Post-printing surface modification and functionalization of 3D-printed biomedical device
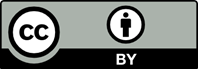
3D printing is a technology well-suited for biomedical applications due to its ability to create highly complex and arbitrary structures from personalized designs with a fast turnaround. However, due to a limited selection of 3D-printable materials, the biofunctionality of many 3D-printed components has not been paid enough attention. In this perspective, we point out that post-3D printing modification is the solution that could close the gap between 3D printing technology and desired biomedical functions. We identify architectural reconfiguration and surface functionalization as the two main post-3D printing modification processes and discuss potential techniques for post-3D printing modification to achieve desired biofunctionality.
1. Leong K F, Cheah C M, and Chua C K, 2003, Solid freeform fabrication of three-dimensional scaffolds for engineering replacement tissues and organs. Biomaterials, vol.24(13): 2363–2378.
https://doi.org/10.1016/S0142-9612(03)00030-9.
2. Utela B, Storti D, Anderson R, et al., 2008, A review of process development steps for new material systems in three dimensional printing (3DP). Journal of Manufacturing Processes, vol.10(2): 96–104.
https://doi.org/10.1016/j.jmapro.2009.03.002.
3. Yang S, Leong K-F, Du Z, et al., 2002, The design of scaffolds for use in tissue engineering. Part II. Rapid prototyping techniques. Tissue Engineering, vol.8(1):
1–11.
https://doi.org/10.1089/107632702753503009.
4. Yeong W-Y, Chua C-K, Leong K-F, et al., 2004, Rapid prototyping in tissue engineering: challenges and potential. Trends in Biotechnology, vol.22(12): 643–652.
https://doi.org/10.1016/j.tibtech.2004.10.004.
5. Gross B C, Erkal J L, Lockwood S Y, et al., 2014, Evaluation of 3D printing and its potential impact on biotech-nology and the chemical sciences. Analytical Chemistry, vol.86(7): 3240–3253.
https://doi.org/10.1021/ac403397r.
6. D'Aveni R, 2015, The 3-D printing revolution. Harvard Business Review, vol.2015(May): 40–48.
7. Rengier F, Mehndiratta A, von Tengg-Kobligk H, et al., 2010, 3D printing based on imaging data: Review of medical applications. International Journal of Computer Assisted Radiology and Surgery, vol.5(4): 335–341.
https://doi.org/10.1007/s11548-010-0476-x.
8. Singare S, Lian Q, Ping Wang W, et al., 2009, Rapid prototyping assisted surgery planning and custom implant design. Rapid Prototyping Journal, vol.15(1): 19–23.
https://dx.doi.org/10.1108/13552540910925027
9. Zein N N, Hanouneh I A, Bishop P D, et al., 2013, Three- dimensional print of a liver for preoperative planning in living donor liver transplantation. Liver Transplantation, vol.19(12): 1304–1310.
https://doi.org/10.1002/lt.23729.
10. Scalfani V F and Vaid T P, 2014, 3D printed molecules and extended solid models for teaching symmetry and point groups. Journal Chemical Education, vol.91(8): 1174–1180.
https://doi.org/10.1021/ed400887t.
11. Tibbits S and Falvello A, 2013, Biomolecular, chiral and irregular self-assemblies, in Proceedings of the Association for Computer Aided Design in Architecture 2013: Adaptive Architecture. 2013: Waterloo, Canada.
12. Cheung H-Y, Lau K-T, Lu T-P, et al., 2007, A critical re-view on polymer-based bio-engineered materials for scaffold development. Composites Part B: Engineering, vol.38(3): 291–300.
https://doi.org/10.1016/j.compositesb.2006.06.014.
13. Ho C M B, Ng S H, and Yoon Y-J, 2015, A review on 3D printed bioimplants. International Journal of Precision Engineering and Manufacturing, vol.16(5): 1035–1046.
https://doi.org/10.1007/s12541-015-0134-x.
