New microorganism isolation techniques with emphasis on laser printing
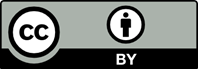
The study of biodiversity, growth, development, and metabolism of cultivated microorganisms is an integral part of modern microbiological, biotechnological, and medical research. Such studies require the development of new methods of isolation, cultivation, manipulation, and study of individual bacterial cells and their consortia. To this end, in recent years, there has been an active development of different isolation and three-dimensional cell positioning methods. In this review, the optical tweezers, surface heterogeneous functionalization, multiphoton lithography, microfluidic techniques, and laser printing are reviewed. Laser printing is considered as one of the most promising techniques and is discussed in detail.
1. Vartoukian S R, Palmer R M, Wade W G, 2010, Strategies for culture of “unculturable” bacteria. FEMS Microbiol Lett, 309(1): 1–7. https://doi.org/10.1111/j.1574- 6968.2010.02000.x.
2. Whitman W B, Coleman D C, Wiebe W J, 1998, Prokaryotes: The unseen majority. Proc Natl Acad Sci, 95(12): 6578–6583. https://doi.org/10.1073/pnas.95.12.6578.
3. Head I M, Saunders J R, Pickup R W, 1998, Microbial evolution, diversity, and ecology: A decade of ribosomal RNA analysis of uncultivated microorganisms. Microb Ecol, 35(1): 1–21. https://doi.org/10.1007/s002489900056.
4. Alain K, Querellou J, 2009, Cultivating the uncultured: Limits, advances and future challenges. Extremophiles, 13(4): 583–594. https://doi.org/10.1007/s00792-009-0261-3.
5. Sogin M L, Morrison H G, Huber J A, et al., 2011, Microbial diversity in the deep sea and the underexplored “rare biosphere”. Handb Mol Microb Ecol II Metagenomics Differ Habitats, 2011(30): 243–252.
6. Hultman J, Waldrop M P, Mackelprang R, et al., 2015, Multi-omics of permafrost, active layer and thermokarst bog soil microbiomes. Nature, 521(7551): 208–212. https://doi. org/10.1038/nature14238.
7. Jansson J K, Taş N, 2014, The microbial ecology of permafrost. Nat Rev Microbiol, 12(6): 414–425. https://doi. org/10.1038/nrmicro3262.
8. Pham V H T, Kim J, 2012, Cultivation of unculturable soil bacteria. Trends Biotechnol, 30(9): 475–484. https://doi. org/10.1016/j.tibtech.2012.05.007.
9. Shao X, Mugler A, Kim J, et al., 2017, Nemenman I. Growth of bacteria in 3-d colonies. PLoS Comput Biol, 13(7): 1–19. https://doi.org/10.1371/journal.pcbi.1005679.
10. Kohanim Y K, Levi D, Jona G, et al., 2018, A bacterial growth law out of steady state. Cell Rep, 23(10): 2891–2900. https:// doi.org/10.1016/j.celrep.2018.05.007.
11. Scott M, Hwa T, 2011, Bacterial growth laws and their applications. Curr Opin Biotechnol, 22(4): 559–565. https:// doi.org/10.1016/j.copbio.2011.04.014.
12. Coleman D C, Callaham M A, Crossley D A Jr., 2017, Fundamentals of Soil Ecology. London: Academic Press.
13. Singh B K, Bardgett R D, Smith P, et al., 2010, Microorganisms and climate change: Terrestrial feedbacks and mitigation options. Nat Rev Microbiol, 8(11): 779–790. https://doi. org/10.1038/nrmicro2439.
14. Ling L L, Schneider T, Peoples A J, et al., 2015, A new antibiotic kills pathogens without detectable resistance. Nature, 517(7535): 455–459. https://doi.org/10.1038/ nature14098.
