Coaxial nozzle-assisted electrohydrodynamic printing for microscale 3D cell-laden constructs
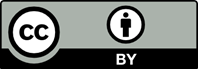
Cell printing has found wide applications in biomedical fields due to its unique capability in fabricating living tissue constructs with precise control over cell arrangements. However, it is still challenging to print cell-laden 3D structures simultaneously with high resolution and high cell viability. Here a coaxial nozzle-assisted electrohydrodynamic cell printing strategy was developed to fabricate living 3D cell-laden constructs. Critical process parameters such as feeding rate and stage moving speed were evaluated to achieve smaller hydrogel filaments. The effect of CaCl2 feeding rate on the printing of 3D alginate hydrogel constructs was also investigated. The results indicated that the presented strategy can print 3D hydrogel structures with relatively uniform filament dimension (about 80 μm) and cell distribution. The viability of the encapsulated cells was over 90%. We envision that the coaxial nozzle-assisted electrohydrodynamic printing will become a promising cell printing strategy to advance biomedical innovations.
[1]Kang H-W, Lee S J, Ko I K, et al., 2016, A 3D bioprinting system to produce human-scale tissue constructs with structural integrity. Nat Biotechno, 34(3): 313–319. http://dx.doi.org/10.1038/nbt.3413
[2]Jordan S M, Kelly R S, Michael T Y, et al., 2012, Rapid casting of patterned vascular networks for perfusable engineered three-dimensional tissues. Nat Mater, 11(9): 768–774. http://dx.doi.org/10.1038/nmat335733
[3]Falguni P, Jinah J, Dong-Heon H, et al., 2014, Printing three-dimensional tissue analogues with decellularized extracellular matrix bioink. Nat Commun, 5: 3935. http://dx.doi.org/10.1038/ncomms4935
[4]Sean V M, Anthony A, 2014, 3D bioprinting of tissues and organs. Nat Biotechnol, 32(8): 773–785. http://dx.doi.org/10.1038/nbt.2958
[5]Malda J, Jetze V, Ferry P M, et al., 2013, 25th Anniversary article: Engineering hydrogels for biofabrication. Adv Mater, 25(36): 5011–5028. http://dx.doi.org/10.1002/adma.201302042
[6]Thomas B, Mieke V, Jorg S, et al., 2012, A review of trends and limitations in hydrogel-rapid prototyping for tissue engineering. Biomaterials, 33(26): 6020–6041. http://dx.doi.org/10.1016/j.biomaterials.2012.04.050
[7]Xu T, Zhao W Z, Zhu J M, et al., 2013, Complex heterogeneous tissue constructs containing multiple cell types prepared by inkjet printing technology. Biomaterials, 34(1): 130–139. http://dx.doi.org/10.1016/j.biomaterials.2012.09.035
[8]Lothar K, Andrea D, Sabrina S, et al., 2012, Skin tissue generation by laser bioprinting. Biotechnology, 109(7): 1855–1863. http://dx.doi.org/10.1002/bit.24455
[9]Zhu W, Ma X Y, Gou M L, et al., 2016, 3D printing of functional biomaterials for tissue engineering. Curr Opin Biotechnol, 40: 103–112. http://dx.doi.org/10.1016/j.copbio.2016.03.014
[10]Ning LQ, Chen X B, 2017, A brief review of extrusion-based tissue scaffold bio-printing. Biotechnol J, 12(8): 1600671. http://dx.doi.org/10.1002/biot.201600671
[11]Koo Y, Kim G, 2016, New strategy for enhancing in situ cell viability of cell-printing process via piezoelectric transducer-assisted three-dimensional printing. Biofabrication, 8(2): 025010. http://dx.doi.org/10.1088/1758-5090/8/2/025010
[12]Zhang B, He J K, Li X, et al., 2016, Micro/nanoscale electrohydrodynamic printing: From 2D to 3D. Nanoscale, 8(34): 15376–15388. http://dx.doi.org/10.1039/c6nr04106j
[13]He J K, Xu FY, Cao Y, et al., 2015, Towards microscale electrohydrodynamic three-dimensional printing. J Phys D Appl Phys, 49(5): 055504. http://dx.doi.org/10.1088/0022-3727/49/5/055504
[14]He J K, Xu F Y, Dong R N, et al., 2016, Electrohydrodynamic 3D printing of microscale poly (ε-caprolactone) scaffolds with multi-walled carbon nanotubes. Biofabrication, 9(1): 015007. http://dx.doi.org/10.1088/1758-5090/aa53bc
[15]Mao M, He J K, Li X, et al., 2017, The emerging frontiers and applications of high-resolution 3D printing. Micromachines, 8(4): 113. http://dx.doi.org/10.3390/mi8040113
[16]Onses M S, Sutanto E, Ferreira P M, et al., 2015, Mechanisms, capabilities, and applications of high-resolution electrohydrodynamic jet printing. Small, 11(34): 4267–4266. http://dx.doi.org/10.1002/smll.201500593
[17]Lee H, Seong B, Jang Y, et al., 2014, Direct alignment and patterning of silver nanowires by electrohydrodynamic jet printing. Small, 10(19): 3918–3922. http://dx.doi.org/10.1002/smll.201400936
[18]Ahmad Z, Rasekh M, Edirisinghe M, 2010, Electrohydrodynamic direct writing of biomedical polymers and composites. Macromol Mater Eng, 295(4): 315–319. http://dx.doi.org/10.1002/mame.200900396
[19]Parajuli D, Koomsap P, Parkhi A A, et al., 2016, Experimental investigation on process parameters of near-field deposition of electrispinning-based rapid prototyping. Virtual Phys Prototyp, 11(3):193–207. http://dx.doi.org/10.1080/17452759.2016.1210314
[20]Wei C, Dong J, 2013, Direct fabrication of high-resolution three-dimensional polymeric scaffolds using electrohydrodynamic hot jet plotting. J Micromech Microeng, 23(2): 025017. http://dx.doi.org/10.1088/0960-1317/23/2/025017.