14. Peltola S M, Melchels F P W, Grijpma D W, et al., 2008, A review of rapid prototyping techniques for tissue engineering purposes. Annals of Medicine, vol.40(4): 268–280.
https://doi.org/10.1080/07853890701881788.
15. Wong K C, Kumta S M, Geel N V, et al., 2015, One-step reconstruction with a 3D-printed, biomechanically evaluated custom implant after complex pelvic tumor resection. Computer Aided Surgery, vol.20(1): 14–23.
https://doi.org/10.3109/10929088.2015.1076039.
16. Bhattacharjee N, Urrios A, Kang S, et al., 2016, The up-coming 3D-printing revolution in microfluidics. Lab on a Chip, vol.16(10): 1720–1742.
https://doi.org/10.1039/C6LC00163G.
17. Au A K, Huynh W, Horowitz L F, et al., 2016, 3D-Printed microfluidics. Angewandte Chemie International Edition.
18. Lee J M, Zhang M, and Yeong W Y, 2016, Characteriza-tion and evaluation of 3D printed microfluidic chip for cell processing. Microfluidics and Nanofluidics, vol.20(1): 1–15.
https://doi.org/10.1007/s10404-015-1688-8.
19. Au A K, Bhattacharjee N, Horowitz L F, et al., 2015, 3D-printed microfluidic automation. Lab on a Chip, vol. 15(8): 1934–1941.
https://doi.org/10.1039/c5lc00126a.
20. Waheed S, Cabot J M, Macdonald N P, et al., 2016, 3D printed microfluidic devices: enablers and barriers. Lab on a Chip, vol.16(11): 1993–2013.
https://doi.org/10.1039/C6LC00284F.
21. Ho C M B, Ng S H, Li K H H, et al., 2015, 3D printed microfluidics for biological applications. Lab on a Chip, vol.15(18): 3627–3637.
https://doi.org/10.1039/C5LC00685F.
22. MacDonald E and Wicker R, 2016, Multiprocess 3D printing for increasing component functionality. Science, vol.353(6307): aaf2093.
https://doi.org/10.1126/science.aaf2093.
23. Ge Q, Sakhaei A H, Lee H, et al., 2016, Multimaterial 4D printing with tailorable shape memory polymers. Scientific Reports,
vol.6. https://doi.org/10.1038/srep31110.
24. Khoo Z X, Teoh J E M, Liu Y, et al., 2015, 3D printing of smart materials: a review on recent progresses in 4D printing. Virtual and Physical Prototyping, vol.10(3): 103–122.
https://dx.doi.org/10.1080/17452759.2015.1097054.
25. Gladman A S, Matsumoto E A, Nuzzo R G, et al., 2016, Biomimetic 4D printing. Nature Materials, vol.15.
https://doi.org/10.1038/nmat4544.
26. An J, Chua C K, and Mironov V, 2016, A perspective on 4D bioprinting. International Journal of Bioprinting, vol 2(1): 3–5.
http://dx.doi.org/10.18063/IJB.2016.01.003.
27. Tibbits S, 2014, 4D printing: multi-material shape change. Architectural Design, vol.84(1): 116-121.
https://doi.org/10.1002/ad.1710.
28. Ilievski F, Mazzeo A D, Shepherd R F, et al., 2011, Soft robotics for chemists. Angewandte Chemie, vol.50(8): 1890– 1895.
https://doi.org/10.1002/anie.201006464.
29. Tan D, Li Y, Qi F, et al., 2007, Reduction in feature size of two-photon polymerization using SCR500. Applied Physics Letters, vol.90(7): 071106.
https://dx.doi.org/10.1063/1.2535504.
30. Grimes A, Breslauer D N, Long M, et al., 2008, Shrinky-Dink microfluidics: rapid generation of deep and rounded patterns. Lab on a Chip, vol.8(1): 170–172.
https://doi.org/10.1039/b711622e.
31. Chia H N and Wu B M, 2015, Recent advances in 3D printing of biomaterials. Journal of Biological Engineering, vol.9(1): 4.
https://doi.org/10.1186/s13036-015-0001-4.