15. Goodfellow M, Nouioui I, Sanderson R, et al., 2018, Rare taxa and dark microbial matter: Novel bioactive actinobacteria abound in Atacama desert soils. Antonie van Leeuwenhoek, 111(8): 1315–1332. https://doi.org/10.1007/ s10482-018-1088-7.
16. Hattori T, Hattori R, 1976, The physical environemnt in soil microbiology: An attempt to extend priciples of microbiology to soil microorgansims. CRC Crit Rev Microbiol, 4: 423-461. https://doi.org/10.3109/10408417609102305.
17. Stotzky G, 1986, Interactions of soil minerals with natural organics and microbes. In: Huang P M, Schnitzer M, editors. Influence of Soil Mineral Colloids on Metabolic Processes, Growth, Adhesion, and Ecology of Microbes and Viruses. Madison, WI, USA: Soil Science Society of America. pp305–428.
18. Torsvik V, Øvreås L, 2008, Microbial diversity, life strategies, and adaptation to life in extreme soils. Microbiol Extrem Soils, 13: 373. https://doi.org/10.1007/978-3-540-74231-9_2.
19. Fajardo A, Martínez J L, 2008, Antibiotics as signals that trigger specific bacterial responses. Curr Opin Microbiol, 11(2): 161–167. https://doi.org/10.1016/j.mib.2008.02.006.
20. García-Contreras R, Nuñez-López L, Jasso-Chávez R, et al., 2015, Quorum sensing enhancement of the stress response promotes resistance to quorum quenching and prevents social cheating. ISME J, 9(1): 115–125. https://doi.org/10.1038/ ismej.2014.98.
21. El-Registan G I, Mulyukin A L, Nikolaev Y A, et al., 2005, The role of microbial low-molecular-weight autoregulatory factors (alkylhydroxybenzenes) in resistance of microorganisms to radiation and heat shock. Adv Space Res, 36(9): 1718–1728. https://doi.org/10.1016/j.asr.2005.02.070.
22. El-Registan G I, Mulyukin A L, Nikolaev Y A, et al., 2006, Adaptogenic functions of extracellular autoregulators of microorganisms. Microbiology, 75(4): 380–389. https://doi. org/10.1134/S0026261706040035.
23. Decho A W, 2000, Microbial biofilms in intertidal systems: An overview. Cont Shelf Res, 20(10-11): 1257–1273. https:// doi.org/10.1016/S0278-4343(00)00022-4.
24. Joint I, Mühling M, Querellou J, 2010, Culturing marine bacteria-an essential prerequisite for biodiscovery: Minireview. Microb Biotechnol, 3(5): 564–575. https://doi. org/10.1111/j.1751-7915.2010.00188.x.
25. Davis K E R, Joseph S J, Peter H, et al., 2005, Effects of growth medium, inoculum size, and incubation time on culturability and isolation of soil bacteria effects of growth medium, inoculum size, and incubation time on culturability and isolation of soil bacteria. Appl Environ Microbiol, 71(2): 826–834. https://doi.org/10.1128/AEM.71.2.826-834.2005.
26. Pulschen A A, Bendia A G, Fricker A D, et al., 2017, Isolation of uncultured bacteria from antarctica using long incubation periods and low nutritional media. Front Microbiol, 8: 1–12. https://doi.org/10.3389/fmicb.2017.01346.
27. Hynes W F, Chacón J, Segrè D, et al., 2018, Bioprinting microbial communities to examine interspecies interactions in time and space. Biomed Phys Eng Express, 4(5): 055010.
28. Bollmann A, Lewis K, Epstein S S, 2007, Incubation of environmental samples in a diffusion chamber increases the diversity of recovered isolates. Appl Environ Microbiol, 73(20): 6386–6390. https://doi.org/10.1128/AEM.01309-07.
29. Ferrari B C, Winsley T, Gillings M, et al., 2008, Cultivating previously uncultured soil bacteria using a soil substrate membrane system. Nat Protoc, 3(8): 1261–1269. https://doi. org/10.1038/nprot.2008.102.
