Mechanism for corrosion protection of β-TCP reinforced ZK60 via laser rapid solidification
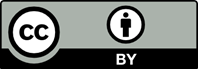
It remains the primary issue to enhance the corrosion resistance of Mg alloys for their clinical applications. In this study, β-tricalcium phosphate (β-TCP) was composited with Mg-6Zn-1Zr (ZK60) using laser rapid solidification to improve the degradation behavior. Results revealed rapid solidification effectively restrained the aggregation of β-TCP, which thus homogenously distributed along grain boundaries of α-Mg. Significantly, the uniformly distributed β-TCP in the matrix promoted the formation of apatite layer on the surface, which contributed to the formation of a compact corrosion product layer, hence retarding the further degradation. Furthermore, ZK60/8β-TCP (wt. %) composite showed improved mechanical strength, as well as improved cytocompatibility. It was suggested that laser rapidly solidified ZK60/8β-TCP composite might be a potential materials for tissue engineering.
[1]Chen Y, Xu Z, Smith C, et al., 2014, Recent advances on the development of magnesium alloys for biodegradable implants. Acta Biomater, 10(11): 4561–4573. http://dx.doi.org/ 10.1016/j.actbio.2014.07.005
[2]Tie D, Guan R, Liu H, et al., 2016, An in vivo study on the metabolism and osteogenic activity of bioabsorbable Mg–1Sr alloy. Acta Biomater, 29: 455–467. http://dx.doi.org/10.1016/j.actbio.2015.11.014
[3]Yazdani M, Yazdani M, Afshar A, et al., 2017, Electrochemical evaluation of AZ 31 magnesium alloy in two simulated biological solutions. Anti-Corros Method M, 64(1): 103–108. http://dx.doi.org/10.1108/ACMM-02-2016-1649
[4]Ge S, Wang Y, Tian J, et al., 2016, An in vitro study on the biocompatibility of WE magnesium alloys. J Biomed Mater Res B Appl Biomater, 104(3): 482–487. http://dx.doi.org/10.1002/jbm.b.33388
[5]Feng A and Han Y, 2010, The microstructure, mechanical and corrosion properties of calcium polyphosphate reinforced ZK60A magnesium alloy composites. J Alloys Compd, 504(2): 585–593. http://dx.doi.org/10.1016/j.jallcom.2010.06.013
[6]Shuai C, Yang Y, Wu P, et al., 2017, Laser rapid solidification improves corrosion behavior of Mg-Zn-Zr alloy. J Alloys Compd, 691: 961–969. https://doi.org/10.1016/j.jallcom.2016.09.019
[7]Li N and Zheng Y, 2013, Novel magnesium alloys developed for biomedical application: A review. J Mater Sci Technol, 29(6): 489–502. https://doi.org/10.1016/j.jmst.2013.02.005
[8]Del Campo R, Savoini B, Munoz A, et al., 2014, Mechanical properties and corrosion behavior of Mg–HAP composites. J Mech Behav Biomed Mater, 39: 238–246. https://doi.org/10.1016/j.jmbbm.2014.07.014
[9]Wan Y, Cui T, Li W, et al., 2016, Mechanical and biological properties of bioglass/magnesium composites prepared via microwave sintering route. Mater Des, 99: 521–527. https://doi.org/10.1016/j.matdes.2016.03.096
[10]Feng A and Han Y, 2011, Mechanical and in vitro degradation behavior of ultrafine calcium polyphosphate reinforced magnesium-alloy composites. Mater Des, 32(5): 2813–2820. https://doi.org/10.1016/j.matdes.2010.12.054
[11]He S-Y, Yue S, Chen M-F, et al., 2011, Microstructure and properties of biodegradable β-TCP reinforced Mg-Zn-Zr composites. Trans Nonferrous Met Soc China, 21(4): 814–819. https://doi.org/10.1016/S1003-6326(11)60786-3
[12]Liu D, Zuo Y, Meng W, et al., 2012, Fabrication of biodegradable nano-sized β-TCP/Mg composite by a novel melt shearing technology. Mater Sci Eng C, 32(5): 1253–1258. https://doi.org/10.1016/j.msec.2012.03.017
[13]Yazdimamaghani M, Razavi M, Vashaee D, et al., 2016, In vitro analysis of Mg scaffolds coated with polymer/hydrogel/ceramic composite layers. Surf Coat Technol, 301: 126–132. https://doi.org/10.1016/j.surfcoat.2016.01.017
[14]Xie D, Zhao J, Qi Y, et al., 2013, Decreasing pores in a laser cladding layer with pulsed current. Chin Opt Lett, 11(11): 111401. https://doi.org/10.3788/COL201311.111401.