32. Kang S M, Hwang N S, Yeom J, et al., 2012, One-step multipurpose surface functionalization by adhesive cate-cholamine. Advanced Functional Materials, vol.22(14): 2949–2955.
https://doi.org/10.1002/adfm.201200177.
33. Lee S J, Lee D, Yoon T R, et al., 2016, Surface modification of 3D-printed porous scaffolds via mussel-inspired polydopamine and effective immobilization of rhBMP-2 to promote osteogenic differentiation for bone tissue engineering. Acta Biomaterialia, vol.40: 182–191.
https://doi.org/10.1016/j.actbio.2016.02.006.
34. Yeh C-H, Chen Y-W, Shie M-Y, et al., 2015, Poly (dopamine)-assisted immobilization of Xu Duan on 3D printed poly (lactic acid) scaffolds to up-regulate osteo-genic and angiogenic markers of bone marrow stem cells. Materials, vol.8(7): 4299–4315.
https://doi.org/10.3390/ma8074299.
35. Liu Y, Ai K, and Lu L, 2014, Polydopamine and its derivative materials: synthesis and promising applications in energy, environmental, and biomedical fields. Chemical Reviews, vol.114(9): 5057–5115.
https://doi.org/10.1021/cr400407a.
36. Kao C-T, Lin C-C, Chen Y-W, et al., 2015, Poly (dopamine) coating of 3D printed poly (lactic acid) scaffolds for bone tissue engineering. Materials Science and Engineering: C, vol.56: 165–173.
https://doi.org/10.1016/j.msec.2015.06.028.
37. Lee H, Dellatore S M, Miller W M, et al., 2007, Mussel-inspired surface chemistry for multifunctional coatings. Science, vol.318(5849): 426–430.
https://doi.org/10.1126/science.1147241.
38. Pensa E, Cortés E, Corthey G n, et al., 2012, The chemistry of the sulfur–gold interface: in search of a unified model. Accounts of Chemical Research, vol.45(8): 1183– 1192.
https://doi.org/10.1021/ar200260p.
39. Wang X, Cai X, Guo Q, et al., 2013, i3DP, a robust 3D printing approach enabling genetic post-printing surface modification. Chemical Communications, vol.49(86): 10064–10066.
https://doi.org/10.1039/c3cc45817b.
40. Valeur E and Bradley M, 2009, Amide bond formation: beyond the myth of coupling reagents. Chemical Society Reviews, vol.38(2): 606–631.
https://doi.org/10.1039/B701677H.
41. Kolb H C, Finn M G, and Sharpless K B, 2001, Click chemistry: diverse chemical function from a few good reactions. Angewandte Chemie International Edition, vol.40(11): 2004–2021.
https://doi.org/10.1002/1521-3773(20010601)40:11<2004::AID-ANIE2004>3.0.CO;2-5.
42. Banerjee I, Pangule R C, and Kane R S, 2011, Antifouling coatings: Recent developments in the design of surfaces that prevent fouling by proteins, bacteria, and marine organisms. Advanced Materials, vol.23(6): 690–718.
https://doi.org/10.1002/adma.201001215.
43. Sánchez-Salcedo S, Colilla M, Izquierdo-Barba I, et al., 2016, Preventing bacterial adhesion on scaffolds for bone tissue engineering. International Journal of Bioprinting, vol.2(1): 20–34.
http://dx.doi.org/10.18063/IJB.2016.01.008.
44. Liu L, Chen G, Chao T, et al., 2008, Reduced foreign body reaction to implanted biomaterials by surface treatment with oriented osteopontin. Journal of Biomaterials Science, Polymer Edition, vol.19(6): 821–835.
https://dx.doi.org/10.1163/156856208784522083.
45. Oskui S M, Diamante G, Liao C, et al., 2015, Assessing and reducing the toxicity of 3D-printed parts. Environmental Science & Technology Letters, vol.3(1): 1–6.
https://doi.org/10.1021/acs.estlett.5b00249.