30. Kushmaro, A., Geresh, S. 2012. U.S. Patent No. 8,158,401. Washington, DC: U.S. Patent and Trademark Office.
31. Nichols D, Cahoon N, Trakhtenberg E M, et al., 2010, Use of ichip for high-throughput in situ cultivation of “uncultivable” microbial species. Appl Environ Microbiol, 76(8): 2445– 2450. https://doi.org/10.1128/AEM.01754-09.
32. Ingham C J, Sprenkels A, Bomer J, et al., 2007, The micro-petri dish, a million-well growth chip for the culture and high-throughput screening of microorganisms. Proc Natl Acad Sci, 104(46): 18217–18222. https://doi.org/10.1073/ pnas.0701693104.
33. Fröhlich J, König H, 2000, New techniques for isolation of single prokaryotic cells. FEMS Microbiol Rev, 24(5): 567–572. https://doi.org/10.1016/S0168-6445(00)00045-0; https://doi.org/10.1111/j.1574-6976.2000.tb00558.x.
34. Ishii S, Tago K, Senoo K, 2010, Single-cell analysis and isolation for microbiology and biotechnology: Methods and applications. Appl Microbiol Biotechnol, 86(5): 1281–1292. https://doi.org/10.1007/s00253-010-2524-4.
35. Akselrod G M, Timp W, Mirsaidov U, et al., 2006, Laser-guided assembly of heterotypic three-dimensional living cell microarrays. Biophys J, 91(9): 3465–3473. https://doi. org/10.1529/biophysj.106.084079.
36. Rowan B, Wheeler M A, Crooks R M, 2002, Patterning bacteria within hyperbranched polymer film templates. Langmuir, 18(25): 9914–9917. https://doi.org/10.1021/la020664h.
37. Weibel D B, Lee A, Mayer M, et al., 2005, Bacterial printing press that regenerates its ink: Contact-printing bacteria using hydrogel stamps. Langmuir, 21(14): 6436–6442. https://doi. org/10.1021/la047173c.
38. Xu L, Robert L, Ouyang Q, et al., 2007, Microcontact printing of living bacteria arrays with cellular resolution. Nano Lett, 7(7): 2068–2072. https://doi.org/10.1021/nl070983z.
39. Eun Y J, Utada A S, Copeland M F, et al., 2011, Encapsulating bacteria in agarose microparticles using microfluidics for high-throughput cell analysis and isolation. ACS Chem Biol, 6(3): 260–266. https://doi.org/10.1021/cb100336p.
40. Volfson D, Cookson S, Hasty J, et al., 2008, Biomechanical ordering of dense cell populations. Proc Natl Acad Sci, 105(40): 15346–15351. https://doi.org/10.1073/pnas.0706805105.
41. Boedicker J Q, Vincent M E, Ismagilov R F, 2009, Microfluidic confinement of single cells of bacteria in small volumes initiates high-density behavior of quorum sensing and growth and reveals its variability. Angew Chemie Int Ed, 48(32): 5908–5911. https://doi.org/10.1002/anie.200901550.
42. Flickinger S T, Copeland M F, Downes E M, et al., 2011, Quorum sensing between Pseudomonas aeruginosa biofilms accelerates cell growth. J Am Chem Soc, 133: 5966–5975. https://doi.org/10.1021/ja111131f.
43. Carnes E C, Lopez D M, Donegan N P, et al., 2010, Confinement-induced quorum sensing of individual Staphylococcus aureus bacteria. Nat Chem Biol, 6(1): 41–45. https://doi.org/10.1038/nchembio.264.
44. Yaguchi T, Dwidar M, Byun C K, et al., 2012, Aqueous two-phase system-derived biofilms for bacterial interaction studies. Biomacromolecules, 13(9): 2655–2661. https://doi. org/10.1021/bm300500y.