[21]Zheng G, Sun L L, Wang X, et al., 2016, Electrohydrodynamic direct-writing microfiber patterns under stretching. Appl Phys A Mater Sci Process, 122(2): 1–9. http://dx.doi.org/10.1007/s00339-015-9584-3
[22]Chanthakulchan A, Koomsap P, Parkhi, et al., 2015, Environmental effects in fiber fabrication using electrispinning-based rapid prototyping. Virtual Phys Prototyp, 10(4): 227–237. http://dx.doi.org/10.1080/17452759.2015.1112411
[23]Chanthakulchan A, Koomsap P, Auyson K, et al., 2015, Development of an electrospinning-based rapid prototyping for scaffold fabrication, Rapid Prototyp J, 21(3): 329–339. http://dx.doi.org/10.1108/RPJ-11-2013-0119
[24]Bisht GS, Ciulin C, Alireza M, et al., 2011, Controlled continuous patterning of polymeric nanofibers on three-dimensional substrates using low voltage near-field electrospinning. Nano Lett, 11(4): 1831–1837. http://dx.doi.org/10.1021/nl2006164
[25]Li J L, Cai YL, Guo Y L, et al., 2014, Fabrication of three-dimensional porous scaffolds with controlled filament orientation and large pore size via an improved E-jetting technique. J Biomed Mater Res B Appl Biomater, 102B(4): 651–658. http://dx.doi.org/10.1002/jbm.b.33043
[26]Bu N, Huang Y G, Wang X M, et al., 2012, Continuous tunable and oriented nanofiber direct-written by mechano-electrospinning. Mater Manuf Process, 27(12): 1318–132. http://dx.doi.org/10.1080/10426914.2012.700145
[27]Jayasinghe S N, 2013, Cell electrospinning: A novel tool for functionalizing fibres, scaffolds and membranes with living cells and other advanced materials for regenerative biology and medicine. Analyst, 138(8): 2215–2223. http://dx.doi.org/10.1039/c3an36599a
[28]Zhao X, He J K, Xu F Y, et al., 2016, Electrohydrodynamic printing: A potential tool for high-resolution hydrogel/cell patterning. Virtual Phys Prototyp, 11 (1): 57–63. http://dx.doi.org/10.1080/17452759.2016.1139378
[29]Ehler E, Jayasinghe S N, 2014, Cell electrospinning cardiac patches for tissue engineering the heart. Analyst, 139(18): 4449–4452. http://dx.doi.org/10.1039/c4an00766b
[30]Jayasinghe S N, Qureshi, A N, Eagles, P A, et al., 2006, Electrohydrodynamic jet processing: An advanced electric-field-driven jetting phenomenon for processing living cells. Small, 2(2): 216–219. http://dx.doi.org/10.1002/smll.200500291
[31]Gasperini L, Manigiglio D, Motta A, et al., 2015, An electrohydrodynamic bioprinter for alginate hydrogels containing living cells. Tissue Eng Part C Methods, 21(2): 123–132. http://dx.doi.org/10.1089./ten.tec.2014.0149
[32]Yeo M, Ha J H, Lee H, et al., 2016, Fabrication of hASCs-laden structures using extrusion-based bioprinting supplemented with an electric field. Acta Biomater, 38: 33–43. http://dx.doi.org/10.1016/j.actbio.2016.04.017
[33]He J K, Zhao X, Chang J K, et al., 2017, Microscale electrohydrodynamic bioprinting with high viability. Small: 1702626. http://dx.doi.org/10.1002/smll.201702626