnected Porous Polymers with Tunable Pore Throat Size Prepared via Pickering High Internal Phase Emulsions. Langmuir, 32:38–45. DOI: 10.1021/acs.langmuir.5b03037
26. Williams JM, Gray AJ, Wilkerson MH, 1990, Emulsion Stability and Rigid Foams from Styrene or Divinylbenzene Water-in-oil Emulsions. Langmuir, 6:437–44. DOI: 10.1021/la00092a026
27. Williams JM, Wrobleski DA, 1988, Spatial Distribution of the Phases in Water-in-oil Emulsions. Open and Closed Microcellular Foams from Cross-linked Polystyrene. Langmuir, 4:656–62. DOI: 10.1021/la00081a027
28. Robinson JL, Moglia RS, Stuebben MC, et al., 2013, Achieving Interconnected Pore Architecture in Injectable PolyHIPEs for Bone Tissue Engineering. Tissue Eng Part A, 20:1103–12. DOI: 10.1089/ten.tea.2013.0319
29. Knight E, Murray B, Carnachan R, et al., 2011, Alvetex®: Polystyrene Scaffold Technology for Routine Three Dimensional Cell Culture. In: Haycock JW, editor. 3D Cell Culture: Methods and Protocols. Humana Press, Totowa, NJ.pp. 323–40. DOI: 10.1007/978-1-60761-984-0_20
30. Viswanathan P, Johnson DW, Hurley C, et al., 2014, 3D Surface Functionalization of Emulsion-Templated Polymeric Foams. Macromolecules, 47:7091–8. DOI: 10.1021/ma500968q
31. Cameron NR, Sherrington DC, Albiston L, et al., 1996, Study of the Formation of the Open-cellular Morphology of Poly(styrene/divinylbenzene) polyHIPE Materials by cryo-SEM. Colloid Polym Sci, 274:592–5. DOI: 10.1007/bf00655236
32. Stevens M Mand George J H, 2005, Exploring and Engineering the Cell Surface Interface. Science, 310:1135–8.
33. Sears NA, Dhavalikar PS, Cosgriff-Hernandez EM, 2016, Emulsion Inks for 3D Printing of High Porosity Materials. Macromol Rapid Commun, 37:1369–74. DOI: 10.1002/marc.201600236
34. Sears N, Dhavalikar P, Whitely M, et al., 2017, Fabrication of Biomimetic Bone Grafts with Multi-material 3D Printing. Biofabrication, 9:025020. DOI: 10.1088/1758-5090/aa7077
35. Susec M, Ligon SC, Stampfl J, et al., 2013, Hierarchically Porous Materials from Layer-by-layer Photopolymerization of High Internal Phase Emulsions. Macromol Rapid Commun, 34:938–43. DOI: 10.1002/marc.201300016
36. Johnson DW, Sherborne C, Didsbury MP, et al., 2013, Macrostructuring of Emulsion-templated Porous Polymers by 3D Laser Patterning. Adv Mater, 25:3178–81. DOI: 10.1002/adma.201300552
37. Lee JM, Ng WL, Yeong WY, 2019, Resolution and Shape in Bioprinting: Strategizing Towards Complex Tissue and Organ Printing. Appl Phys Rev, 6:011307. DOI: 10.1063/1.5053909
38. Ng WL, Lee JM, Zhou M, et al., 2020, Vat Polymerizationbased Bioprinting Process, Materials, Applications and Regulatory Challenges. Biofabrication, 12:022001. DOI: 10.1088/1758-5090/ab6034
39. Zhang J, Hu Q, Wang S, et al., 2020, Digital Light Processing Based Three-dimensional Printing for Medical Applications. Int J Bioprint, 6:1–10.
40. Thadavirul N, Pavasant P, Supaphol P, 2014, Development of Polycaprolactone Porous Scaffolds by Combining Solvent Casting, Particulate Leaching, and Polymer Leaching Techniques for Bone Tissue Engineering. J Biomed Mater Res Part A, 102:3379–92. DOI: 10.1002/jbm.a.35010
41. Kim TG, Chung HJ, Park TG, 2008, Macroporous and Nanofibrous Hyaluronic Acid/collagen Hybrid Scaffold Fabricated by Concurrent Electrospinning and Deposition/leaching of Salt Particles. Acta Biomater, 4:1611–9. DOI: 10.1016/j.actbio.2008.06.008
42. Sin D, Miao X, Liu G, et al., 2010, Polyurethane (PU) Scaffolds Prepared by Solvent Casting/particulate Leaching (SCPL) Combined with Centrifugation. Mater Sci Eng C, 30:78–85. DOI: 10.1016/j.msec.2009.09.002
43. Bencherif SA, Braschler TM, Renaud P, 2013, Advances in the Design of Macroporous Polymer Scaffolds for Potential Applications in Dentistry. J Periodontal Implant Sci, 43:251–61. DOI: 10.5051/jpis.2013.43.6.251
44. Hutmacher DW, 2001, Scaffold Design and Fabrication Technologies for Engineering Tissues State of the Art and Future Perspectives. J Biomater Sci Polym Ed, 12:107–24.
45. Venkatesan J, Bhatnagar I, Manivasagan P, et al., 2015, Alginate Composites for Bone Tissue Engineering: A Review. Int J Biol Macromol, 72:269–81.
46. Schindelin J, Arganda-Carreras I, Frise E, et al., 2012, Fiji: An Open-source Platform for Biological-image Analysis. Nat Methods, 9:676–82. DOI: 10.1038/nmeth.2019
47. Schneider CA, Rasband WS, Eliceiri KW, 2012, NIH Image to Image J: 25 Years of Image Analysis. Nat Methods, 9:671–5. DOI: 10.1038/nmeth.2089
48. Owen R, Bahmaee H, Claeyssens F, et al., 2020, Comparison of the Anabolic Effects of Reported Osteogenic Compounds on Human Mesenchymal Progenitor-derived Osteoblasts. Bioengineering (Basel), 7:7010012. DOI: 10.3390/bioengineering7010012
49. Linkert M, Rueden CT, Allan C, et al., 2010, Metadata Matters: Access to Image Data in the Real World. J Cell Biol, 189:777–82.
50. Paljevac M, Gradišnik L, Lipovšek S, et al., 2018, MultipleLevel Porous Polymer Monoliths with Interconnected Cellular Topology Prepared by Combining Hard Sphere and Emulsion Templating for Use in Bone Tissue Engineering. Macromol Biosci, 18:1700306. DOI: 10.1002/mabi.201700306
51. Hayward AS, Eissa AM, Maltman DJ, et al., 2013, Galactosefunctionalized PolyHIPE Scaffolds for Use in Routine Three Dimensional Culture of Mammalian Hepatocytes. Biomacromolecules, 14:4271–7. DOI: 10.1021/bm401145x
52. Akay G, Birch MA, Bokhari MA, 2004, Microcellular polyhipe Polymer Supports Osteoblast Growth and Bone Formation in vitro. Biomaterials, 25:3991–4000. DOI: 10.1016/j.biomaterials.2003.10.086
53. Workman VL, Dunnett SB, Kille P, et al., 2008, On-Chip Alginate Microencapsulation of Functional Cells. Macromol Rapid Commun, 29:165–70. DOI: 10.1002/marc.200700641
54. Beck GR Jr., Zerler B, Moran E, 2001, Gene Array Analysis of Osteoblast Differentiation. Cell Growth Differ, 12:61–83.