45. Cho H J, Jönsson H, Campbell K, et al., 2007, Self-organization in high-density bacterial colonies: Efficient crowd control. PLoS Biol, 5(11): 2614–2623. https://doi. org/10.1371/journal.pbio.0050302.
46. Connell J L, Whiteley M, Shear J B, 2012, Sociomicrobiology in engineered landscapes. Nat Chem Biol, 8(1): 10–13. https:// doi.org/10.1038/nchembio.749.
47. Connell J L, Wessel A K, Parsek M R, 2010, Probing prokaryotic social behaviors with bacterial “lobster traps”. Mbio, 1(4): 1–8. https://doi.org/10.1128/mBio.00202-10.
48. Farsari M, Chichkov B N, 2009, Two-photon fabrication. Nat Photonics, 3: 450–452. https://doi.org/10.1038/ nphoton.2009.131.
49. Nielson R, Kaehr B, Shear J B, 2009, Microreplication and design of biological architectures using dynamic-mask multiphoton lithography. Small, 5(1): 120–125. https://doi. org/10.1002/smll.200801084.
50. Kawata S, Sun H B, Tanaka T, et al., 2001, Finer features for functional microdevices. Nature, 412(6848): 697–698. https://doi.org/10.1038/35089130.
51. Kim D, So P T C, 2010, High-throughput three-dimensional lithographic microfabrication. Opt Lett, 35(10): 1602. https:// doi.org/10.1364/OL.35.001602.
52. Connell J L, Ritschdorff E T, Whiteley M, et al., 2013, 3D printing of microscopic bacterial communities. Proc Natl Acad Sci, 110(46): 18380–18385. https://doi.org/10.1073/ pnas.1309729110.
53. Obara Y, Yamai S, Nikkawa T, et al., 1981, Preservation and transportation of bacteria by a simple gelatin disk method. J Clin Microbiol, 14(1): 61–66.
54. Kailas L, Ratcliffe E C, Hayhurst E J, et al., 2009, Immobilizing live bacteria for AFM imaging of cellular processes. Ultramicroscopy, 109(7): 775–780. https://doi. org/10.1016/j.ultramic.2009.01.012.
55. Jordan P, Leach J, Padgett M, et al., 2005, Creating permanent 3D arrangements of isolated cells using holographic optical tweezers. Lab Chip, 5(11): 1224–1228. https://doi. org/10.1039/b509218c.
56. Barron J A, Krizman D B, Ringeisen B R, 2005, Laser printing of single cells: Statistical analysis, cell viability, and stress. Ann Biomed Eng, 33(2): 121–130. https://doi.org/10.1007/ s10439-005-8971-x.
57. BarronJ A, WuP, LadouceurHD, etal., 2004, Biologicallaserprinting: A novel technique for creating heterogeneous 3-dimensional cell patterns. Biomed Microdevices, 6(2): 139–147. https://doi. org/10.1023/B: BMMD.0000031751.67267.9f.
58. Mironov V, Kasyanov V, Drake C, et al., 2008, Organ printing: Promises and challenges. Regen Med, 3(1): 93–103. https://doi.org/10.2217/17460751.3.1.93.
59. Fitzgerald L A, Wu P K, Gurnon J R, et al., 2010, Isolation of the phycodnavirus PBCV-1 by biological laser printing. J Virol Methods, 167(2): 223–225. https://doi.org/10.1016/j.jviromet.2010.04.005.
60. Ringeisen B R, Othon C M, Barron J A, et al., 2006, Jet-based methods to print living cells. Biotechnol J, 1(9): 930–948. https://doi.org/10.1002/biot.200600058.
61. Barron J A, Rosen R, Jones-Meehan J, et al., 2004, Biological laser printing of genetically modified Escherichia coli for biosensor applications. Biosens Bioelectron, 20(2): 246–252. https://doi.org/10.1016/j.bios.2004.01.011.