[15]Liang Y-J, Li J, Li A, et al., 2017, Solidification path of single-crystal nickel-base superalloys with minor carbon additions under laser rapid directional solidification conditions. Scr Mater, 127: 58–62. https://doi.org/10.1016/j.scriptamat.2016.08.039
[16]Banerjee R, Collins P Cand Fraser H L, 2002, Laser deposition of in situ Ti–TiB composites. Adv Eng Mater, 4(11): 847-851. https://doi.org/10.1002/1527-2648(20021105)4:11<847::AID-ADEM847>3.0.CO;2-C
[17]Yang Y, Wu P, Lin X, et al., 2016, System development, formability quality and microstructure evolution of selective laser-melted magnesium. Virtual Phys Prototyp, 11(3): 1–9. http://dx.doi.org/10.1080/17452759.2016.1210522
[18]Pillai R S, Frasnelli Mand Sglavo V M, 2017, HA/β-TCP Plasma Sprayed Coatings on Ti Substrate for Biomedical Applications. Ceram Int. https://doi.org/10.1016/j.ceramint.2017.08.113
[19]Sutton A T, Kriewall C S, Ming C L, et al., 2016, Powder characterisation techniques and effects of powder characteristics on part properties in powder-bed fusion processes. Virtual Phys Prototyp, 12(1): 3–29. http://dx.doi.org/10.1080/17452759.2016.1250605
[20]Ivanchenko P, Delgado-López J M, Iafisco M, et al., 2017, On the surface effects of citrates on nano-apatites: Evidence of a decreased hydrophilicity. Sci Rep, 7. http://dx.doi.org/10.1038/s41598-017-09376-x
[21]Sing S L, Yeong W Y, Wiria F E, et al., 2017, Direct selective laser sintering and melting of ceramics: a review. Rapid Prototyp J, 23(3): 611–623. http://dx.doi.org/10.1108/RPJ-11-2015-0178
[22]Huang Y, Liu D, Anguilano L, et al., 2015, Fabrication and characterization of a biodegradable Mg–2Zn–0.5 Ca/1β-TCP composite. Mater Sci Eng C, 54: 120–132. http://dx.doi.org/10.1016/j.msec.2015.05.035
[23]Yan Y, Kang Y, Li D, et al., 2017, Improvement of the mechanical properties and corrosion resistance of biodegradable β-Ca 3 (PO 4) 2/Mg-Zn composites prepared by powder metallurgy: The adding β-Ca 3 (PO 4) 2, hot extrusion and aging treatment. Mater Sci Eng C, 74: 582–596. http://dx.doi.org/10.1016/j.msec.2016.12.132
[24]Yashima M, Sakai A, Kamiyama T, et al., 2003, Crystal structure analysis of β-tricalcium phosphate Ca3(PO4)2 by neutron powder diffraction. J Solid State Chem, 175(2): 272–277. http://dx.doi.org/10.1016/S0022-4596(03)00279-2
[25]Garoushi S K, Hatem M, Lassila L V, et al., 2015, The effect of short fiber composite base on microleakage and load-bearing capacity of posterior restorations. Acta Biomater Odontol Scand, 1(1): 6–12. http://dx.doi.org/10.3109/23337931.2015.1017576
[26]Agarwal S, Curtin J, Duffy B, et al., 2016, Biodegradable magnesium alloys for orthopaedic applications: A review on corrosion, biocompatibility and surface modifications. Mater Sci Eng C, 68: 948–963. http://dx.doi.org/10.1016/j.msec.2016.06.020
[27]Geng F, Tan L, Jin X, et al., 2009, The preparation, cytocompatibility, and in vitro biodegradation study of pure β-TCP on magnesium. J Mater Sci Mater Med, 20(5): 1149–1157. http://dx.doi.org/10.1007/s10856-008-3669-x
[28]Kokubo T, 1996, Formation of biologically active bone-like apatite on metals and polymers by a biomimetic process. Thermochim Acta, 280–281: 479–490. http://dx.doi.org/10.1016/0040-6031(95)02784-X
[29]Zhang L, Pei J, Wang H, et al., 2017, Facile preparation of poly (lactic acid)/brushite bilayer coating on biodegradable magnesium alloys with multiple functionalities for orthopedic application. ACS Appl Mater Interfaces, 9(11): 9437–9448. http://dx.doi.org/10.1021/acsami.7b00209
[30]Ilich J Z and Kerstetter J E, 2000, Nutrition in bone health revisited: A story beyond calcium. J Am Coll Nutr, 19(6): 715–737. http://dx.doi.org/10.1080/07315724.2000.10718070
[31]Li Z, Gu X, Lou S, et al., 2008, The development of binary Mg–Ca alloys for use as biodegradable materials within bone. Biomaterials, 29(10): 1329–1344. http://dx.doi.org/10.1016/j.biomaterials.2007.12.021