62. Barron J A, Young H D, Dlott D D, et al., 2005, Printing of protein microarrays via a capillary-free fluid jetting mechanism. Proteomics, 5(16): 4138–4144. https://doi. org/10.1002/pmic.200401294.
63. Taidi B, Lebernede G, Koch L, et al., 2016, Colony development of laser printed eukaryotic (yeast and microalga) microorganisms in co-culture. Int J Bioprinting, 2(2): 37–43. https://doi.org/10.18063/IJB.2016.02.001.
64. Unger C, Gruene M, Koch L, et al., 2011, Time-resolved imaging of hydrogel printing via laser-induced forward transfer. Appl Phys A Mater Sci Process, 103(2): 271–277. https://doi.org/10.1007/s00339-010-6030-4.
65. Gruene M, Unger C, Koch L, et al., 2011, Dispensing pico to nanolitre of a natural hydrogel by laser-assisted bioprinting. Biomed Eng Online, 10(3): 9–12. https://doi. org/10.1186/1475-925X-10-19.
66. Koch L, Kuhn S, Sorg H, et al., 2010, Laser printing of skin cells and human stem cells. Tissue Eng Part C Methods, 16(5): 847–854. https://doi.org/10.1089/ten.tec.2009.0397.
67. Deng Y, Renaud P, Guo Z, et al., 2017, Single cell isolation process with laser induced forward transfer. J Biol Eng, 11(1): 2. https://doi.org/10.1186/s13036-016-0045-0.
68. Ringeisen B R, Rincon K, Fitzgerald L A, et al., 2015, Printing soil: A single-step, high-throughput method to isolate micro-organisms and near-neighbour microbial consortia from a complex environmental sample. Methods Ecol Evol, 6(2): 209–217. https://doi.org/10.1111/2041-210X.12303.
69. Ringeisen B R, Lizewski S E, Fitzgerald L A, et al., 2010, Single cell isolation of bacteria from microbial fuel cells and potomac river sediment. Electroanalysis, 22(7-8): 875–882. https://doi.org/10.1002/elan.200880012.
70. Yusupov V I, Gorlenko M V, Cheptsov V S, et al., 2018, Laser engineering of microbial systems. Laser Phys Lett, 15(6): 065604. https://doi.org/10.1088/1612-202X/aab5ef.
71. Gorlenko M, Chutko E, Churbanova E, et al., 2018, Laser microsampling of soil microbial community. J Biol Eng Rev, 12: 27. https://doi.org/10.1186/s13036-018-0117-4.
72. Cheptsov V S, Churbanova E S, Yusupov V I, et al., 2018, Laser printing of microbial systems: Effect of absorbing metal film. Lett Appl Microbiol Rev, 67: 544-549. https://doi. org/10.1111/lam.13074.
73. Ara I, Kudo T, Matsumoto A, et al., 2007, Nonomuraea bangladeshensis Sp. Nov. and Nonomuraea coxensis Sp. Nov. Int J Syst Evol Microbiol, 57(7): 1504–1509. https://doi. org/10.1099/ijs.0.65054-0.
74. Sungthong R, Nakaew N, 2015, The genus Nonomuraea: A review of a rare actinomycete taxon for novel metabolites. J Basic Microbiol, 55(5): 554–565. https://doi.org/10.1002/ jobm.201300691.
75. Yusupov V I, Zhigarkov V S, Churbanova E S, et al., 2017, Laser-induced transfer of gel microdroplets for cell printing. Quantum Electron, 47(12): 1158–1165. https://doi. org/10.1070/QEL16512.
76. Kohli R, Bose B, Gupta P K, 2001, Induction of phr gene expression in E. coli strain KY706/pPL-1 by He-Ne laser (632.8 nm) irradiation. J Photochem Photobiol B Biol, 60(2-3): 136–142. https://doi.org/10.1016/S1011-1344(01)00139-7.
77. Nussbaum E L, Lilge L, Mazzulli T, 2002, Delivering radiant exposure of 1-50 J/cm 2 on three species of bacteria in vitro. Surgery, 20(6): 325–333.
78. Nussbaum E L, Lilge L, Mazzulli T, 2003, Effects of low-level laser therapy (LLLT) of 810 nm upon in vitro growth of bacteria: Relevance of irradiance and radiant exposure. J Clin Laser Med Surg, 21(5): 283–290. https://doi. org/10.1089/104454703322564497.
79. Chen Z, Lu J, Gao S H, et al., 2018, Silver nanoparticles stimulate the proliferation of sulfate reducing bacterium Desulfovibrio vulgaris. Water Res Pergamon, 129: 163–171. https://doi.org/10.1016/j.watres.2017.11.021.
80. Miazek K, Iwanek W, Remacle C, et al., 2015, Effect of metals, metalloids and metallic nanoparticles on microalgae growth and industrial product biosynthesis: A review. Int J Mol Sci, 16(10): 23929–23969. https://doi.org/10.3390/ ijms161023929.
81. Slavin Y N, Asnis J, Häfeli U O, et al., 2017, Metal nanoparticles: Understanding the mechanisms behind antibacterial activity. J Nanobiotechnol, 15(1): 1–20. https:// doi.org/10.1186/s12951-017-0308-z.
82. Schacht V J, Neumann L V, Sandhi S K, et al., 2013, Effects of silver nanoparticles on microbial growth dynamics. J Appl Microbiol, 114(1): 25–35. https://doi.org/10.1111/jam.12000.
83. Haider A J, Haider M J, Majed M D, et al., 2017, Effect of laser fluence on a microarray droplets micro-organisms cells by LIFT technique. Energy Procedia, 119: 256–263. https:// doi.org/10.1016/j.egypro.2017.07.078.
84. Ringeisen B R, Chrisey D B, Piqué A, et al., 2002, Generation of mesoscopic patterns of viable Escherichia coli by ambient laser transfer. Biomaterials, 23(1): 161–166. https://doi. org/10.1016/S0142-9612(01)00091-6.
85. Hopp B, Smausz T, Antal Z, et al., 2004, Absorbing film assisted laser induced forward transfer of fungi (Trichoderma conidia). J Appl Phys, 96(6): 3478–3481. https://doi. org/10.1063/1.1782275.
86. Francois K, Devlieghere F, Standaert A R, et al., 2003, Modelling the individual cell lag phase. Isolating single cells: Protocol development. Lett Appl Microbiol, 37(1): 26–30. https://doi.org/10.1046/j.1472-765X.2003.01340.x.
87. Gross A, Schoendube J, Zimmermann S, et al., 2015, Technologies for single-cell isolation. Int J Mol Sci, 16(8): 16897–16919. https://doi.org/10.3390/ijms160816897.
88. Höfer P, Vermette P, Groleau D, 2011, Introducing a new bioengineered bug: Methylobacterium extorquens tuned as a microbial bioplastic factory. Bioeng Bugs, 2(2): 71–79. https://doi.org/10.4161/bbug.2.2.15009.
89. Zhong C, Gurry T, Cheng A A, et al., 2014, Strong underwater adhesives made by self-assembling multi-protein nanofibres. Nat Nanotechnol, 9(10): 858–866. https://doi.org/10.1038/ nnano.2014.199.
90. Chen A Y, Deng Z, Billings A N, et al., 2014, Synthesis and patterning of tunable multiscale materials with engineered cells. Nat Mater, 13(5): 515–523. https://doi.org/10.1016/j. matdes.2013.08.029; https://doi.org/10.1038/nmat3912.
91. Lehner B A E, Schmieden D T, Meyer A S, 2017, A straightforward approach for 3D bacterial printing. ACS Synth Biol, 6(7): 1124–1130. https://doi.org/10.1021/ acssynbio.6b00395.